Vitreoretinal surgery has been part of significant advancements in the ever-evolving landscape of medical technology. Integration of digital technologies has started to change the traditional operating room (OR) setup. Various technologies have emerged to offer vitreoretinal surgeons enhanced visualization, precision, and efficiency. This article explores some highlights of recent digital technologies in the retina OR.
Three-dimensional Heads-up Display Technology
One recent advancement is that of 3-dimensional (3D) heads-up display (HUD) technology. Traditionally, vitreoretinal surgeons have relied on optical microscopes for magnification during surgery. 3D HUD systems, such as the Artevo 800 (Carl Zeiss Meditec) and the Ngenuity (Alcon), consist of a high-resolution display unit and a camera system inserted onto the microscope. The camera projects overlapping images onto a large screen, with stereopsis provided by the use of polarized glasses.1 The 3D HUD has been used for a myriad of surgical indications,2-8 with no significant cases of complications attributable to the HUD.1-4,6 The HUD has been shown to be comparable to standard operating microscopes in terms of surgical success rates.1,2,9,10
There are several advantages to the 3D HUD. One advantage particularly applicable to the retina OR is the ability to provide a 3D stereoscopic view, with improved depth of field. Retinal surgeons in various studies have positively reviewed the enhanced depth of field as an aspect of the system that was noteworthy, particularly in macular cases and in diabetic tractional detachments.2,11 Additionally, the 3D HUD offers enhanced visualization of vitreoretinal structures during surgery. Surgeons can manipulate image contrast and color in real time, optimizing the visibility of various structures. The real-time digital processing includes brightness control among other filters, and can allow surgeons to operate comfortably with lower endoillumination levels.6,7,12 This may reduce the risk of retinal phototoxicity.12 Additionally, the color filters may reduce the amount of dye needed or the need for dye altogether, which can also help limit retinal toxicity.13
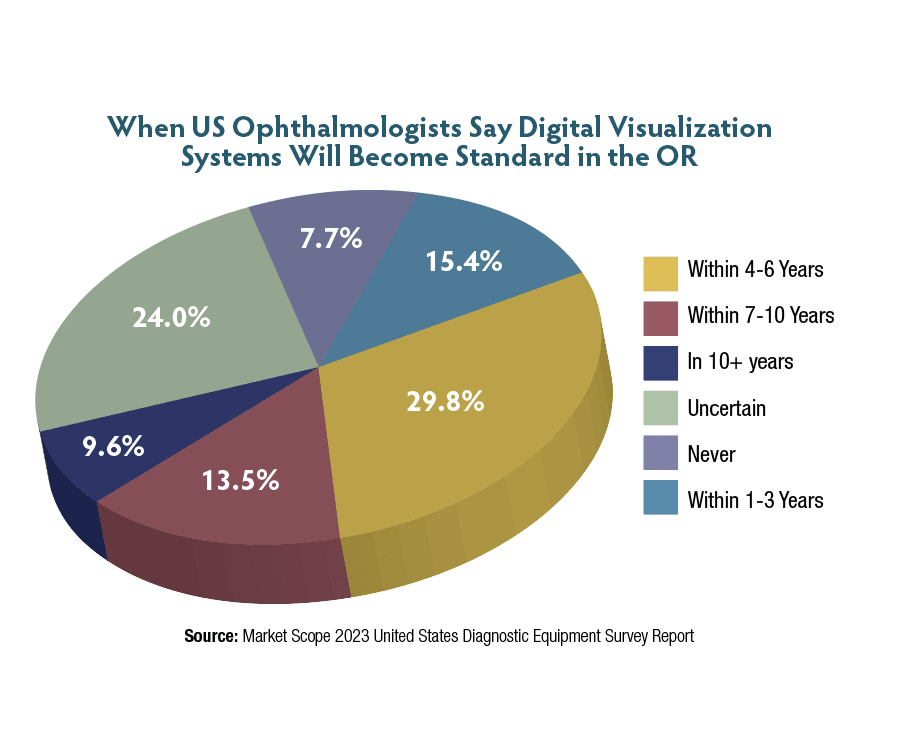
Various studies have shown improved surgeon14,15 and resident11,16 ergonomics with HUDs, an important consideration for surgeon quality of life and longevity. Another advantage is that the HUD allows everyone in the OR to observe in detail how the surgery is progressing, as opposed to the standard operating microscope in which only the surgeon and typically one assistant have adequate visualization. Nurses may be better able to track case progress and anticipate which tools are needed when using the 3D HUD.16
In addition to its clinical benefits, the HUD has the potential to serve as a tool for medical education and training. A survey of medical students found that they preferred learning using a 3D heads-up system in the OR.16 Similarly, when residents were surveyed, they also felt that the HUD improved their understanding of the anatomy.16
There are some limitations to heads-up technology. Firstly, use of a heads-up flat display requires different positioning than at a standard operating microscope, with a direct line of sight to the display often found across from the surgeon or at the foot of the bed. Awkward viewing angles was one of the primary concerns raised in a survey.17 Setup difficulties and lack of access have also been noted.17 Additionally, given the distance from the display screen, the HUD does not provide the same immersive visual field experience of a standard surgical microscope.18 Lastly, there is often a learning curve, although results have varied across studies as to whether surgeries done with the HUD have longer surgical times (in some studies, only longer surgical times were seen initially), and how this lines up with the surgeons’ learning curves.3,4,6,10,19,20
Head-mounted Displays
Similar to HUDs, there have been advances in head-mounted displays, such as Beyeonics One (Beyeonics Vision) and the Head-Mounted System HMS-3000 MT (Sony Electronics) digital visualization platforms.21-23 Beyeonics One, a digitally enhanced surgical visualization system that was adapted from fighter pilot technology, also has the ability to integrate data and images from multiple sources and can include augmented reality to provide real-time guidance based on preoperative imaging data.23 It is approved for use in the United States, as is the HMS-3000.
Head-mounted displays have not yet had as much clinical use as HUDs, largely due to their more recent approval and adoption. The first reports of the use of head-mounted displays in ophthalmology were by Dutra-Meideros et al and Martinez et al in 2017, using the head-mounted system HMS-3000 MT for cataract and vitreoretinal surgery.21,22 Head-mounted displays have been used in cataract surgery as well as vitreoretinal surgery.18,21-23
Similarly to HUDs, head-mounted displays can also allow lower levels of endoillumination than the standard operating microscope, reducing the risk of phototoxicity.24 Also, as with HUDs, head-mounted displays provide ergonomic benefits, but they are even further customizable to each surgeon than HUDs. These also address some of the concerns about HUDs, including awkward viewing angles and a nonimmersive viewing experience. For example, head-mounted displays do not require that the surgeon turn their head to see the image. Moreover, head-mounted displays have the benefit of a close-up, immersive visual field more similar to that of a standard operating microscope.18,23 Another difference is the addition of head gestures, which add another layer of control to the display.18 Head-mounted display technologies continue to evolve and become more accessible, and there is certainly more to come in this arena.
Robot-assisted Surgery
The standard of retinal surgery currently involves the use of manual techniques and instrumentation. Robots for possible use in vitreoretinal surgery, defined as with any machine that can mechanistically augment or replace a surgeon’s manual capabilities,25 can be grouped into 5 different categories: handheld devices that can increase steadiness; comanipulated platforms that can filter tremor but are unable to scale or execute motion; telemanipulation systems that can provide tremor filtering and motion scaling; partially or fully automated instruments; and magnetically controlled microrobots, which lack most of the benefits of robotic assistance but can potentially provide alternate surgical approaches.26-30 There are several motivations for robotic-assisted retinal surgery.25 One motivation is that there are limitations to human control and dexterity, and these restrict the types of surgical manipulations possible while increasing the probability of complications. Despite extensive training and experience, every surgeon will demonstrate microscopic, involuntary tremor movements.31 The retina is a microns-thin structure and can easily be damaged by forces below the ability of human tactile perception.32-34 The introduction of robotic assistance brings a new potential level of precision and control to the OR, for techniques such as retinal vein cannulation, intravascular drug delivery, and subretinal injections.25
An example of a comanipulated platform that can support tremor filtering but not motion scaling is the Johns Hopkins Steady-Hand Eye Robot. This steady-hand manipulator senses forces from the surgeon and transmits these through different modes to produce more precise and tremor-free control, and has been used for retinal vein cannulation and membrane peeling in model eye simulations.25,35 Telemanipulation or remote operator systems involve a separation between the robot controls and the effector machine, and can provide tremor filtering and motion scaling.25 An example of this is the Da Vinci system (Intuitive), used widely in general, gynecologic, and urologic surgery, which has been piloted on porcine eyes for anterior-segment surgery and retinal surgery.36,37 Due to various limitations of Da Vinci for intraocular surgery, including difficulty maneuvering the arms intraocularly and a high center of motion from the eye, the Hexapod Surgical System, a microrobot mounted on the Da Vinci macrorobot, was developed for intraocular surgery.38 Even with this modification, the Da Vinci continued to have issues with translational and rotational maneuvers.38 Other notable examples of telemanipulation systems include the automatable Intraocular Robotic Interventional and Surgical System (IRISS), consisting of 2 joysticks and 2 robotic arms to hold surgical instruments, which has successfully been used to perform vitrectomy with posterior vitreous detachment induction and simulated microcannulation of a temporal retinal vein in porcine eyes.39 The only commercially available robotic surgical system for ophthalmic surgery at this time is the Preceyes Surgical System (Carl Zeiss Meditech), another telemanipulation platform. The Preceyes system consists of a surgeon-controlled joystick and 2 robotic arms secured to the operating table, with an electric-powered headrest to align the robotic arms. This system has a 10-fold to 20-fold increase in precision compared to the human hand,26 and it has been used with success in human patients for membrane peeling and subretinal plasminogen activator injections.26
The trajectory of robotic assistance in retinal surgery is projected to move from dependence on robotic assistance for specific procedural tasks to advancing into semiautonomous surgery. This transition will be facilitated by integrating intelligent sensors that enhance the capabilities of robotic systems; for example, programming in the ability to avoid lens or retina touch during vitrectomy and adding automation of repetitive tasks.
Intraoperative OCT
Optical coherence tomography (OCT) is widely used by retina specialists in preoperative diagnosis and postoperative management of numerous conditions. Intraoperative OCT is a relatively recent innovation that has been particularly helpful for vitreoretinal interface conditions including macular hole surgery, membrane peeling, and repair of vitreomacular traction syndrome, but also in retinal detachment repair and other vitreoretinal surgeries.6,40-43
In terms of the evolution of this technology, intraoperative OCT began with handheld and portable probes for use in the OR. These required surgeons to stop the surgery during measurements, and were not sterile instruments.6 Next, microscope-mounted portable OCT bypassed some of the limitations of handheld and probe-integrated intraoperative OCT. Microscope-mounted intraoperative OCT provided additional stability, improved ease of use, and increased reproducibility of the images.40,41,44 With microscope-mounted intraoperative OCT, the OCT was simply mounted onto the microscope, still requiring surgery to be paused to acquire imaging. The OCT was subsequently integrated into the microscope, allowing for real-time intraoperative OCT.45
Real-time intraoperative OCT has been a breakthrough in integrating this technology into the surgical workflow.41,46 Microscope-integrated OCT systems include the Haag-Streit iOCT system, the Zeiss Rescan 700, and Leica Enfocus. The Determination of Feasibility of Intraoperative Spectral Domain Microscope Combined/Integrated OCT Visualization during En Face Retinal and Ophthalmic Surgery (DISCOVER) study was a prospective study that included the Rescan 700, EnFocus, and another custom iOCT system, again in anterior and posterior segment surgeries. The DISCOVER study found that intraoperative OCT with the microscope-integrated system was feasible in 98% of cases, and altered surgical decision-making in 29% of posterior segment surgeries.47 One emerging use of intraoperative OCT is in subretinal drug delivery; as more gene therapies and other potential treatments are planned for subretinal injection, the precision afforded by intraoperative OCT will prove advantageous.45,48
Intraoperative OCT continues to progress, with the addition of swept-source technology and integration of intraoperative OCT into 3D head-up visual displays,44,49 as well as into robotic systems,50 to use as image guidance.51 Additional refinements in technology allowing automated focusing tracking, as opposed to manual focusing of the image by the surgeon controlling the microscope, as is currently the standard,52 will further streamline the integration of intraoperative OCT in the OR. Given findings that obtaining intraoperative OCT is feasible and can affect surgical decision-making, this technology will likely become widespread in surgical practice in the upcoming years.
Challenges and Future Directions
Although these new technologies provide numerous benefits, it is essential to address associated challenges and considerations. One important barrier to address is the cost. The high costs likely limit accessibility and hinder widespread adoption, particularly in resource-limited healthcare settings. However, a potential way that these technologies can mitigate the possibility for limited access and inequity is through telementoring, in which vitreoretinal procedures can be live-streamed. This could provide a way for surgical mentors not to have to be located in physical proximity to mentees.53,54 This may help train or even assist surgeons in lower resourced areas. Robotic surgery on telemanipulation systems also may someday provide access to retinal surgeries in areas that have fewer retina specialists, because the retina specialist may not need to be located near the patient as long as there is a robotic setup and trained staff nearby.50
Additionally, system inconvenience and the barrier to entry may impede the adoption of these technologies.17 However, as these technologies offer more and more advantages, and use becomes more widespread, more surgeons will likely be trained on them and mentor others in their use.
The above-mentioned technologies — HUDs, head-mounted displays, and robotic-assisted surgery — have the potential to integrate virtual or augmented reality, possibly in conjunction with data from intraoperative OCT. Although other surgical fields are exploring the use of virtual or augmented reality for surgical guidance, this is still in the early phases of study for ophthalmic surgery.55 This is an area that will benefit from additional research to delineate how best virtual and augmented reality can enhance the surgeon’s experience and provide improved patient outcomes.
Conclusions
Retinal surgery is at the forefront of innovation, as shown by the numerous recent developments in OR technology. The digital OR offers surgeons new tools to enhance precision, visualization, and patient outcomes. As these technologies continue to mature, the landscape of retinal surgery is poised for further transformation, ultimately benefiting both surgeons and patients. RP
Hear discussion of this article on the Retina Podcast at retinapodcast.com.
References
- Razavi P, Cakir B, Baldwin G, D'Amico DJ, Miller JB. Heads-up three-dimensional viewing systems in vitreoretinal surgery: an updated perspective. Clin Ophthalmol. 2023;17:2539-2552. doi:10.2147/OPTH.S424229
- Agranat JS, Miller JB, Douglas VP, et al. The scope of three-dimensional digital visualization systems in vitreoretinal surgery. Clin Ophthalmol. 2019;13:2093-2096. doi:10.2147/OPTH.S213834
- Zeng R, Feng Y, Begaj T, Baldwin G, Miller JB. Comparison of the safety and efficacy of a 3-dimensional heads-up display vs a standard operating microscope in retinal detachment repair. J Vitreoretin Dis. 2023;7(2):97-102. doi:10.1177/24741264221150074
- Asani B, Siedlecki J, Schworm B, et al. 3D heads-up display vs standard operating microscope vitrectomy for rhegmatogenous retinal detachment. Front Med (Lausanne). 2020;7:615515. doi:10.3389/fmed.2020.615515
- Modi YS, Ehlers JP. Heads-up vitreoretinal surgery: emerging technology in surgical visualization. Retinal Physician. 2016;13(1):26-29. https://www.retinalphysician.com/issues/2016/janfeb/heads-up-vitreoretinal-surgery-emerging-technology-in-surgical-visualization/
- Talcott KE, Adam MK, Sioufi K, et al. Comparison of a three-dimensional heads-up display surgical platform with a standard operating microscope for macular surgery. Ophthalmol Retina. 2019;3(3):244-251. doi:10.1016/j.oret.2018.10.016
- Kumar A, Hasan N, Kakkar P, et al. Comparison of clinical outcomes between "heads-up" 3D viewing system and conventional microscope in macular hole surgeries: a pilot study. Indian J Ophthalmol. 2018;66(12):1816-1819. doi:10.4103/ijo.IJO_59_18
- Rachitskaya A, Lane L, Ehlers J, DeBenedictis M, Yuan A. Argus II retinal prosthesis implantation using three-dimensional visualization system. Retina. 2019;39 Suppl 1:S199-S200. doi:10.1097/IAE.0000000000002296
- Kantor P, Matonti F, Varenne F, et al. Use of the heads-up NGENUITY 3D Visualization System for vitreoretinal surgery: a retrospective evaluation of outcomes in a French tertiary center. Sci Rep. 2021;11(1):10031. doi:10.1038/s41598-021-88993-z
- Zhang T, Tang W, Xu G. Comparative analysis of three-dimensional heads-up vitrectomy and traditional microscopic vitrectomy for vitreoretinal diseases. Curr Eye Res. 2019;44(10):1080-1086. doi:10.1080/02713683.2019.1612443
- Zhao XY, Zhao Q, Li NN, et al. Surgery-related characteristics, efficacy, safety, and surgical team satisfaction of three-dimensional heads-up system versus traditional microscopic equipment for various vitreoretinal diseases. Graefes Arch Clin Exp Ophthalmol. 2023;261(3):669-679. doi:10.1007/s00417-022-05850-z
- Adam MK, Thornton S, Regillo CD, Park C, Ho AC, Hsu J. Minimal endoillumination levels and display luminous emittance during three-dimensional heads-up vitreoretinal surgery. Retina. 2017;37(9):1746-1749. doi:10.1097/IAE.0000000000001420
- Park SJ, Do JR, Shin JP, Park DH. Customized color settings of digitally assisted vitreoretinal surgery to enable use of lower dye concentrations during macular surgery [published correction appears in Front Med (Lausanne). 2022 Apr 21;9:901546]. Front Med (Lausanne). 2022;8:810070. doi:10.3389/fmed.2021.810070
- Eckardt C, Paulo EB. Heads-up surgery for vitreoretinal procedures: an experimental and clinical study. Retina. 2016;36(1):137-147. doi:10.1097/IAE.0000000000000689
- Weinstock RJ, Diakonis VF, Schwartz AJ, Weinstock AJ. Heads-up cataract surgery: complication rates, surgical duration, and comparison with traditional microscopes. J Refract Surg. 2019;35(5):318-322. doi:10.3928/1081597X-20190410-02
- Shoshany TN, Agranat JS, Armstrong G, Miller JB. The user experience on a 3-dimensional heads-up display for vitreoretinal surgery across all members of the health care team: a survey of medical students, residents, fellows, attending surgeons, nurses, and anesthesiologists. J Vitreoretin Dis. 2020;4(6):459-466. doi:10.1177/2474126420929614
- Tan NE, Wortz BT, Rosenberg ED, Radcliffe NM, Gupta PK. Impact of heads-up display use on ophthalmologist productivity, wellness, and musculoskeletal symptoms: a survey study. J Curr Ophthalmol. 2022;34(3):305-311. doi:10.4103/joco.joco_115_22
- Sorkin N, Levinger E, Achiron A, et al. Use of a three-dimensional head-mounted digital visualization platform in cataract surgery. Eye (Lond). 2023;37(14):2905-2908. doi:10.1038/s41433-023-02427-w
- Romano MR, Cennamo G, Comune C, et al. Evaluation of 3D heads-up vitrectomy: outcomes of psychometric skills testing and surgeon satisfaction. Eye (Lond). 2018;32(6):1093-1098. doi:10.1038/s41433-018-0027-1
- Palácios RM, Maia A, Farah ME, Maia M. Learning curve of three-dimensional heads-up vitreoretinal surgery for treating macular holes: a prospective study. Int Ophthalmol. 2019;39(10):2353-2359. doi:10.1007/s10792-019-01075-y
- Dutra-Medeiros M, Nascimento J, Henriques J, et al. Three-dimensional head-mounted display system for ophthalmic surgical procedures. Retina. 2017;37(7):1411-1414. doi:10.1097/IAE.0000000000001514
- Martínez-Toldos JJ, Fernández-Martínez C, Navarro-Navarro A. Experience using a 3D head-mounted display system in ophthalmic surgery. Retina. 2017;37(7):1419-1421. doi:10.1097/IAE.0000000000001664
- Barak A, Loewenstein A, Schneider R, Bakalash SH. Primary results of clinical use of the Beyeonics digitally enhanced surgical visualization system in cataract and vitreoretinal surgery: a prospective study. Invest Ophthalmol Vis Sci. 2019;60:PB0170.
- Loewestein A, Schneider R, Barak A. First look: a head-mounted OR display. Rev Ophthalmol. 2019. Accessed April 8, 2024. https://www.reviewofophthalmology.com/article/first-look-a-headmounted-or-display
- Zhu I, Mieler WF. Robotic retinal surgery. In: Chang A, Mieler WF, Ohji M, eds. Macular Surgery: Current Practice and Trends. Singapore: Springer; 2020:565–574.
- Edwards TL, Xue K, Meenink HCM, et al. First-in-human study of the safety and viability of intraocular robotic surgery. Nat Biomed Eng. 2018;2:649-656. doi:10.1038/s41551-018-0248-4
- Sadeghi E, Mohan S, Iannetta D, Chhablani J. Recent developments in imaging and surgical vision technologies currently available for improving vitreoretinal surgery: a narrative review. Expert Rev Med Devices. 2023;20(8):651-672. doi:10.1080/17434440.2023.2231841
- Ramamurthy SR, Dave VP. Robotics in vitreo-retinal surgery. Sem Ophthalmol. 2022;37:795-800. doi:10.1080/08820538.2022.2075705
- Roizenblatt M, Edwards TL, Gehlbach PL. Robot-assisted vitreoretinal surgery: current perspectives. Robot Surg 2018;5:1–11.
- de Smet MD, Naus GJL, Faridpooya K, Mura M. Robotic-assisted surgery in ophthalmology. Curr Opin Ophthalmol. 2018;29(3):248-253. doi:10.1097/ICU.0000000000000476
- Xue K, Edwards TL, Meenink HCM, et al. Robot-assisted retinal surgery: overcoming human limitations. In: Ohji M, ed. Surgical Retina. Retina Atlas. Singapore: Springer; 2019:109-114. doi:10.1007/978-981-13-6214-9_11
- Gonenc B, Balicki MA, Handa J, et al. Evaluation of a micro-force sensing handheld robot for vitreoretinal surgery. Rep U S. 2012;2012:4125-4130. doi:10.1109/IROS.2012.6385715
- Vander Poorten E, Riviere CN, Abbott JJ, et al. Robotic retinal surgery. In: Abedin-Nasab MH, ed. Handbook of Robotic and Image-Guided Surgery. Elsevier; 2020:627-672.
- Gupta PK, Jensen PS, de Juan E. Surgical forces and tactile perception during retinal microsurgery. In: Taylor C, Colchester A, eds. Medical Image Computing and Computer-Assisted Intervention – MICCAI’99. Lecture Notes in Computer Science. Berlin, Heidelberg: Springer; 1999:1218-1225.
- Mitchell B, Koo J, Iordachita I, et al. Development and application of a new steady-hand manipulator for retinal surgery. In: Proceedings 2007 IEEE International Conference on Robotics and Automation. 2007;623-629.
- Bourla DH, Hubschman JP, Culjat M, Tsirbas A, Gupta A, Schwartz SD. Feasibility study of intraocular robotic surgery with the da Vinci surgical system. Retina. 2008;28(1):154-158. doi:10.1097/IAE.0b013e318068de46
- Tsirbas A, Mango C, Dutson E. Robotic ocular surgery. Br J Ophthalmol. 2007;91(1):18-21. doi:10.1136/bjo.2006.096040
- Bourges JL, Hubschman JP, Wilson J, Prince S, Tsao TC, Schwartz S. Assessment of a hexapod surgical system for robotic micro-macro manipulations in ocular surgery. Ophthalmic Res. 2011;46(1):25-30. doi:10.1159/000314719
- Rahimy E, Wilson J, Tsao TC, Schwartz S, Hubschman JP. Robot-assisted intraocular surgery: development of the IRISS and feasibility studies in an animal model. Eye (Lond). 2013;27(8):972-978. doi:10.1038/eye.2013.105
- Ehlers JP. Intraoperative optical coherence tomography: past, present, and future. Eye (Lond). 2016;30(2):193-201. doi:10.1038/eye.2015.255
- Carrasco-Zevallos OM, Viehland C, Keller B, et al. Review of intraoperative optical coherence tomography: technology and applications [Invited]. Biomed Opt Express. 2017;8(3):1607-1637. doi:10.1364/BOE.8.001607
- Lee LB, Srivastava SK. Intraoperative spectral-domain optical coherence tomography during complex retinal detachment repair. Ophthalmic Surg Lasers Imaging. 2011;42 Online:e71-e74. doi:10.3928/15428877-20110804-05
- Obeid A, Ehmann D, Adam M, et al. Comparison of residual subfoveal fluid by intraoperative oct after macula-involving rrd repair using direct drainage, drainage retinotomy, or perfluoro-n-octane. Ophthalmic Surg Lasers Imaging Retina. 2019;50(8):497-503. doi:10.3928/23258160-20190806-04
- Talcott KE, Ehlers JP. Intraoperative OCT. In: Albert D, Miller J, Azar D, Young LH, eds. Albert and Jakobiec’s Principles and Practice of Ophthalmology. Cham: Springer International Publishing; 2020:1-14.
- Ehlers JP, Petkovsek DS, Yuan A, Singh RP, Srivastava SK. Intrasurgical assessment of subretinal tPA injection for submacular hemorrhage in the PIONEER study utilizing intraoperative OCT. Ophthalmic Surg Lasers Imaging Retina. 2015;46(3):327-332. doi:10.3928/23258160-20150323-05
- Seider MI, Carrasco-Zevallos OM, Gunther R, et al. Real-time volumetric imaging of vitreoretinal surgery with a prototype microscope-integrated swept-source OCT device. Ophthalmol Retina. 2018;2(5):401-410. doi:10.1016/j.oret.2017.08.023
- Ehlers JP, Modi YS, Pecen PE, et al. The DISCOVER study 3-year results: feasibility and usefulness of microscope-integrated intraoperative OCT during ophthalmic surgery. Ophthalmology. 2018;125(7):1014-1027. doi:10.1016/j.ophtha.2017.12.037
- Poh SSJ, Sia JT, Yip MYT, et al. Artificial intelligence, digital imaging, and robotics technologies for surgical vitreoretinal diseases. Ophthalmol Retina. 2024 Jan 26:S2468-6530(24)00044-7. doi:10.1016/j.oret.2024.01.018
- Cai S, Therattil A, Vajzovic L. Pediatric vitreoretinal surgery and integrated intraoperative optical coherence tomography [published correction appears in Dev Ophthalmol. 2021 Apr 16;61:25]. Dev Ophthalmol. 2021;61:15-25. doi:10.1159/000511818
- Channa R, Iordachita I, Handa JT. Robotic vitreoretinal surgery. Retina. 2017;37(7):1220-1228. doi:10.1097/IAE.0000000000001398
- Cereda MG, Parrulli S, Douven YGM, et al. Clinical evaluation of an instrument-integrated OCT-based distance sensor for robotic vitreoretinal surgery. Ophthalmol Sci. 2021;1(4):100085. doi:10.1016/j.xops.2021.100085
- Ciarmatori N, Pellegrini M, Nasini F, Talli PM, Sarti L, Mura M. The state of intraoperative OCT in vitreoretinal surgery: recent advances and future challenges. Tomography. 2023;9(5):1649-1659. doi:10.3390/tomography9050132
- Seddon IA, Rahimy E, Miller JB, Charles S, Kitchens J, Houston SK 3rd. Feasibility and potential for real-time 3D vitreoretinal surgery telementoring. Retina. 2023;43(12):2162-2165. doi:10.1097/IAE.0000000000003656
- Lu ES, Reppucci VS, Houston SKS 3rd, Kras AL, Miller JB. Three-dimensional telesurgery and remote proctoring over a 5G network. Digit J Ophthalmol. 2021;27(3):38-43. doi:10.5693/djo.01.2021.06.003
- Iskander M, Ogunsola T, Ramachandran R, McGowan R, Al-Aswad LA. Virtual reality and augmented reality in ophthalmology: a contemporary prospective. Asia Pac J Ophthalmol (Phila). 2021;10(3):244-252. doi:10.1097/APO.0000000000000409