Perfusion deficits have consequences in a wide range of organs. It is understood for the kidney, lung, heart, liver, or peripheral anatomy, when insufficient perfusion results in hypoxic imbalance, it negatively affects performance of the organ and exacerbates disease progression.1 Conversely, increasing perfusion has demonstrated the ability to improve the overall health of an end organ, including arresting or reducing disease progression. The eye is no exception. In the central nervous system (CNS), perfusion deficit is closely linked to both atrophic lesions and storage accumulation, with consequences for brain function.2 Although the retina is part of the CNS, little has been considered regarding the impact of a perfusion deficit on the origin of pathology. Only a few authors such as Hayreh,3 Friedman,4 and, more recently, Smith5 and Rosenfeld,6 have discussed the role perfusion deficits may play in the onset and/or development and progression of macular pathology.
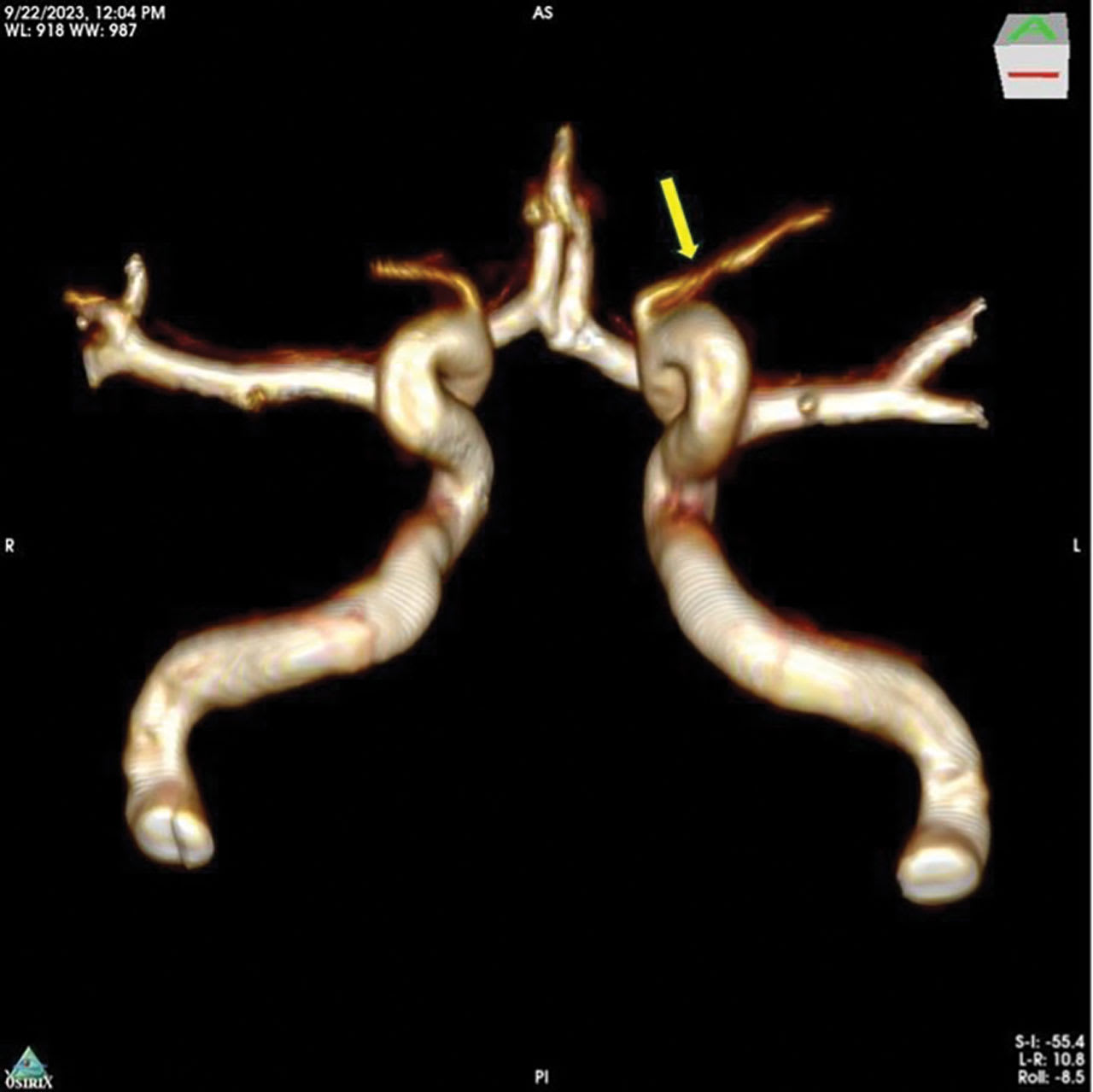
Figure 1. Magnetic resonance imaging angiography shows bilateral internal carotid arteries with ophthalmic artery segments demonstrating defuse stenosis in the long limb of the left ophthalmic artery (yellow arrow). Imaging performed with an Ingenia 3.0T magnetic resonance device (Philips NV). Image courtesy OcuDyne.
Hayreh, who perhaps studied the vascular anatomy of the eye as no one ever did, described the mosaic lobular pattern of the choriocapillaris at the macular level as a very efficient system. However, he also warned that the whole system becomes very inefficient and susceptible to disease processes, as described by age-related macular degeneration (AMD) in the presence of chronic ocular ischemia.7
The relationship between ischemia, impaired nutritional exchange, hemodynamic influence, and AMD,6 as well as its correlation with cardiovascular disease,8 has been known for a number of years. However, the focus necessary to identify the root cause and potential treatment strategy remained elusive. The conversations and scientific debate have had some semblance to the paradox of the chicken and the egg. If retinal damage and perfusion deficit coexist, which comes first? A general consensus is that macular damage of genetic or inflammatory origin results in some feedback mechanism to reduce perfusion to provide for the remaining healthy tissue, but such robust feedback mechanism in the choroid has yet to be identified. What if the opposite could also be true—meaning that a perfusion deficit could induce activation of apoptotic genes, inflammation, and subsequent damage by activating the complement cascade or other pathways? This would lead to functional impairment, atrophy, and cell death, until only the tissue that can be supported with the minimum vital perfusion survives.
Interestingly, an analogous paradigm in cardiovascular pathology is undisputed. That is, a coronary obstruction will deteriorate the function of the tissue, producing ischemia and infarction in the area of insufficient perfusion. Why is this paradigm universally accepted? Probably because cardiology has provided a solid foundation of data. Occlusion can be demonstrated with images and related to functional deficit. Intervention effectively achieves organ reperfusion and rehabilitation. There is no place in cardiology to propose a contrary paradigm in which myocardial cells die by genetic design and thus perfusion is reduced in proportion with tissue loss.
In ophthalmology, adhering strictly to a genetic paradigm with tangential environmental influences has advanced recommendations and treatments that target changes in lifestyle and the cascading responses to ischemia. These include anti-VEGF treatments, complement inhibition,9,10 and attempts to manage the inflammatory response.11 Attempting to control the body’s inherent responses, and thus symptoms of the condition, have demonstrated some success in slowing the damage these responses inflict. However, if the root cause of ischemia is addressed, it could provide the best treatment option and render all medical management more effective in the process. An influx of perfusion, bringing fresh oxygen and improved nutrient exchange, should provide the same benefits to the eye that it does for other parts of the body.
In recent years, a statistical correlation between AMD disease progression and decreased blood flow in the ophthalmic artery (OA) has been reported.12 A retrospective analysis involving a case series of patients evaluated and treated as a compassionate use exercise in Argentina followed. Arterial stenosis was identified in the OA of patients with end-stage AMD. An interventional neuroradiologist then treated the stenosis via balloon angioplasty, resulting in improved visual acuity for these patients.13 Furthermore, as a result of these reports, efforts have increased to effectively capture and document the presence of OA stenosis as a novel target anatomy, using today’s imaging technologies,14 with reasonable success.
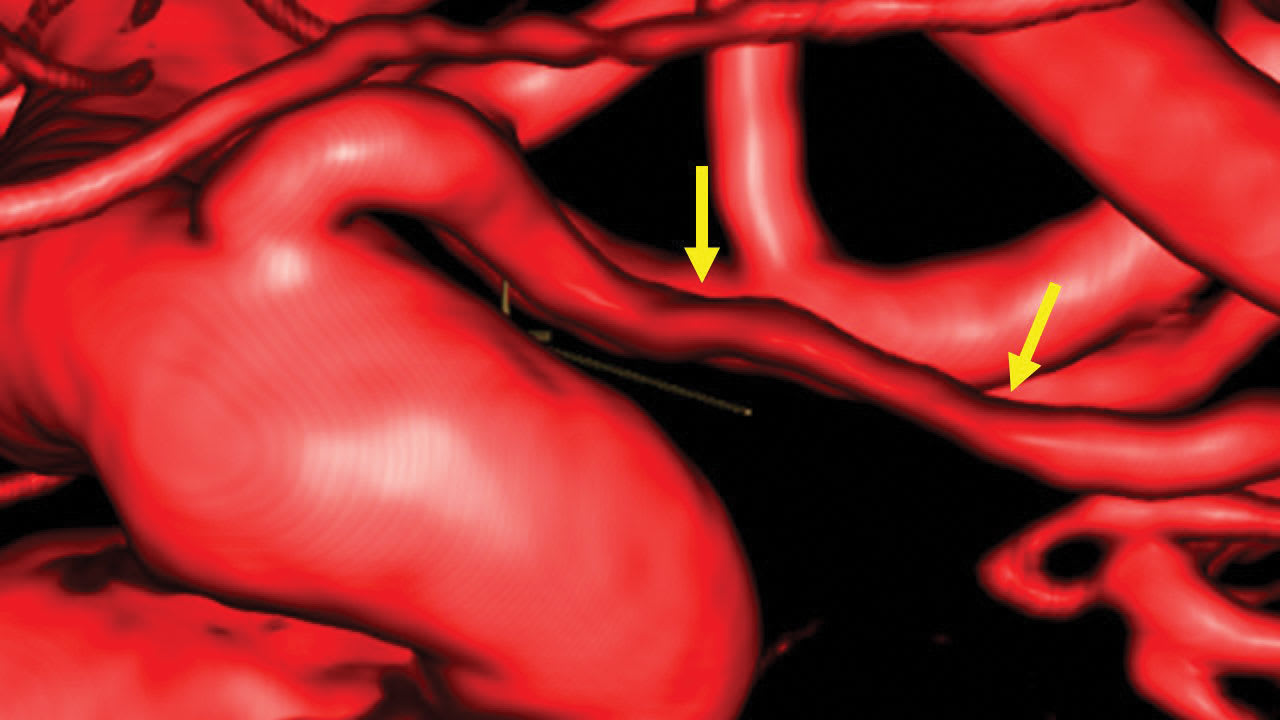
Figure 2. Ophthalmic segment of the left internal carotid artery and ophthalmic artery demonstrating defuse stenosis in the long limb of the ophthalmic artery (between yellow arrows). Three-dimensional rotational angiography image taken with Philips Azurion biplane. Image courtesy OcuDyne.
Testing the Mechanism of Action in a Controlled Clinical Trial
A clinical trial has recently been completed using angioplasty to treat OA stenosis in participants with late-stage dry AMD, to test the ability to replicate the retrospective compassionate use outcomes in a formal safety and feasibility study. A system of investigational devices designed specifically for accessing and treating OA stenosis were tested in the study.15
The study’s inclusion and exclusion criteria were designed to include the presence of OA stenosis and late-stage dry AMD, while decreasing potentially confounding variables and procedural risk to the extent possible (eg, 6-month stability in vascular history and the exclusion of those with more advanced pathology, bleeding risks, or undiagnosed vascular pathology on preoperative magnetic resonance imaging [MRI] angiography and computed tomography [CT] scans).
Subjective ophthalmic testing included Early Treatment Diabetic Retinopathy Study (ETDRS) best-corrected visual acuity (BCVA), as well as native language MN Read testing16,17 and Impact of Vision Impairment (IVI) profile questionnaire.18 Objective ophthalmic testing included subfoveal choroidal thickness (SFChT) via optical coherence tomography (OCT), and color fundus and autofluorescence imaging conducted throughout the study. All ophthalmic imaging was processed through the independent Boston Image Reading Center.
From an interventional neuroradiology perspective, the presence of OA stenosis was identified via screening MRI angiography (Figure 1) and confirmed with preprocedural digital subtraction angiography (Figure 2). Neurologic evaluations were completed before and after the procedure to monitor the subjects. The procedures were completed by an experienced interventional neuroradiologist under general anesthesia, using standard-of-care vascular access procedures, monitoring, and imaging. The study participants were admitted to the treating facility and observed for 18 to 36 hours after the procedure to ensure recovery and stability.
A total of 17 patients were enrolled and treatment was completed in 11 of them. Study eye mean BCVA improved 3.6 ETDRS letters (P=.004), 7.2 letters (P=.001), 7.0 letters (P=.001), and 6.7 letters (P=.003) at the week 1, week 4, month 3, and month 6 visits, respectively. Study eye mean BCVA improvement over mean BCVA of fellow eye were also statistically significant from the week 1 visit, throughout participation. MN Read testing demonstrated improvement in all evaluable eyes through the exit visit. The IVI questionnaire demonstrated mean improvements in both mobility and reading. The OCT imaging demonstrated statistically significant increases over baseline (P=.004) and fellow eyes (P=.03) in mean SFChT through the week 4 visit, remaining above baseline through the month 6 visit. Although the sample size was small for evaluable geographic atrophy (GA) area evaluations, the study treatment did signal potentially significant growth inhibition.
There were no unexpected adverse events reported in the study. Although there were procedure-related ocular adverse events that occurred, all were transient, asymptomatic, and resolved without treatment or sequelae. Similarly, all systemic adverse events were associated with the use of either general anesthesia or vascular access, and were successfully treated and resolved.
A New Treatment Paradigm
The baseline fundamentals that support a perfusion mechanism as a contributing factor to disease progression in dry AMD are consistent with observations of other end organs experiencing decreased perfusion. Furthermore, the presence of OA stenosis has been discovered in this population of patients and this treatment strategy demonstrates a very real potential to disrupt the AMD disease process.
The procedure provides an acceptable safety profile as well as a mean functional improvement in this population of late-stage patients. Due to the nature of the late-stage disease necessary in this study, the sample size was small for evaluable GA area size analysis. The inhibition of GA area growth as compared with untreated fellow eyes must be confirmed in a larger study that includes eyes with smaller and consistently evaluable GA.
From a vascular perspective, although there has been extensive experience accessing the OA for the super-selective administration of chemotherapy in pediatric patients, accessing and navigating the OA for the purposes of OA angioplasty in the treatment of AMD is unique. Though the use of angioplasty to treat a blood flow deficit is a logical and comfortable approach from an interventional neuroradiology standpoint, it is a profound shift in treatment paradigm for the retinal physician, representing a significant departure from today’s standard of care.
To change the paradigm of macular disease, it should be demonstrated that there is a perfusion deficit, explained by stenosis; that an intervention can reverse the state of perfusion deficit; and that a change in perfusion status can reflect favorable changes in function. These elements are consistent with the results of the feasibility study, which will be followed by a larger pivotal study soon.
Changes of this magnitude are not easy and the addition of OA angioplasty to the AMD treatment armamentarium would require a collaborative, multidisciplinary approach to patient treatment and management—no small feat in some places. However, as is the case with all innovative advancements in the medical management of patients, though challenges may cause some discomfort at the onset, they can be consistently and effectively overcome. RP
References
1. Bairey Merz CN, Cheng S, Raggi P, Wei J. End-organ ischemia in the absence of proximal obstructive arterial disease: déjà vu or jamais vu? Atherosclerosis. 2019;287:162-164. doi:10.1016/j.atherosclerosis.2019.05.021
2. Zou X, Liao Y, Jiang C, et al. Brain perfusion, cognition, and plasma Alzheimer’s biomarkers in moyamoya disease. Alzheimers Dement. 2023;19(8):3316-3326. doi:10.1002/alz.12958
3. Hayreh SS. Posterior ciliary artery circulation in health and disease: the Weisenfeld lecture. Invest Ophthalmol Vis Sci. 2004;45(3):749-748. doi:10.1167/iovs.03-0469
4. Friedman E. A hemodynamic model of the pathogenesis of age-related macular degeneration. Am J Ophthalmol. 1997;124(5):677-682. doi:10.1016/s0002-9394(14)70906-7
5. Smith RT, Olsen TW, Chong V, et al. Subretinal drusenoid deposits, age-related macular degeneration, and cardiovascular disease. Asia Pac J Ophthalmol (Phila). 2024;13(1):100036. doi:10.1016/j.apjo.2024.100036
6. Rosenfeld PJ, Trivizki O, Gregori G, Wang RK. An update on the hemodynamic model of age-related macular degeneration. Am J Ophthalmol. 2022;235:291-299. doi:10.1016/j.ajo.2021.08.015
7. Hayreh SS. Submacular choroidal vascular pattern. experimental fluorescein fundus angiographic studies. Albrecht Von Graefes Arch Klin Exp Ophthalmol. 1974;192(3):181-196. doi:10.1007/BF00416865
8. Pennington KL, DeAngelis MM. Epidemiology of age-related macular degeneration (AMD): associations with cardiovascular disease phenotypes and lipid factors. Eye Vis (Lond). 2016;3:34. doi:10.1186/s40662-016-0063-5
9. Heier JS, Lad EM, Holz FG, et al. Pegcetacoplan for the treatment of geographic atrophy secondary to age-related macular degeneration (OAKS and DERBY): two multicentre, randomised, double-masked, sham-controlled, phase 3 trials. Lancet. 2023;402(10411):1434-1448. doi:10.1016/S0140-6736(23)01520-9
10. Khanani AM, Patel SS, Staurenghi G, et al. Efficacy and safety of avacincaptad pegol in patients with geographic atrophy (GATHER2): 12-month results from a randomised, double-masked, phase 3 trial. Lancet. 2023;402(10411):1449-1458. doi:10.1016/S0140-6736(23)01583-0
11. Tan W, Zou J, Yoshida S, Jiang B, Zhou Y. The role of inflammation in age-related macular degeneration. Int J Biol Sci. 2020;16(15):2989-3001. doi:10.7150/ijbs.49890
12. Hibert ML, Chen YI, Ohringer N, et al. Altered blood flow in the ophthalmic and internal carotid arteries in patients with age-related macular degeneration measured using noncontrast MR angiography at 7T. AJNR Am J Neuroradiol. 2021;42(9):1653-1660. doi:10.3174/ajnr.A7187
13. Lylyk I, Bleise C, Lylyk PN, et al. Ophthalmic artery angioplasty for age-related macular degeneration. J Neurointerv Surg. 2022;14(10):968-972. doi:10.1136/neurintsurg-2021-018222
14. Diprose WK, Wang MTM, Reidy J, Ma A, Brodie J, Steinfort B. Ophthalmic artery stenosis on three-dimensional rotational angiography: Interrater agreement, prevalence, and risk factors. Interv Neuroradiol. Published online February 22, 2024. doi:10.1177/15910199241233020
15. A clinical study to evaluate the safety and feasibility of the OcuDyne System in the treatmnet of age-related macular degeneration (AMD). ClinicalTrials.gov identifier: NCT05091476. Updated February 11, 2025. Accessed February 17, 2025.
16. Altinbay D, Adibelli FM, Taskin I, Tekin A. The evaluation of reading performance with Minnesota low vision reading charts in patients with age-related macular degeneration. Middle East Afr J Ophthalmol. 2016;23(4):302-306. doi:10.4103/0974-9233.194078
17. Legge GE, Ross JA, Luebker A, LaMay JM. Psychophysics of reading. VIII. the Minnesota low-vision reading test. Optom Vis Sci. 1989;66(12):843-853. doi:10.1097/00006324-198912000-00008
18. Lamoureux EL, Pallant JF, Pesudovs K, Rees G, Hassell JB, Keeffe JE. The impact of vision impairment questionnaire: an assessment of its domain structure using confirmatory factor analysis and rasch analysis. Invest Ophthalmol Vis Sci. 2007;48(3):1001-1006. doi:10.1167/iovs.06-0361