Optogenetics is a technique of genetic engineering that enables cells to express photoactive proteins whose activity can be modulated using light. Although the groundwork for optogenetics was laid decades ago in basic science, recent approaches aim to equip photoceptor alternative cells in the retina to work as a biological actuator by light-induced restoration of downstream vision signaling. This vision restoration approach is particularly appealing in retinal degenerations such as retinitis pigmentosa (RP) and others where there is relative preservation of the inner retina even in end-stage disease. This article discusses the fundamental principles of optogenetics, clinical relevance to retinal disease, and future considerations.
OPSINS
Opsins are near-universal photoreceptor molecules in living organisms and are at the front lines of phototransduction: the process of capturing a photon of light and transforming that photon into physiologic responses. Opsins are stimulated by light and propagate signals to effector targets through various downstream signaling pathways, some of which are complex and others relatively simple, depending on the type of opsin. Human native retinal photoceptors use opsins to capture light for phototransduction. Rod photoceptors express rhodopsin, while native cone photoreceptors express unique cone opsins. Retinal optogenetics relies on the targeted insertion of an optogene into a target retinal cell, commonly using an adeno-associated virus (AAV) vector usually delivered via an intravitreal injection. The various functional properties of different opsins allow for various photoactive capabilities and dictate the nature of intracellular signaling and cellular membrane potential changes.
OPTOGENETICS THERAPY FOR VISION RESTORATION
Optogenetics therapy for vision restoration is multifaceted. First is the engineering and delivery of the optogene to its target cell or cells. Adequate optogene expression then confers light-sensitivity to neurons (photoreceptors, ganglion cells, bipolar cells, horizontal cells, amacrine cells) in the retina. These cells’ primary native function, except for cones, is signal propagation rather than light detection.
Currently, retinal ganglion cells (RGCs) are a frequent target for therapy, because studies have shown good penetration of AAV vectors from the vitreous cavity to the inner retina,1 although some investigators have targeted bipolar and other retinal interneurons,2 as well as dormant cone photoreceptors. Depending on the opsin characteristics, there are specific wavelengths of light activation or intensity required to elicit a light-induced cellular physiologic response, thus some investigators have used specialized visual interface goggles to transmit light at the appropriate intensity or wavelength into the eye onto the retina and to perform image processing prior to retinal stimulation.3 After gene therapy treatment and adequate time for optogene expression, patient vision training is a vital component of the vision rehabilitation process, so that the patient can better learn how to function and leverage the unique characteristics of the restored visual perceptions.
OPTOGENETICS WITHIN THE RETINAL GENE THERAPY LANDSCAPE
Although classical gene therapy involves replacement of a defective disease-causing gene or gene product, optogenetics therapy is a gene mutation–independent approach. In diseases such as RP where there are greater than 70 known disease-causing mutated genes, with numerous pathogenic variants, the cost and manpower for the development of a safe and effective gene-specific therapy for each mutation is not practical. Nor would deploying a gene-specific therapy be suitable for patients with end-stage photoceptor degeneration, because the photoceptor population is too decimated to revive even with theoretical replacement of the defective disease-causing gene or gene product. Retinal optogenetics may have broad applicability for a variety of retinal degenerations but may be particularly useful in end-stage disease with widespread or complete photoreceptor loss. Nevertheless, retinal optogenetics and classical gene-specific therapy are not mutually exclusive, as there may also be a role for combined therapeutic approaches in the future to preserve native photoreceptors while improving retinal photosensitivity through optogenetics.
There are several ongoing in-human retinal optogenetics therapies in early phase clinical trials and prospective retinal optogene candidates in development (Table 1). The landscape is growing, with several relatively new retinal optogenetics-based gene therapy startups and interest from big pharma. This is demonstrated by the recent acquisition of 2 retinal optogenetic based companies, Vedere Bio and Arctos Medical, by Novartis. Another company, Ray Therapeutics, is pursing FDA IND for its optogenetic gene therapy for RP, Stargardt disease, and choroideremia, with aspirations to add geographic atrophy applications after successful preclinical testing.
COMPANY | TARGET | DEVICE NEEDED? | NEED FOR CHROMOPHORE SUPPLEMENTATION? | SENSITIVITY | SPECTRAL SENSITIVITY | KINETICS | INDICATION | STATUS | |
Allergan (formerly RetroSense) | Retinal ganglion cells (RGC) | Yes; high-intensity light activation goggles | No | Low | Channelrhodopsin-2; only works in blue light | Fast | Retinitis pigmentosa (RP) | Phase 1/2 (started 2016); no data yet released | |
GenSight Biologics | RGC | Yes; high-intensity laser scanning goggles | No | Low | ChrimsonR; only works in amber light (590 nm) | Fast | RP, dry AMD | Phase 1/2 (started 2018) | |
Bionic Sight (formerly AGTC) | RGC | Yes; high-intensity light activation googles | No | Low | ChronosFP (light-sensitive channel rhodopsin); only works in blue light | Fast | RP | Phase 1/2 (started 2020) | |
Novartis (formerly Vedere Bio) | RGC | Unknown | Yes | Very high | Medium wavelength cone opsin; only works in green light | Very slow | RP | Preclinical | |
Novartis (formerly Arctos) | Bipolar cells | Unknown | No | High | Opto-mGluR6; only works in blue light | Slow | RP, dry AMD | Preclinical | |
Nanoscope Therapeutics | Bipolar cells | None needed | No | High | Multicharacteristic (polychromatic) opsin; broad (visible light) | Fast | RP, dry AMD | Phase 2 ongoing | |
Ray Therapeutics | RGC | None needed | No | Very high | ChRown; broad (visible light) | Fast | RP, dry AMD, Stargardt, choroideremia | Preclinical |
CLINICAL TRIALS
Retinal optogenetic therapies are in early in-human clinical trials treating patients mostly with end-stage RP.4-7 The first peer-reviewed publication in 2021 of partial vision restoration in a blind patient with end-stage RP participating in the PIONEER trial was an important milestone in demonstrating proof of principal of optogenetic gene therapy for restoration of formed vision, not merely light perception.3 The study, sponsored by GenSight Biologics, used a microbial algae–derived channelrhodopsin, ChrimsonR, targeting RGCs in tandem with light-stimulating goggles worn by the patient to stimulate the genetically transduced RGCs with monochromatic light. More patients in this trial are experiencing similar levels of perceptions. The Bionic Sight optogenetic product also targets the RGC with a specific opsin and in tandem with visual interface system worn by the patient consisting of “retinal neural decoder” and light transducer to create patterns of light that augment the light stimulus to optimize the responses. Bionic Sight have reportedly treated several patients in a low-dose cohort with plans for a higher dose cohort to follow. Allergan’s early-phase trial began in 2015 using the optogene for channelrhodopsin-2. This study showed reassuring safety signals, but no data have yet been released on efficacy. Nanoscope uses an ambient light–detecting multicharacteristic (polychromatic) opsin targeting bipolar cells rather than RGCs and without a light-stimulating device or neuroprosthetic adjuvant. Theoretically, the polychromatic opsin has a broad wavelength activation spectrum. Nanoscope completed a preliminary safety trial conducted in India and reportedly completed enrollment of a phase 2b trial in the United States with a data readout to follow shortly. In all studies to date, the safety signals for the early phase clinical trials seem reassuring, with intraocular inflammation common but not serious.
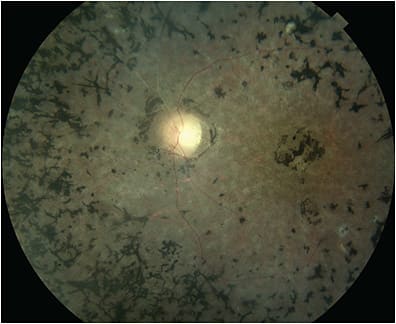
FUTURE OUTLOOK
Optogenetic gene-independent vision restoration is novel and has unique potential to impact the field of vision restoration. Although early in-human clinical trials indicate potential for formed vision restoration in blind patients, many approaches are currently under development to further improve the quality of optogenetically formed vision. Some retinal processing is lost when bypassing the retinal interneuron system by utilizing the downstream RGCs as an optogenetic actuator. As such, first-order retinal interneurons are attractive optogene target candidates because they link the initial light signal into the inner retinal circuitry and thereby maximize the receptive-field diversity within the RGC population. Other hardware engineering advances to the visual interface may augment the light stimulus to optimize excitation of the genetically modified retina. It is also well recognized that patient visual rehabilitation and vision training is imperative for the success of patients’ learning how to leverage the capabilities of the visual perceptions. Further efforts on optimizing posttreatment vision training may further enhance visual capabilities and streamline the visual rehabilitation process.
Ongoing work to observe cells expressing the photoactive protein in vivo after gene therapy transduction could prove particularly useful to monitor vector transfection and to individually tailor the size and location of the light beam projected by the light-stimulating devices to better adapt to the topography of retinal nerve cells. Further work is being done on opsin optimization to reduce the intensity of light necessary for activation and to better tailor activation.8 Currently, the focus of retinal optogenetics is the targeting of inner retinal neuronal cells, those without native direct photoactive capabilities, although some researchers have targeted cone photoreceptors.9,10 There may also be a role for combined vision restoration approaches with treatments that preserve native photoreceptors in combination with improving retinal photosensitivity through optogenetics. As the retinal optogenetics space emerges from its infancy, comparing to retinal prosthetic devices and direct cortical stimulation, the integration of this new therapeutic strategy in the range of potential vision restoration strategies will be important. RP
REFERENCES
- Gauvain G, Akolkar H, Chaffiol A, et al. Optogenetic therapy: high spatiotemporal resolution and pattern discrimination compatible with vision restoration in non-human primates. Commun Biol. 2021;4(1):125. doi:10.1038/s42003-020-01594-w
- Kralik J, van Wyk M, Stocker N, et al. Bipolar cell targeted optogenetic gene therapy restores parallel retinal signaling and high-level vision in the degenerated retina. Commun Biol. 2022;5:1116. https://doi.org/10.1038/s42003-022-04016-1
- Sahel JA, Boulanger-Scemama E, Pagot C, et al. Partial recovery of visual function in a blind patient after optogenetic therapy. Nat Med. 2021;27(7):1223-1229. doi:10.1038/s41591-021-01351-4
- Dose-escalation study to evaluate the safety and tolerability of GS030 in subjects with retinitis pigmentosa (PIONEER). Clinicaltrials.gov identifier: NCT03326336. Updated July 26, 2022. Accessed February 7, 2023. https://clinicaltrials.gov/ct2/show/NCT03326336
- RST-001 phase I/II trial for advanced retinitis pigmentosa. Clinicaltrials.gov identifier: NCT02556736. Updated October 6, 2022. Accessed February 7, 2023. https://clinicaltrials.gov/ct2/show/NCT02556736
- BS01 in patients with retinitis pigmentosa. Clinicaltrials.gov identifier: NCT04278131. Updated July 26, 2022. Accessed February 7, 2023. https://clinicaltrials.gov/ct2/show/NCT04278131
- Efficacy and safety of vMCO-010 optogenetic therapy in adults with retinitis pigmentosa [RESTORE] (RESTORE). Clinicaltrials.gov identifier: NCT04945772. Updated July 26, 2022. Accessed February 7, 2023. https://clinicaltrials.gov/ct2/show/NCT04945772
- Berry MH, Holt A, Broichhagen J, Donthamsetti P, Flannery JG, Isacoff EY. Photopharmacology for vision restoration. Curr Opin Pharmacol. 2022;65:102259. doi: 10.1016/j.coph.2022.102259.
- Busskamp V, Duebel J, Balya D, et al. Genetic reactivation of cone photoreceptors restores visual responses in retinitis pigmentosa. Science. 2010;329(5990):413-417. doi: 10.1126/science.1190897.
- Bertin S, Brazhnikova E, Jaillard C, Sahel JA, Dalkara D. AAV-mediated gene delivery to foveal cones. Methods Mol Biol. 2020;2173:101-112. doi:10.1007/978-1-0716-0755-8_6