Over the past decades, new imaging modalities have emerged for the diagnosis of geographic atrophy (GA) secondary to age-related macular degeneration (AMD) (Figure 1). These advances have the potential to improve disease monitoring, allowing clinicians to tailor care to individual patients more effectively than ever before. Yet the variety of imaging options introduces greater complexity into the selection of the most appropriate imaging technique for the clinical scenario.
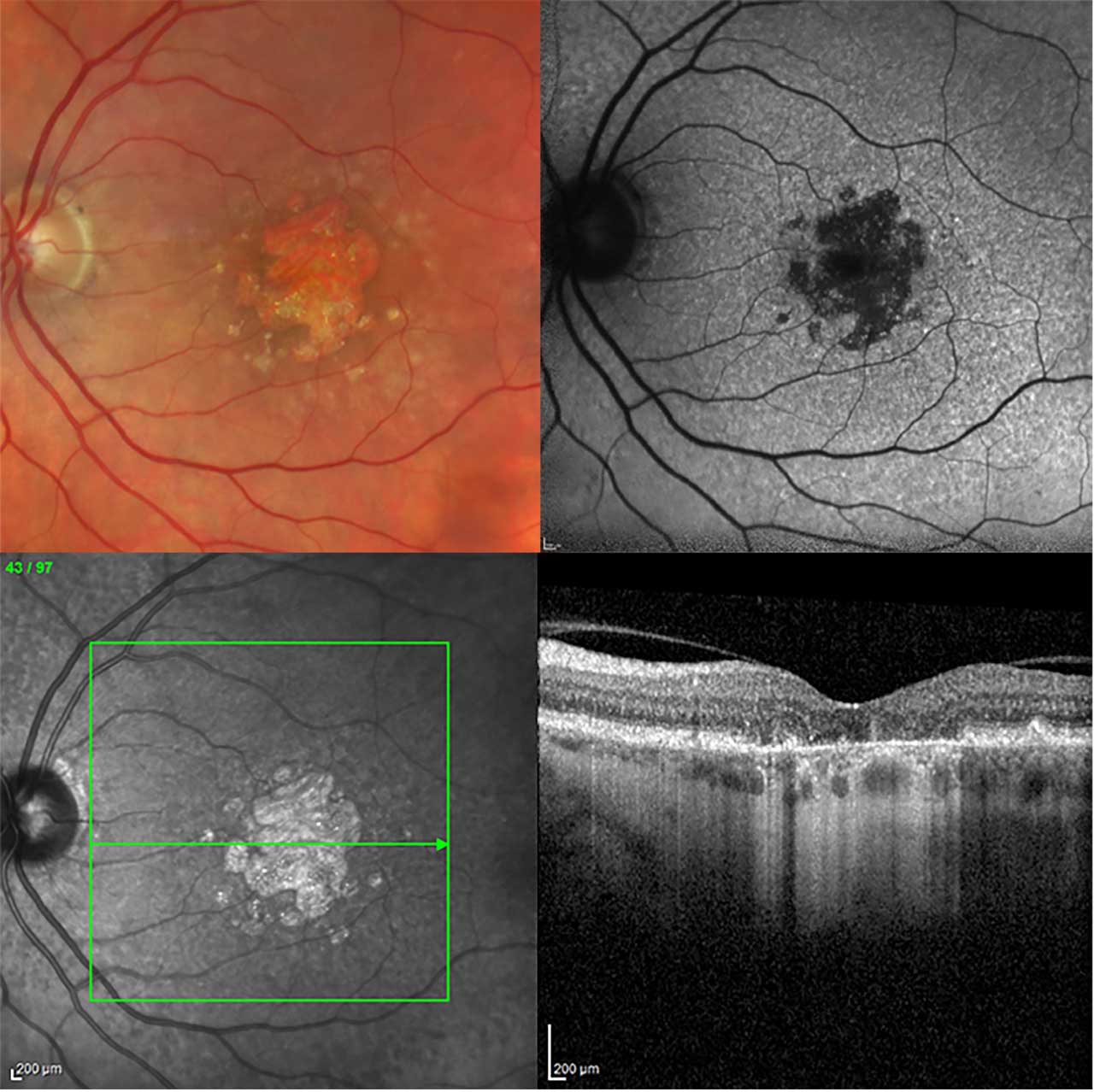
Background
Age-related macular degeneration represents a wide disease spectrum containing a complex interaction involving age, environmental risk factors, and genetic predisposition. It is characterized by extracellular deposition and accumulation of lipofuscin, specifically drusen, leading to photoreceptor and RPE loss, which thus results in subsequent central scotoma. Complete disease pathogenesis has yet to be fully unveiled, although strong implications have been found with chronic inflammation, lipid deposition, oxidative stress, and impaired extracellular matrix maintenance. Clinical classification of the disease is predominantly conducted via drusen size and the presence of GA and/or macular neovascularization (MNV). Geographic atrophy is represented through the concurrent and often progressive atrophy of retinal photoreceptors, RPE, and choriocapillaris.1,2
Color Fundus Photography
Historically, color fundus photography (CFP) has been the standard for imaging in AMD. CFP closely correlates with clinical ophthalmoscopy. It can detect a wide range of characteristic changes in AMD, including drusen, atrophy, fibrosis, and focal pigmentary changes. Although CFP is useful for identifying areas of atrophy and growth over time, it has limited precision in demarcating lesion margins given the lack of contrast. This is particularly true for small or multifocal lesions and limits its ability to quantify GA. Despite these shortcomings, CFP remains the standard validated imaging modality for detection of focal pigmentary changes and merits continued use as other imaging modalities are further validated.2,3
Fundus Autofluorescence
Fundus autofluorescence (FAF) provides high-contrast retinal images, allowing for better visualization of atrophy margins. Fundus autofluorescence signal intensities are highest in lipofuscin-rich areas, because lipofuscin contains fluorophores responsible for FAF. Lipofuscin, along with retinal pigment epithelium (RPE), is lost in areas of atrophy with resultant low signal intensities. The contrast between low and high signal intensities at the border of lesions permits more precise quantification of atrophy than with CFP. This allows clinicians to closely track lesion growth across visits.2,4 To achieve reasonable quality FAF images, the patient’s pupils need to be dilated.
The type of FAF imaging used most commonly relies on blue light excitation and is associated with some discomfort for patients.4 The quality of blue light signals can be affected by factors such as nuclear lens opacities, vitreous floaters, and pupil dilation. Further, macular pigment blocks blue light, meaning atrophic borders near the fovea may be more difficult to clearly visualize. Recent research has attempted to address this problem with longer wavelength (ie, green) light. Green light is less sensitive to optical medial opacities and macular pigment, theoretically minimizing the disadvantages of FAF imaging.5,6 More studies are needed to validate the use of green light in FAF. Overall, the ability of FAF to detect changes in atrophy makes it an essential component of dry AMD monitoring, and FAF should be included in regular follow up of patients.
RegionFinder, which is available on the Spectralis imaging platform (Heidelberg Engineering Inc.), allows for automated tracking of GA.7 RegionFinder provides semiautomatic quantification of the area of GA based on blue laser autofluorescence imaging that can be acquired through the Spectralis product family (Figure 2). The image processing software offers accurate, reproducible, and time-efficient identification and quantification of outer retinal atrophy and its progression over time.7 Alternative image analysis tools to segment GA areas and assess GA progression over time, along with additional features such as prediction of future GA enlargement, are currently under development (eg, RetinAI and Retinsight) but these have not yet become broadly available in the clinical setting.
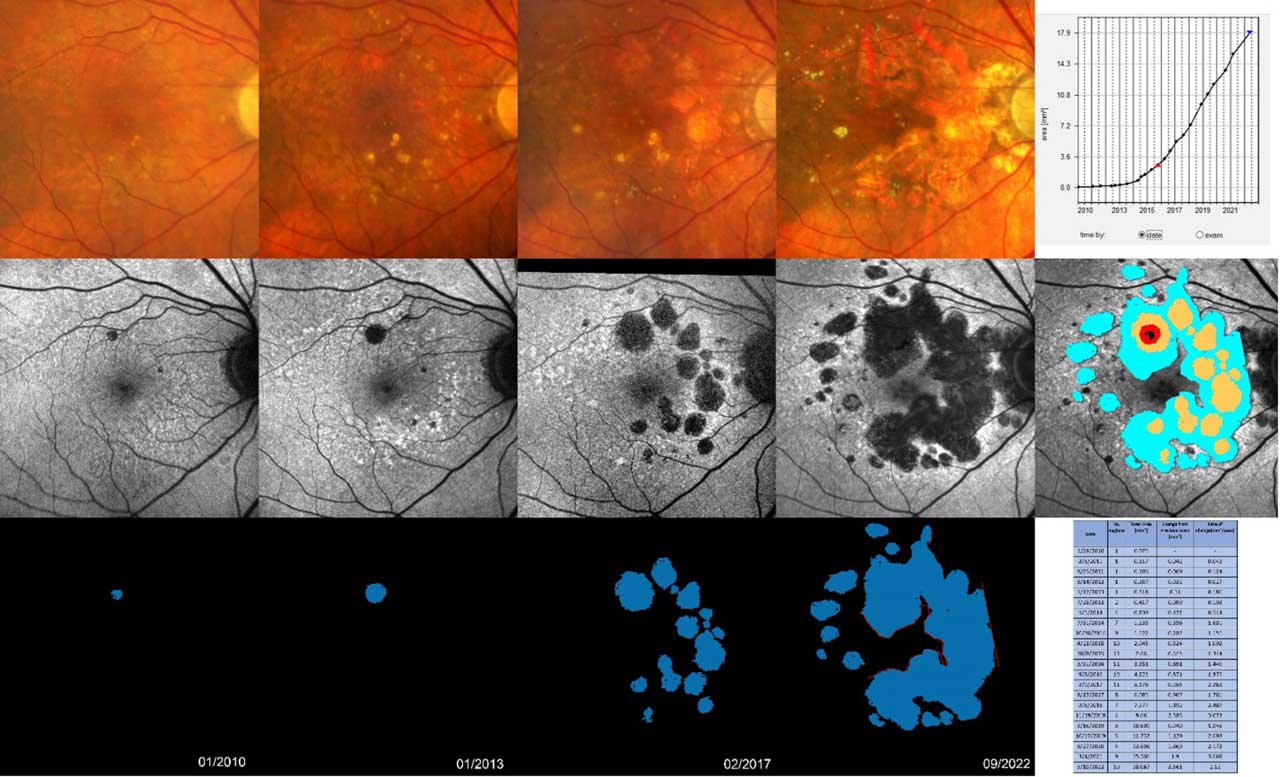
Optical Coherence Tomography
Optical coherence tomography (OCT) provides in vivo cross-sectional and en face visualization of the retina and RPE. Spectral-domain OCT (SD-OCT) is widely available and validated to assess and quantify phenotypes of AMD. Volume scans (SD-OCT or swept-source OCT) integrate a dense raster pattern of line-scans to display a representative image of macula topography. Spacing between line-scans can be modified to provide more or less detail within the image. It is important to select spacing sufficiently small to detect small areas of atrophy or subtle intraretinal or subretinal fluid. The level of detail desired must be balanced against time constraints, because denser scans require longer acquisition times. Although SD-OCT settings will be determined to some extent by the clinical question, SD-OCT should cover an area of at least 6 mm x 6 mm with distances between single line-scans no greater than 120 mm. An OCT should be completed at each visit and directly compared to prior images to monitor for progression of dry AMD/GA and for conversion to exudative AMD.2
Angiography
Fluorescein angiography (FA) has limited utility for monitoring dry AMD. A baseline FA can be considered to rule out the presence of MNV. Outside of this purpose, FA is not necessary for routine management of dry AMD.2,8
Optical coherence tomography angiography (OCTA) detects blood flow using motion contrast (eg, Doppler). Unlike conventional dye-based angiography, OCTA is noninvasive and depth-resolved. It is primarily useful for visualizing MNV and can detect early, nonexudative MNV. As such, it does not have a role in regular monitoring of dry AMD. It can be used when there is a specific concern for MNV formation based on OCT imaging in patients being followed for dry AMD.2,9,10
Differential Diagnostics
Several distinct inherited retinal degenerations and toxic maculopathies can manifest at a similar age and exhibit shared characteristics with AMD.11 Clinicians should be mindful that the mere presence of well-defined macular atrophy lesions in individuals over the age of 55 does not automatically point to an AMD-related cause. Recent research suggests that common genetic variants linked to AMD rarely result in GA before the age of 70.12
For a precise differential diagnosis, retinal physicians, equipped with readily available multimodal imaging diagnostics in addition to clinical examination, should be vigilant for specific structural changes indicative of other retinal diseases. These include, but are not limited to, unusual FAF signal alterations around atrophic areas, such as flecks and focal regions with increased FAF, which are more characteristic of late-onset Stargardt disease (Figure 3). Drusen related to AMD typically do not lead to an elevated FAF signal. Therefore, when a clinician observes an increased signal precisely at the site of drusen or fails to detect drusen around atrophic regions, suspicion should arise regarding atrophy not attributed to AMD. Fundus autofluorescence imaging serves as a crucial tool in the process of differential diagnosis, because it can identify anomalous signal changes surrounding atrophic zones or even unusual distributions of atrophic areas themselves.
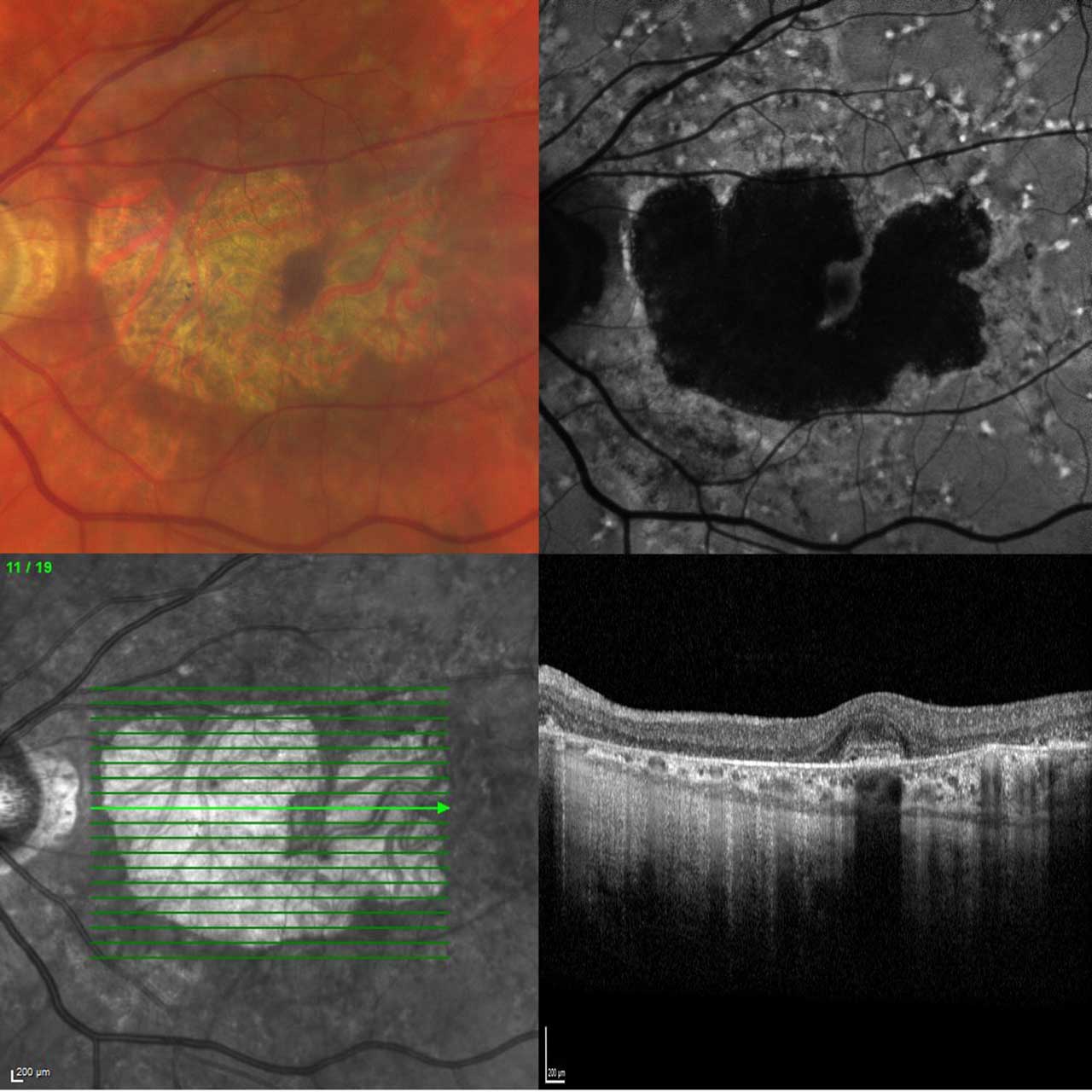
Going beyond imaging diagnostics, several key factors should be considered. These include a positive family history, particularly when an individual is diagnosed with macular atrophy at a young age. Additionally, the presence of atypical symptoms such as deafness, diabetes, skin changes, and a high cardiovascular risk profile, especially when combined with heavy smoking or prior use of medications like hydroxychloroquine or pentosan polysulfate sodium, should lead to a reconsideration of the AMD diagnosis. These factors can have significant implications for patient management, particularly when it comes to initiating disease-specific therapeutic strategies.
Conclusion
Current options for imaging patients with dry AMD include a baseline CFP, OCT, and FAF. In all subsequent follow-ups, OCT can be repeated using the same reference point to enable direct image comparisons. Employing the RegionFinder technology with FAF images can facilitate the assessment of GA progression. These tests are straightforward and noninvasive, streamlining the monitoring process of patients with dry AMD.
For patients undergoing GA treatment, clinicians may also consider obtaining a baseline OCT retinal nerve fiber layer scan to evaluate optic nerve health. This baseline scan can serve as a foundation for future monitoring, particularly in the context of serial high-volume injections and the potential risk of ischemic retinopathy. RP
Editor's note: This work was supported by National Institutes of Health Core Grant (EY014800), and an unrestricted grant from Research to Prevent Blindness to the Department of Ophthalmology and Visual Sciences, University of Utah.
This article is discussed on the Retina Podcast at www.retinapodcast.com.
References
Fleckenstein M, Keenan TDL, Guymer RH, et al. Age-related macular degeneration. Nat Rev Dis Primers. 2021;7(1):31. doi:10.1038/s41572-021-00265-2
Holz FG, Sadda SR, Staurenghi G, et al. Imaging protocols in clinical studies in advanced age-related macular degeneration: recommendations from classification of atrophy consensus meetings. Ophthalmology. 2017;124(4):464-478. doi:10.1016/j.ophtha.2016.12.002
Grunwald JE, Daniel E, Ying GS, et al. Photographic assessment of baseline fundus morphologic features in the Comparison of Age-Related Macular Degeneration Treatments Trials. Ophthalmology. 2012;119(8):1634-1641. doi:10.1016/j.ophtha.2012.02.013
Schmitz-Valckenberg S, Pfau M, Fleckenstein M, et al. Fundus autofluorescence imaging. Prog Retin Eye Res. 2021;81:100893. doi:10.1016/j.preteyeres.2020.100893
Wolf-Schnurrbusch UE, Wittwer VV, Ghanem R, et al. Blue-light versus green-light autofluorescence: lesion size of areas of geographic atrophy. Invest Ophthalmol Vis Sci. 2011;52(13):9497-9502. Published 2011 Dec 16. doi:10.1167/iovs.11-8346
Pfau M, Goerdt L, Schmitz-Valckenberg S, et al. Green-light autofluorescence versus combined blue-light autofluorescence and near-infrared reflectance imaging in geographic atrophy secondary to age-related macular degeneration. Invest Ophthalmol Vis Sci. 2017;58(6):BIO121-BIO130. doi: 10.1167/iovs.17-21764
Schmitz-Valckenberg S, Brinkmann CK, Alten F, et al. Semiautomated image processing method for identification and quantification of geographic atrophy in age-related macular degeneration. Invest Ophthalmol Vis Sci. 2011;52(10):7640-7646. doi:10.1167/iovs.11-7457
Vujosevic S, Vaclavik V, Bird AC, Leung I, Dandekar S, Peto T. Combined grading for choroidal neovascularisation: colour, fluorescein angiography and autofluorescence images. Graefes Arch Clin Exp Ophthalmol. 2007;245(10):1453-1460. doi:10.1007/s00417-007-0574-9
Müller PL, Pfau M, Schmitz-Valckenberg S, et al. Optical coherence tomography-angiography in geographic atrophy. Ophthalmologica. 2021;244(1):42-50. doi: 10.1159/000510727
Roisman L, Zhang Q, Wang RK, et al. Optical coherence tomography angiography of asymptomatic neovascularization in intermediate age-related macular degeneration. Ophthalmology. 2016;123(6):1309-1319. doi:10.1016/j.ophtha.2016.01.044
Saksens NT, Fleckenstein M, Schmitz-Valckenberg S, et al. Macular dystrophies mimicking age-related macular degeneration. Prog Retin Eye Res. 2014;39:23-57. doi:10.1016/j.preteyeres.2013.11.001
Schmitz-Valckenberg S, Fleckenstein M, Pappas C, Hageman J, Zouache MA, Hageman GS. Initial manifestation of complete retinal pigment epithelium and outer retinal atrophy (cRORA) in age-related macular degeneration: associations with genetic risk profile. Invest Ophthalmol Vis Sci. 2023; 64(8):918.