Retinal prosthesis devices, also known as bionic eyes, are implantable electronic devices designed to convert light into electrical signals and transmit them to retinal tissue, the optic nerve, or the brain. These devices aim to restore vision to individuals with retinal diseases ranging from age-related causes from macular degeneration to inherited retinal diseases such as retinitis pigmentosa (RP). Irreversible loss of vision impacts quality of life and hinders the ability to perform activities of daily living. With technological advancements, patients may have the potential to regain both partial sight and a sense of independence. Patients with severe visual impairment or blindness may have the opportunity to see phosphenes, and, in the best cases, identify objects, read large letters, and navigate walking paths. This article will provide an up-to-date overview of the different types of retinal prostheses based on anatomic location (Table 1).
Table 1. Current Status of Retina Prosthesis Devices
DEVICE NAME
|
CURRENT STATUS |
---|---|
EPIRETINAL DESIGNS |
|
IMIE 256 (Channel Intelligent Micro Implant Eye)
|
The first human clinical testing occurred on 5 patients with end-stage retinitis pigmentosa. All patients underwent visual rehabilitation for 90 days and visual performance was evaluated using grating/Tumbling E visual acuity test, direction of motion, square localization, and orientation/mobility test. Results were favorable and all patients performed significantly better on all performance evaluations using the IMIE 256. Only 1 reported adverse event occurred in 1 patient with electrode array movement and low intraocular pressure.4 Future clinical trials with larger sample size and testing duration are under way. |
EPI-RET 3
|
This device was tested on 6 patients who were legally blind from retinitis pigmentosa. It was subsequently removed 1 month later per the ethics committee guidelines, due to being an experimental device. No unexpected alterations or adverse events were observed in any of the eyes. Quality of life did not worsen with the addition of the device and visual acuity ranged from light perception to hand motion.6 |
NR600 System
|
The initial trial began on January 17, 2020, and 9 patients underwent the implantation procedure. This project was recently terminated at the end of 2023 due to lack of funding, according to clinicaltrials.gov. |
Argus II
|
Argus II was the first FDA-approved retinal prosthesis. The initial trial began in 2006 to study the safety and efficacy in patients with end-stage retinitis pigmentosa. Since 2007, more than 350 patients were implanted with the Argus II. In 2020, Second Sight stopped its production due to financial dif-ficulties. |
Polyretina
|
Ex-vivo and in-vivo studies demonstrated the capability to activate retinal ganglion cells at a safe irradiance level.5 Overall, preclinical studies found no evidence of cytotoxicity, in addition to having a long lifetime of 2 years.5 |
SUBRETINAL DESIGNS |
|
Prima
|
In the PRIMAvera study, an open-label, multicenter, prospective, single-arm pivotal trial, a total of 38 patients were implanted with Prima system. The study aimed to confirm the safety and clinical benefits provided by the Prima system.10 Most recently, a 4-year follow-up of 5 patients with geographic atrophy confirmed safety of the Prima system and demonstrated an improve-ment in visual acuity of up to 8 ETDRS lines.13 |
IMTC HARP4k
|
The retinal prosthetic is currently undergoing modifications for further devel-opment. A computer stimulation has demonstrated the safety of the device in minimizing the amount of mechanical stress on the retinal tissue, decreasing the potential risk for retinal tears.11 |
The Boston Retinal Implant Project
|
This wireless power implant consists of 256+ independently configurable retinal stimulation channels. The integrated circuit chip is packaged in a titanium case attached to the sclera. Only a thin flexible electrode array enters the eye, and it is placed in the subretinal space. |
SUPRACHOROIDAL DESIGNS |
|
Bionic Eye System (generation 2)
|
The results of a phase 2 clinical trial of generation 2 in 4 patients have demonstrated the safety of this device with meaningful improvements in vi-sion based on screen-based, functional assessments. The device is currently undergoing further modifications with the hopes of improving visual acuity. |
Suprachoroidal-transretinal stimulation
|
A study in 3 patients has found a favorable safety profile but with variable functional results. |
Phoenix99
|
Phoenix99 has successfully completed in vivo studies in a bovine model, which demonstrated the safety of the device. |
OPTIC DISC DESIGN |
|
Artificial vision by direct optic nerve electrode (AV-DONE)
|
A clinical trial completed in 1 patient with a favorable safety profile in 2009. However, the original design became dislodged and required reinsertions. To address this concern, the group designed new device comprising of 7 stimu-lation electrodes and 1 reference electrode to facilitate 1-step implantation of the electrode tips into the disc and has demonstrated successful implantation in animal models as well as in 1 human volunteer.16,17 |
CORTICAL DESIGNS |
|
Orion visual cortex prosthetics system
|
The Orion visual cortical prosthesis system completed the fifth year of its early feasibility study in 6 blind patients implanted with the device. Three subjects are still participating in the study and continue to use the system.22 |
Cortical Vision Neuroprosthesis for the Blind
|
An early study in monkeys demonstrated successful elicitation of visual potential. A human trial in 1 patient demonstrated consistent stimulation thresholds. Further trials are under way. |
Intracortical Visual Prosthesis
|
A prototype was successfully implanted in 1 blind patient with a favorable safety profile. The company is currently recruiting for its phase 1 clinical trial. |
Devices
Epiretinal Devices
Epiretinal prostheses capture images through an external camera that transmits the information to a video processor unit (VPU). This transmission is then converted into an electrical signal and sent to an electrode array implanted on top of the retina. A series of impulses is generated within the ganglion cell layer for the epiretinal configuration.1 The device is surgically placed in the inner retinal surface, near the ganglion cells, which is a simpler procedure than a subretinal implant.2 Challenges can arise in fixating the array and the possible distorted mapping caused by stimulation of the ganglion cell axons. Current devices include the Argus II (Second Sight Medical Products), NanoRetina 600 System (NR600; Nano Retina), 256 Channel Intelligent Micro Implant Eye (IMIE 256; Golden Eye Bionic and IntelliMicro Medical, Figure 1), Polyretina (Dr. Ghezzi’s research team), and EPI-RET 3 (RWTH Aachen University). These devices use both internal and external components with wireless communication, although the internal components are often connected by a transscleral connection.
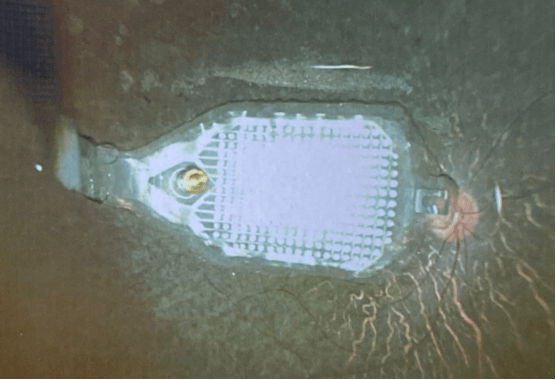
Each device has unique features. The NR600 has an array of intraretinal penetrating microelectrodes powered wirelessly by an infrared beam that controls the stimulation parameters.3 The IMIE consists of an external VPU component and 3 internal components: an episcleral electronic implant, a transscleral cable, and a retinal electrode array of 256 electrodes.4 The Polyretina has moldable plasticity, allowing for full retinal surface coverage.5 The EPI-RET 3 incorporates a complete intraocular internal component and eliminates the transscleral connection. In this model, the transmitter coil is mounted on a pair of glasses and the receiver coil is placed within an intraocular lens along with 2 microchips that interpret the stimulation signal and produce the stimulation pulses to an epiretinal flexible microcable containing 25 microelectrodes.6
The Argus II was the first and only FDA-approved retina prosthesis. This device restores partial functional vision in patients with bare to no light perception due to advanced RP. It consists of an external video camera mounted in a pair of glasses that captures the images and sends them to a VPU, where the images are transformed into electrical impulses that are transmitted wirelessly using an antenna to an electrode array sitting on the macula. The electrical pulses stimulate the inner retinal cells. The most commonly reported adverse events were hypotony, conjunctival dehiscence, presumed endophthalmitis, and conjunctival erosion. More than 350 patients were implanted worldwide with the Argus II; however, in 2019, Second Sight discontinued its retinal implant due to financial difficulties (Figure 2).7
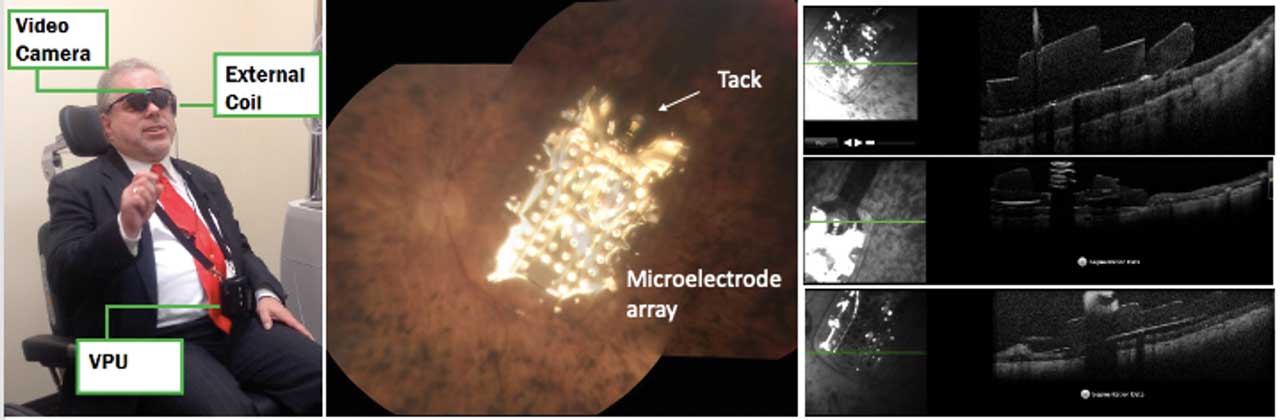
Subretinal Devices
Subretinal implantation is a more invasive approach than epiretinal implantation. The device is often positioned under the retina, in the remnants of the retinal pigmented epithelial (RPE) or photoreceptor layers. Placement stimulates the intact middle retinal processing layers (amacrine, horizontal, and bipolar cells). This approach takes advantage of the existing neural processing to stimulate ganglion cells. With this approach, the implant can be held without a tack.8 Placing the implant in the subretinal space might require a posterior retinotomy and temporarily detaching the retina by injecting a balanced salt solution and/or Healon in the subretinal space. These challenges could limit the implant size. In addition, the implant itself may block the fluid communication between the retina and the choroid, and there may be problems with heat dissipation.9 Current models include the Photovoltaic Retinal Implant (Prima) bionic vision system (Pixium Vision; Figure 3), the HARP4K Retinal Prosthesis System (Iridium Medical Technology Company) and the Boston Retinal Implant (Boston Retinal Implant Project, commercial partners Bionic Eye Technologies, Inc. and Visus Technology).10-12 The Prima design consists of a 2x2 photovoltaic subretinal implant, which is powered by pulsed near-infrared light through a small digital projector. It is integrated into a pair of glasses. It can capture visual environmental scenes and translate them from light pulsations to electrical currents for retinal cell excitation and visual processing.10 A recent preliminary study demonstrated that implantation of Prima was not only feasible but well tolerated with no reduction of natural peripheral vision in 5 patients with geographic atrophy at 4-year follow-up.13 The study also showed an improvement of up to 8 ETDRS lines in visual acuity.
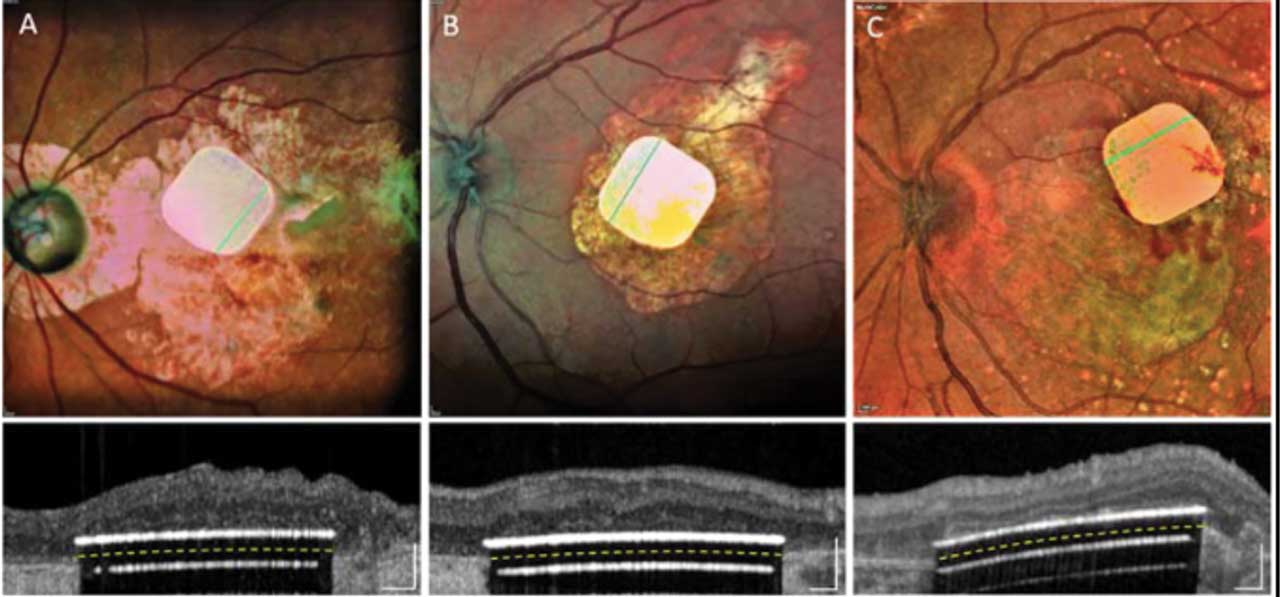
Suprachoroidal Devices
Suprachoroidal devices are implanted between the choroid and sclera. Because these devices do not enter the vitreous cavity, they are less invasive and minimize the risk of retinal damage. However, devices in this location require a larger stimulation power due to the increased distance from the neurosensory retina. Additionally, these devices carry a significant risk of hemorrhage, as the choroid is well vascularized.9 Models currently being tested are Generation 2 by Bionic Vision Australia (consisting of 44 platinum disc electrodes that send signals to postauricular headpiece), Phoenix99 by Bionics Institute (consisting of 98 stimulation sites returned by 1 common electrode), and suprachoroidal-transretinal stimulation by Osaka University Graduate School of Medicine (consisting of a 49-microelectrode array implanted into a scleral pocket).14-16
These devices are successful in restoring some functional vision without significant adverse events. Preliminary data suggest that suprachoroidal devices are more limited in vision regained compared to their epiretinal and subretinal counterparts, because the greater spread of current reduces spatial resolution in final perceived vision.17
Optic Nerve Stimulation
Artificial vision by direct optic nerve electrode (AV-DONE) is an electrode-utilizing device that is implanted into the optic disc.18,19 In 1 human volunteer, using a definition of positive perception of phosphene when more than 50% of the test was positive for perception, the subject reported localized phosphene perception via stimulation of the optic disc electrode.19 However, due to the small surface area of the optic disc, the electrode array became dislodged easily and required several repeat insertions. To address this concern, the same group in Japan developed a second-generation model that allows better fixation through the use of 7 stimulation electrodes and 1 reference electrode with 1-step implantation; this latest model has been tested in rabbit models and more recently in 1 human volunteer.18 There were no complications associated with the device. The human volunteer perceived phosphenes in 6 of the 7 electrodes implanted.
Cortical Devices
Cortical devices directly stimulate the visual cortex of the occipital lobe of the brain. By bypassing the compromised ocular anatomy, cortical devices offer the advantage of being protected from retinal damage or atrophy and corneal or lens opacities. The utility of these devices is not limited to vision loss secondary to retinal degeneration; they are intended for patients with vision loss due to glaucoma, diabetic retinopathy, optic nerve injury or disease, ocular cancer, and ocular trauma. There are currently 4 models under investigation. The Orion Visual Cortex Prosthetics system (Cortigent [Vivani Medical Inc.]) consists of a subdural electrode grid implant with 60 electrodes implanted on the medial surface of the occipital lobe, a receiver coil, and an internal circuit.20-22 A second-generation system is being developed for use in a wide array of retinal pathologies. The Cortical Vision Neuroprosthesis for the Blind (Biomedical Technologies) comprises an electrode implant in the intracortical layer.23 The Intracortical Visual Prosthesis (Illinois Institute of Technology) stimulates the brain through floating microelectrode arrays that receive its power and information signal wirelessly so that no connectors traverse the scalp.24 Early data for all cortical devices offer promising results in restoring some degree of sight effectively. Further studies evaluating the safety profiles, as well as the full extent of utility, are under way.
Future Directions
Since the first human retinal prosthetic implantation in 2002, every subsequent step has been met with something novel and exciting.25 It is remarkable that it took 20 years for the first clinically approved retinal prosthesis implantation since the first human test of retinal electrical stimulation in a blind patient with RP. However, it is important to remember that vision regained from these devices has been modest at best and largely remains well below the threshold of the legal definition of blindness. Future retina prosthesis devices will need to provide an increased safety profile, increased effectiveness in terms of visual function, and decreased cost compared to prior devices.
Nonetheless, there has been continued interest in discovering new ways to stimulate different cells or pathways to restore sight as the understanding of visual neurosciences advances.9 As of February 2024, 14 active clinical trials are focusing on the use of stem cell therapy to repair retinal tissue and improve visual acuity, and 18 active clinical trials are exploring gene therapy for RP.26 Recent advances in gene therapy and stem cell therapy have potential to restore sight, but major challenges remain. For one, adverse effects of stem cell therapy described in the literature include inflammatory response, carcinogenesis through suppression of tumor suppressor genes or stimulation of oncogenes, and retinal detachment.9 Adverse effects of gene therapy described in the literature include inflammatory responses, increased intraocular pressures, loss of retinal layers, and toxicity in the photoreceptor and RPE layers.27 Regardless, the patient population who benefits from retinal prostheses is different from those who undergo gene therapy. The former patients are in much later disease stages.
Another area of interest has been the role of artificial intelligence in optimizing retinal prostheses and the visual processing algorithm.28 Retinal prosthetics often include different parts (eg, image sensor, processing unit, and implanted photostimulator) that need to be optimized and linked for maximal visual benefit. Recently, progress has been made in the field of processing algorithms for retinal prosthetics, where the retina’s working principle is combined with computer vision models. There is continued interest in this area with the understanding of machine learning and computer algorithms advance.28
Conclusion
This review describes 15 devices that have been considered in the last 2 decades since the conceptualization of the retinal prosthesis. The field of retina prostheses remains challenging. Many of these devices have achieved some visual restoration in animal and human models, but visual gains have been modest at best, limiting their clinical use. Continued progress is needed, but there are promising data that may translate to meaningful vision gain for patients. RP
Hear discussion of this article on the Retina Podcast at retinapodcast.com.
References
1. Ghezzi D. Retinal prostheses: progress toward the next generation implants. Front Neurosci. 2015;9:290. doi:10.3389/fnins.2015.00290
2. Nowik K, Langwińska-Wośko E, Skopiński P, Nowik KE, Szaflik JP. Bionic eye review - an update. J Clin Neurosci. 2020;78:8-19. doi:10.1016/j.jocn.2020.05.041
3. Yanovitch L, Raz-Prag D, Hanein Y. A new high-resolution three-dimensional retinal implant: system design and preliminary human results. Preprint in bioRxiv. doi:10.1101/2022.09.14.507901
4. Xu H, Zhong X, Pang C, et al. First human results with the 256 channel intelligent micro implant eye (IMIE 256). Transl Vis Sci Technol. 2021;10(10):14. doi:10.1167/tvst.10.10.14
5. Ferlauto L, Airaghi Leccardi MJI, Chenais NAL, et al. Design and validation of a foldable and photovoltaic wide-field epiretinal prosthesis. Nat Commun. 2018;9(1):992. doi:10.1038/s41467-018-03386-7
6. Menzel-Severing J, Laube T, Brockmann C, et al. Implantation and explantation of an active epiretinal visual prosthesis: 2-year follow-up data from the EPIRET3 prospective clinical trial. Eye (Lond). 2012;26(4):501-509. doi:10.1038/eye.2012.35
7. Ostad-Ahmadi Z, Daemi A, Modabberi MR, Mostafaie A. Safety, effectiveness, and cost-effectiveness of Argus II in patients with retinitis pigmentosa: a systematic review. Int J Ophthalmol. 2021;14(2):310-316. doi:10.18240/ijo.2021.02.20
8. Loewenstein JI, Montezuma SR, Rizzo JF. Outer retinal degeneration: an electronic retinal prosthesis as a treatment strategy. Arch Ophthalmol. 2004;122(4):587-596. doi:10.1001/archopht.122.4.587
9. Ramirez KA, Drew-Bear LE, Vega-Garces M, Betancourt-Belandria H, Arevalo JF. An update on visual prosthesis. Int J Retin Vitr. 2023;9(1):73. doi:10.1186/s40942-023-00498-1
10. Pixium Vision SA. PRIMA US-feasibility study in atrophic dry AMD (PRIMA-FS-US). Clinicaltrials.gov identifier: NCT03392324. Updated January 24, 2024. Accessed April 4, 2024. https://clinicaltrials.gov/study/NCT03392324
11. Iridium Medical Technology Company. Accessed March 10, 2024. http://www.irmedtech.com/index_en.html
12. The Boston Retinal Implant Project. Accessed March 10, 2024. https://www.bostonretinalimplant.org
13. Muqit M, Mer YL, de Koo LO, Holz FG, Sahel JA, Palanker D. Prosthetic visual acuity with the PRIMA system in patients with atrophic age-related macular degeneration at 4 years follow-up. Preprint in medRxiv. 2023;2023.11.12.23298227. doi:10.1101/2023.11.12.23298227
14. Petoe MA, Titchener SA, Kolic M, et al. A second-generation (44-channel) suprachoroidal retinal prosthesis: interim clinical trial results. Transl Vis Sci Technol. 2021;10(10):12. doi:10.1167/tvst.10.10.12
15. Eggenberger SC, James NL, Ho C, et al. Implantation and long-term assessment of the stability and biocompatibility of a novel 98 channel suprachoroidal visual prosthesis in sheep. Biomaterials. 2021;279:121191. doi:10.1016/j.biomaterials.2021.121191
16. Fujikado T, Kamei M, Sakaguchi H, et al. Testing of semichronically implanted retinal prosthesis by suprachoroidal-transretinal stimulation in patients with retinitis pigmentosa. Invest Ophthalmol Vis Sci. 2011;52(7):4726-4733. doi:10.1167/iovs.10-6836
17. Bloch E, Luo Y, da Cruz L. Advances in retinal prosthesis systems. Ther Adv Ophthalmol. 2019;11:2515841418817501. doi:10.1177/2515841418817501
18. Nishida K, Sakaguchi H, Kamei M, et al. Visual sensation by electrical stimulation using a new direct optic nerve electrode device. Brain Stimul. 2015;8(3):678-681. doi:10.1016/j.brs.2015.03.001
19. Sakaguchi H, Kamei M, Fujikado T, et al. Artificial vision by direct optic nerve electrode (AV-DONE) implantation in a blind patient with retinitis pigmentosa. J Artif Organs. 2009;12(3):206-209. doi:10.1007/s10047-009-0467-2
20. Wang V, Kuriyan AE. Optoelectronic devices for vision restoration. Curr Ophthalmol Rep. 2020;8(2):69-77. doi:10.1007/s40135-020-00232-2
21. Harris M. Second Sight´s implant technology gets a second chance. IEEE Spectrum. August 15, 2023. Accessed April 13, 2024. https://spectrum.ieee.org/bionic-eye
22. Vivani Medical, Inc. Subsidiary Cortigent reports 5-year early feasibility study update for the Orion visual cortical prosthesis system. July 11, 2023. Accessed April 13, 2024. https://investors.vivani.com/investors/news-events/press-releases/detail/161/vivani-medical-inc-subsidiary-cortigent-reports-5-year
23. Cortical Visual Prothesis for the Blind. Accessed April 13, 2024. http://biomedical-technologies.com/index-2.html
24. The Chicago Lighthouse. Intracortical Visual Prosthesis Project (ICVP). Accessed February 11, 2024. https://chicagolighthouse.org/icvp/
25. Dagnelie G. Retinal implants: emergence of a multidisciplinary field. Curr Opin Neurol. 2012;25(1):67-75. doi:10.1097/WCO.0b013e32834f02c3
26. Hosseini Shabanan S, Seyedmirzaei H, Barnea A, Hanaei S, Rezaei N. Stem cell transplantation as a progressing treatment for retinitis pigmentosa. Cell Tissue Res. 2022;387(2):177-205. doi:10.1007/s00441-021-03551-3
27. Drag S, Dotiwala F, Upadhyay AK. Gene therapy for retinal degenerative diseases: progress, challenges, and future directions. Invest Ophthalmol Vis Sci. 2023;64(7):39. doi:10.1167/iovs.64.7.39
28. Wang C, Fang C, Zou Y, Yang J, Sawan M. Artificial intelligence techniques for retinal prostheses: a comprehensive review and future direction. J Neural Eng. 2023;20(1):10.1088/1741-2552/acb295. doi:10.1088/1741-2552/acb295