Since its introduction in the mid-2010s, optical coherence tomography angiography (OCTA) has rapidly become a popular tool used by clinicians and researchers for noninvasive diagnosis and management of retinal disorders. Unlike traditional optical coherence tomography (OCT), which provides detailed structured imaging of the retina and choroid, OCTA offers the added capability of visualizing blood flow within retinal and choroidal vasculature.
In more recent years, the development of swept-source (SS) technology and SS-OCTA has allowed great depth of penetration and resolution. Additional improvements in swept-source scanning speeds have allowed for even wider fields of view. Expanded field (EF), widefield (WF), and ultrawidefield (UWF) SS-OCTA capture much larger fields of view, covering between 100° and 220° depending on the specific technology. These extended fields of view allow for the detection of peripheral retinal vascular abnormalities, offering a more comprehensive assessment of retinal pathologies (Figure 1). Here, we aim to discuss widefield SS-OCTA technology, including its clinical applications, limitations, and future directions.
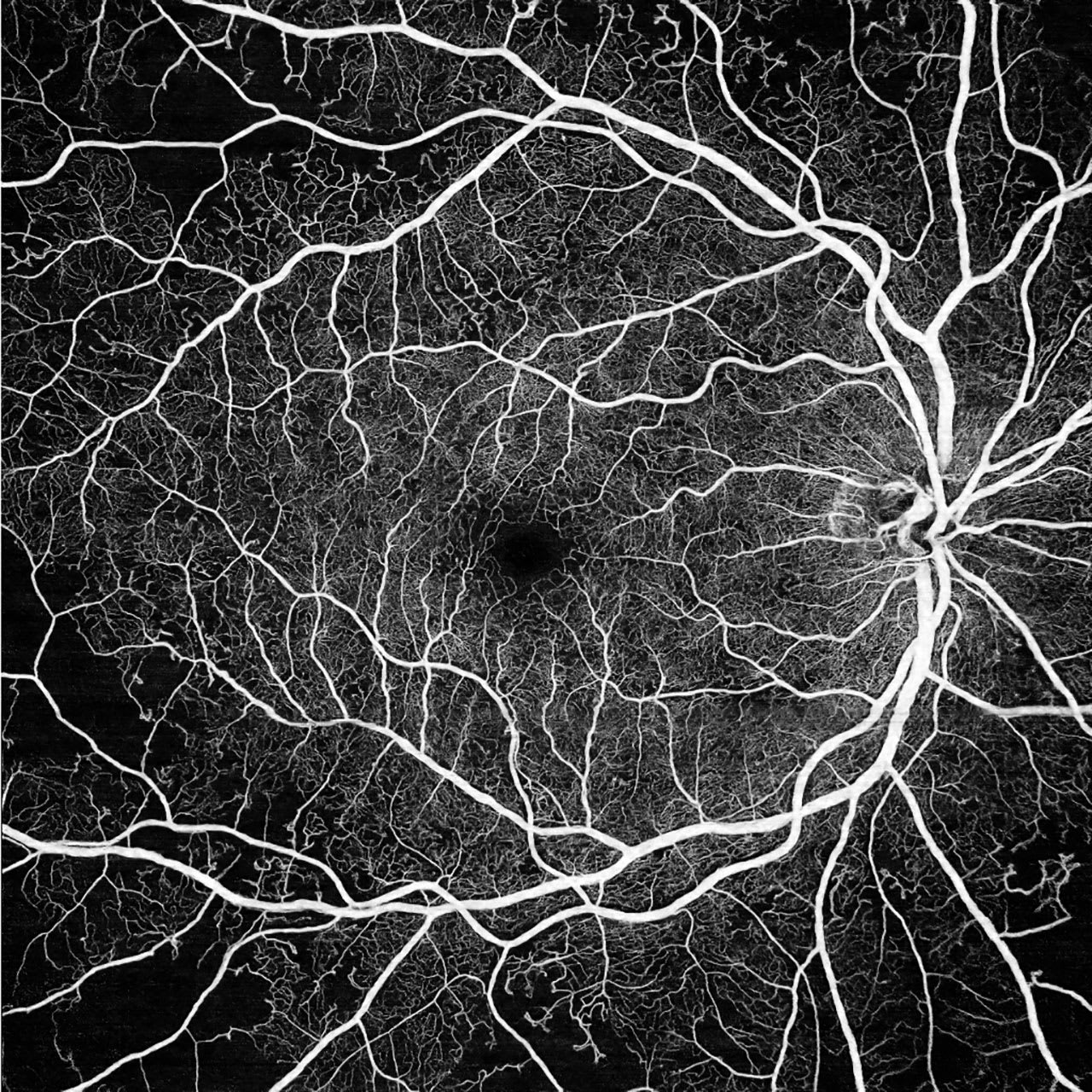
Figure 1. 12 mm x 12 mm widefield scan of a patient with nonproliferative diabetic retinopathy (DR). Nonperfusion areas (NPAs) are seen predominantly in the periphery of the scan, highlighting the advantages provided by widefield and ultrawidefield swept-source optical coherence tomography angiography (SS-OCTA). Image courtesy John B. Miller, MD.
Comparative Analysis with Traditional Imaging Modalities
Widefield OCTA (WF OCTA) has certain advantages and drawbacks compared to the traditional retinal imaging modalities of fluorescein angiography (FA) and fundus photography. Fundus photography has long been the gold standard for diabetic retinopathy (DR) screening and evaluation. It is more readily available and easier to use than other imaging modalities for DR, but it can only visualize in 2 dimensions and does not provide retinal blood flow information. FA has grown increasingly more common in the evaluation of advanced stages of DR, particularly with the addition of UWF FA technology in most retina clinics now. However, FA is more time-consuming and invasive, posing systemic risks to the patient. Diagnostically, FA cannot provide depth-resolved imaging to help in the differentiation of DR lesions.
In contrast, WF OCTA can provide 3-dimensional, depth-resolved images of retinal and choroidal vasculature while maintaining a low risk profile.1,2 Widefield OCTA does not require dye, making it safer for patients with known allergies or systemic illnesses, and can provide more precise and quantitative information about peripheral nonperfusion and neovascularization, which appear more “muddy” in traditional imaging.
Clinical Applications in Retinal Diseases
Widefield OCTA has proven to be a valuable diagnostic tool for various retinal diseases. In DR, WF OCTA accurately detects vascular abnormalities, particularly peripheral nonperfused areas (NPAs), which are key indicators of disease severity and progression.3 Studies also indicate that WF OCTA matches or outperforms traditional imaging methods in identifying DR-related retinal lesions, such as intraretinal microvascular abnormalities.1,4
Widefield OCTA is also valuable in diagnosing and managing retinal vein occlusions (RVOs), inflammatory retinal conditions, and age-related macular degeneration (AMD). For both ischemic and nonischemic RVO, WF OCTA offers detailed, noninvasive visualization and quantification of NPAs, providing critical insights into the extent of ischemia (Figure 2). This information aids clinicians in determining prognosis and planning treatments, such as laser therapy or anti-VEGF injections.5 In retinal vasculitis, WF OCTA can detect peripheral vascular abnormalities and ischemia that might otherwise go unnoticed with other imaging techniques.6 Additionally, while AMD primarily affects the central retina, WF OCTA can identify peripheral neovascularization extending beyond the macula, offering valuable insights for early diagnosis and advanced disease management.
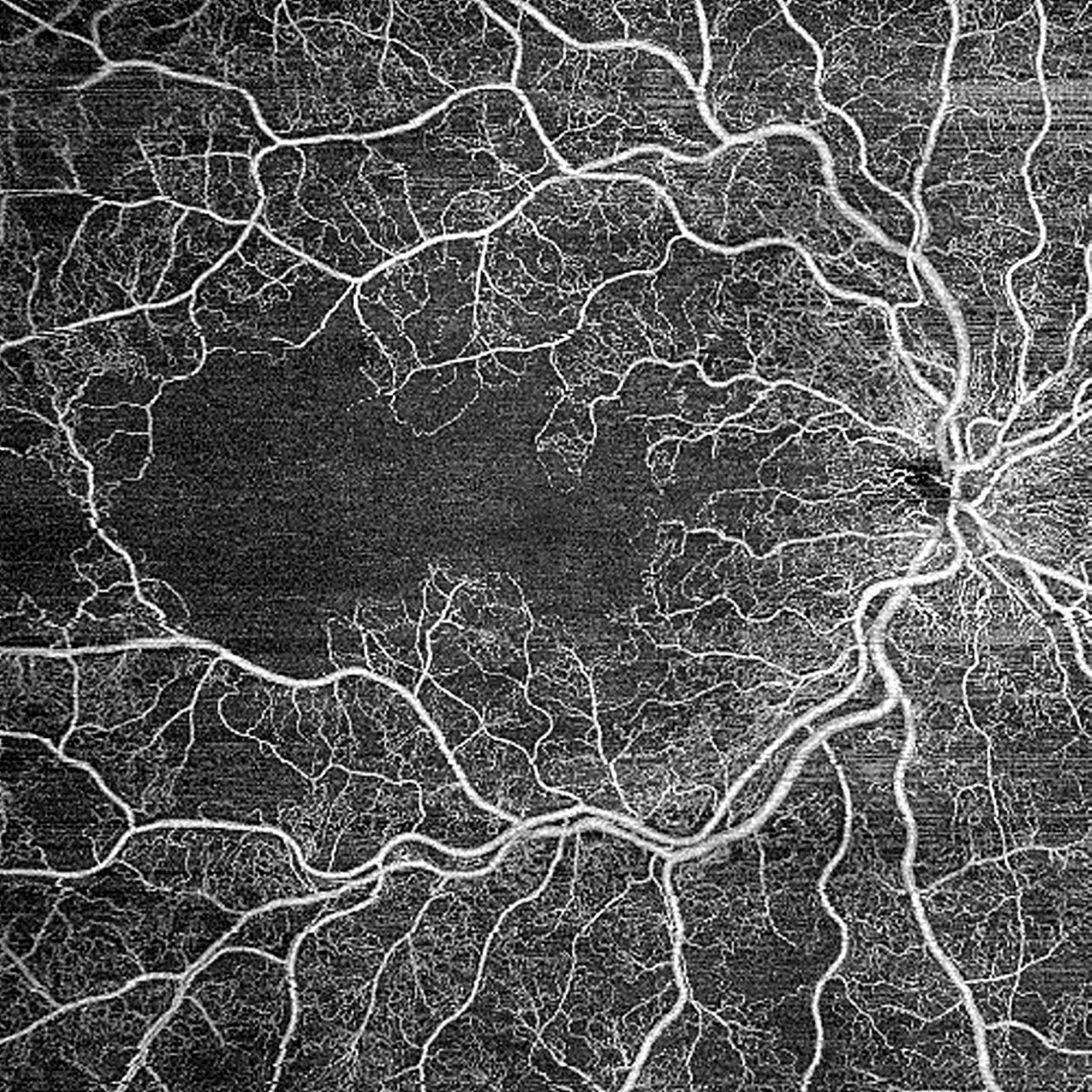
Figure 2. 12 mm x 12 mm widefield scan of a patient diagnosed with ischemic retinal vein occlusion (RVO). A large area of ischemia is seen, demonstrating the detailed imaging capabilities of widefield swept-source optical coherence tomography angiography (SS-OCTA). Image courtesy John B. Miller, MD.
Challenges and Limitations
Despite the utility of widefield SS-OCTA, there remain several challenges and limitations. One major limitation of these imaging techniques is image quality, resolution, and signal strength in the peripheral retina. As the field of view increases, there is a well-documented tradeoff in image resolution, particularly with ultrawidefield SS-OCTA.7 This can limit the detection of subtle changes in the peripheral retina, an area that ultrawidefield can visualize but traditional SS-OCTA cannot. For similar reasons, notably increased imaging distance and angular distortion, peripheral signal strength is often weaker in the periphery. In some instances, areas of reduced signal strength may even mimic NPAs, further complicating analysis.8
Furthermore, WF OCTA is more susceptible to motion artifact than traditional SS-OCTA. Involuntary eye movements and blinking may consequently lead to the distortion or misinterpretation of vascular structures. This phenomenon is especially evident in the visualization of deeper retinal layers such as the choriocapillaris, which are more affected by motion artifact than superficial layers.
Lastly, cost and interdevice variability pose unique challenges. Variability between WF and UWF SS-OCTA devices manufactured by different companies limits the quantitative utility of WF SS-OCTA. Although there has been an emergence of leading widefield OCTA devices, such as the Zeiss CLARUS 700 and Optos Silverstone, variability in image protocols, resolution, and software algorithms between devices makes quantitative comparisons difficult. In a similar vein, the cost of WF SS-OCTA devices presents yet another barrier to widespread implementation in clinical practice. These advanced imaging systems are more expensive than traditional devices, with high up-front costs and additional costs for maintenance, software updates, and operator training. Efforts to reduce costs or develop more affordable models will be crucial for expanding the accessibility and utility of widefield and ultrawidefield SS-OCTA.
Technological Advancements in Widefield OCTA
Despite the relatively recent introduction of WF OCTA, notable technological improvements have further increased its utility. First, integrating SS technology into WF OCTA has proven beneficial for increasing the practicality of wider field OCTA in clinical settings. Swept-source technology, which is already used in traditional OCTA, makes use of longer wavelengths to better penetrate the retinal and choroidal layers and to avoid scattering by cataracts and the RPE. Because SS technology is capable of higher A-scan speeds (more than 100,000 scans per second), it can help reduce the longer scan time that WF OCTA requires to cover the extra area. SS technology can also help improve signal strength and image quality at the scan’s edges, which can be more challenging to precisely visualize in WF OCTA.
Motion and blinking detection algorithms are currently being developed to implement real-time rescanning in areas where correction is necessary due to eye movement and blinking during imaging.9 This feature might eventually help improve clinician efficiency and patient experience. Early efforts have also been made to integrate deep learning and artificial intelligence (AI) into WF OCTA, including automatic diagnosis, which will likely continue to develop as AI and diagnostic infrastructure evolve.10,11
Future Directions and Research Opportunities
The future of WF and UWF OCTA is bright and marked by several advancements aimed at overcoming current limitations and expanding clinical utility. One promising direction is the integration of AI and machine learning (ML) algorithms. Lin et al used deep learning to improve blood flow contrast and reduce motion artifacts.12 Artificial intelligence and ML may also be used to address the issue of SS-OCTA device standardization, which would improve reproducibility across devices and streamline clinic workflows.
The integration of WF SS-OCTA with other imaging modalities, such as FA and fundus photography, is an area of further interest. Multimodal imaging platforms could combine the strengths of each modality individually, offering a more comprehensive approach to disease management. This concept was explored by Hao Wei and colleagues, who introduced the first multimodal, multidisease, UWF OCTA data set, which combined structural OCTA images with other modalities to improve diagnostic accuracy for various retinal conditions.13
Widefield SS-OCTA devices can be improved. Advancements in hardware and software are currently focused on addressing signal attenuation segmentation changes in the peripheral retina. Improved algorithms specifically tailored to UWF imaging may provide more accurate visualization of the peripheral retina, where current systems struggle. Furthermore, enhanced light sources, such as longer wavelength SS-OCTA, could lead to improved penetration and, consequently, improved signal consistency in the peripheral retina.
The current utility of WF SS-OCTA remains only partially understood, with its full potential yet to be fully explored. Emerging applications, such as the development of novel metrics like vessel tortuosity and capillary perfusion density in the peripheral retina, hold promise for offering deeper insights into systemic diseases with ocular manifestations. These efforts aim to broaden our understanding of how WF SS-OCTA can be effectively used in clinical and research settings.
Conclusion
Widefield and UWF SS-OCTA represent recent advancements in retinal imaging, offering an expanded visualization of the peripheral retina compared to traditional SS-OCTA. These technologies will continue to advance the diagnosis and management of common retinal diseases, including DR, RVO, and AMD. Despite their potential to revolutionize the field of ophthalmology, challenges such as increased artifact, peripheral signal strength, and high costs remain barriers to widespread implementation. Current evidence best supports the integration of wider field SS-OCTA with other imaging modalities to optimize patient care. As research continues to broaden the utility of WF SS-OCTA, these innovations are poised to become an essential component of routine eye care. RP
References
1. Cui Y, Zhu Y, Wang JC, et al. Comparison of widefield swept-source optical coherence tomography angiography with ultra-widefield colour fundus photography and fluorescein angiography for detection of lesions in diabetic retinopathy. Br J Ophthalmol. 2021;105(4):577-581. doi:10.1136/bjophthalmol-2020-316245
2. Hirano T, Hoshiyama K, Takahashi Y, Murata T. Wide-field swept-source OCT angiography (23 × 20 mm) for detecting retinal neovascularization in eyes with proliferative diabetic retinopathy. Graefes Arch Clin Exp Ophthalmol. 2023;261(2):339-344. doi:10.1007/s00417-022-05878-1
3. Kawai K, Murakami T, Mori Y, et al. Clinically significant nonperfusion areas on widefield OCT angiography in diabetic retinopathy. Ophthalmol Sci. 2022;3(1):100241. doi:10.1016/j.xops.2022.100241
4. Cui Y, Zhu Y, Lu ES, et al. Widefield swept-source OCT angiography metrics associated with the development of diabetic vitreous hemorrhage: a prospective study. Ophthalmology. 2021;128(9):1312-1324. doi:10.1016/j.ophtha.2021.02.020
5. Kadomoto S, Muraoka Y, Uji A, et al. Nonperfusion area quantification in branch retinal vein occlusion: a widefield optical coherence tomography angiography study. Retina. 2021;41(6):1210-1218. doi:10.1097/IAE.0000000000002999
6. Tian M, Tappeiner C, Zinkernagel MS, Huf W, Wolf S, Munk MR. Evaluation of vascular changes in intermediate uveitis and retinal vasculitis using swept-source wide-field optical coherence tomography angiography. Br J Ophthalmol. 2019;103(9):1289-1295. doi:10.1136/bjophthalmol-2018-313078
7. Siying L, Qiaozhu Z, Xinyao H, Linqi Z, Mingwei Z, Jinfeng Q. Comparison of widefield swept-source optical coherence tomography angiography with ultra-widefield fluorescein angiography for the evaluation of lesions in retinal vein occlusion. BMC Ophthalmol. 2022;22(1):422. doi:10.1186/s12886-022-02642-1
8. Lujan BJ, Calhoun CT, Glassman AR, et al. Optical coherence tomography angiography quality across three multicenter clinical studies of diabetic retinopathy. Transl Vis Sci Technol. 2021;10(3):2. doi:10.1167/tvst.10.3.2
9. Wei X, Hormel TT, Guo Y, Hwang TS, Jia Y. High-resolution wide-field OCT angiography with a self-navigation method to correct microsaccades and blinks. Biomed Opt Express. 2020;11(6):3234. https://doi.org/10.1364/boe.390430.
10. Bhambra N, Antaki F, Malt FE, Xu A, Duval R. Dezzzep learning for ultra-widefield imaging: a scoping review. Graefes Arch Clin Exp Ophthalmol. 2022;260(12):3737-3778. doi:10.1007/s00417-022-05741-3
11. Li Y, El Habib Daho M, Conze PH, et al. Hybrid fusion of high-resolution and ultra-widefield OCTA acquisitions for the automatic diagnosis of diabetic retinopathy. Diagnostics. 2023;13(17):2770. doi:10.3390/diagnostics13172770
12. Lin Z, Zhang Q, Lan G, et al. Deep learning for motion artifact-suppressed OCTA image generation from both repeated and adjacent OCT scans. Mathematics. 2024;12(3):446. https://doi.org/10.3390/math12030446
13. Wei H, Shi P, Bai G, Zhang M, Li S, Yuan W. Leveraging multimodal fusion for enhanced diagnosis of multiple retinal diseases in ultrawide OCTA. 2024 IEEE International Symposium on Biomedical Imaging (ISBI), Athens, Greece:1-5. doi:10.1109/ISBI56570.2024.10635142.