The origins of vitrectomy surgery can be traced back to the 1960s when Dr. David Kasner discovered that the vitreous could be safely removed from the eye using cellulose sponges and scissors, a technique he termed “open-sky vitrectomy.”1 This work piqued the interest of Dr. Robert Machemer, who in the 1970s pioneered the first single-port closed pars plana approach with his vitreous infusion suction cutter (VISC) system.
Since then, significant advancements in vitrectomy technology have improved the safety and efficacy of the procedure. This article will review some of the technological innovations in vitrectomy, focusing on gauge size, cut speeds, adaptive fluidics, endoillumination, and advanced instrumentation.
SMALLER GAUGE INSTRUMENTS
One of the major innovations in vitrectomy technology has been the development of smaller, more precise surgical tools. After the introduction of the first 3-port vitrectomy system in 1975, the instrumentation size has decreased from 20 gauge to what is known today as microincision vitrectomy systems (MIVS).2 These systems promote self-sealing sutureless sclerotomies2,3 and include 23-gauge, 25-gauge, and 27-gauge instruments. (Figure 1)
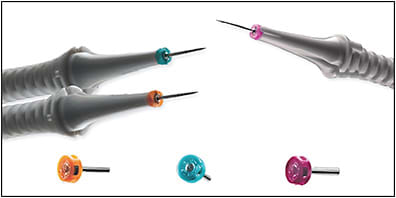
The use of smaller instruments has numerous benefits such as quicker recovery, less scarring, greater comfort, shorter surgery times, and less astigmatism. Additionally, these small-gauge instruments enable surgeons to perform complex maneuvers in challenging surgeries.
Small-gauge vitrectomy presents many benefits, but the reduction in sclerotomy and instrument size reveals balancing drawbacks as well. As demonstrated by Poiseuille’s law, the flow rate is directly proportional to the radius raised to the fourth power.4 Therefore, as the radius of the probe reduces, the aspiration flow rate also decreases. To counter this problem, manufacturers have devised various solutions, which will be discussed below.
HIGHER CUT SPEEDS
Ideal tissue cutting should produce no vitreoretinal traction. This can be achieved by using higher cutting rates, which reduces pulsatile fluid flow, an important source of vitreoretinal traction (Figure 2).5,6 Duty cycle, which refers to the amount of time the port probe is open during a cycle, is a crucial factor in determining the efficiency and safety of cutting.6
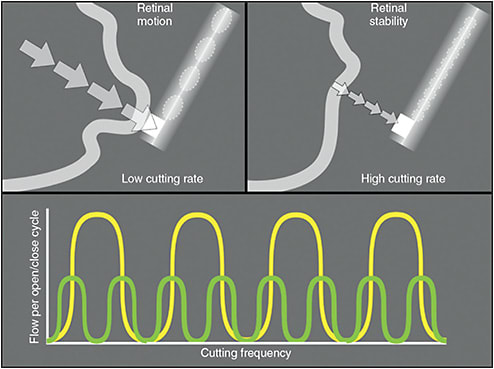
Before 2008, cutters were actuated using a spring to open the guillotine and were known as single-actuation cutters (Figure 3).7 However, at higher cutting rates, these cutters had reduced duty cycle and flow rates.7,8 To overcome this limitation, Alcon developed the Ultravit probe, which used a dual-actuation mode system that relied on air pressure to both open and close the guillotine (Figure 4).9 In this system, the probe is open for longer intervals between cuts, maintaining an effective aspiration flow rate and duty cycle with cut rates up to 5,000 cpm. Later, the Constellation Advanced Ultravit cutter was developed, achieving even higher cut rates of up to 10,000 cpm.
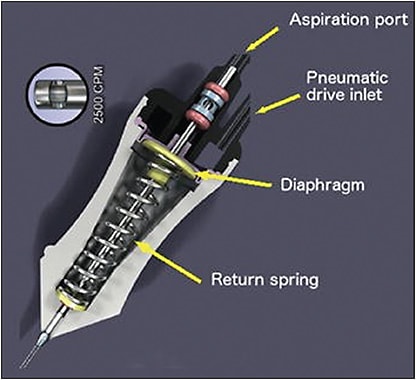
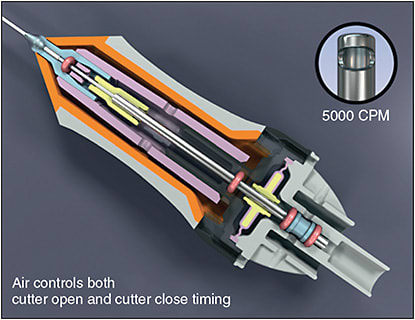
In 2014, DORC introduced the TDC probe with a 2-dimensional cutting system that doubled the cut rate up to 16,000 cpm while maintaining a duty cycle above 90% and stable aspiration flow rate independent of the cut rate (Figure 5).10 Other surgical systems, such as Bausch + Lomb’s Bi-Blade cutter, Alcon’s Hypervit cutter, and DORC’s TDC Veloce cutter, have adopted this dual-blade design, offering cutting rates up to 20,000 cpm and higher aspiration flow rates in multiple gauges.
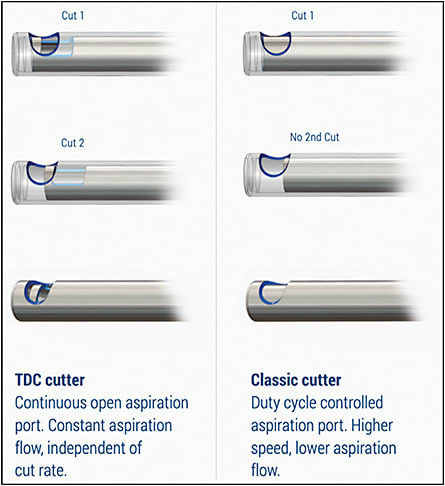
High-speed cutting technology has revolutionized vitrectomy surgery by making surgeries faster, safer, and more efficient. Patients benefit from reduced surgical times, less tissue damage, and faster recovery times, while surgeons can perform more complex surgeries with greater precision and accuracy.
ADAPTIVE FLUIDICS
Maintenance of stable fluid dynamics during vitrectomy surgery has been a focus of research and development in new vitrectomy systems. The goal is to efficiently remove vitreous while minimizing turbulence. To achieve this, the industry has developed intelligent systems that can modulate fluid flow while minimizing changes in intraocular pressure, known as adaptive fluidics.
Until very recently, 2 types of pumping and cartridge systems existed: Venturi and peristaltic (Figure 6). In the Venturi system, the vacuum is created by the flow of compressed air or nitrogen over an opening.11 These lines and tubes are contained within a sealed plastic unit called a cassette. In these systems, the vacuum is controlled by a foot pedal and the resulting flow depends on the viscosity, size of aspiration path, cut rate, and achieved vacuum value. Both Alcon’s Constellation and Bausch + Lomb’s Stellaris platforms use this type of pumping system.
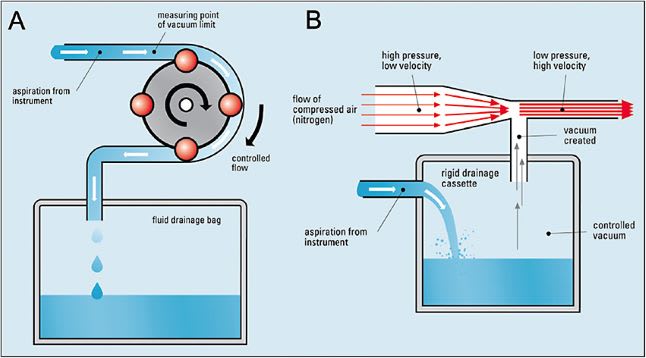
In contrast, the peristaltic pump uses rollers to compress the outflow tubing, creating flow. The rotation speed of the rollers controls the flow, and vacuum is generated only upon occlusion of the aspiration line. The flow is controlled by the foot pedal and the vacuum adjusts itself to the lowest level required to maintain that flow.11
Of equal importance is the concept of intraocular pressure control. Early vitrectomy systems used gravity to move fluid from a bottle into the eye and the position of the bottle determined the intraocular pressure.6 In 1997, Alcon introduced the Accurus system, which used vented gas forced infusion to pressurize the balanced salt solution bottle, giving the surgeon more control over the pressure. However, given the lack of integration between the infusion and extraction components, pressure control was still imprecise, with fluctuations and instability.12 To compensate for this and avoid hypotony during surgery, most surgeons operated at higher pressures, which increased the risk of corneal edema, retinal incarceration, and decreased ocular perfusion.12
In 2009, the Constellation Vision System incorporated an IOP compensation feature that used flow sensors to maintain a desired pressure. This allowed surgeons to work at lower pressures while preventing “outrunning” the infusion.13 The system also incorporated smaller chambers in the cassette for faster vacuum buildup.
In 2012, DORC introduced the Eva surgical platform with VacuFlow valve timing intelligence pump system, which uses computer-controlled operating pistons and closure valves to adjust fluid flow based on real-time data from the surgical environment (Figure 7).14 This technology combines the functionality of Venturi and peristaltic pumps, allowing for smooth transitions between vacuum and flow modes to optimize fluidics for each surgical demand.
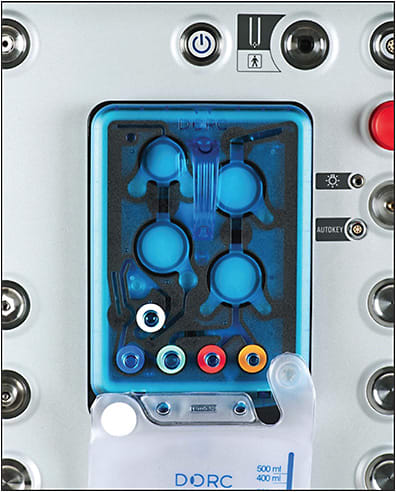
ILLUMINATION
There have been numerous advances in endoillumination technology since the 20-gauge era. In the past, halogen or metal halide bulbs were used as the illuminating light source.15 However, as MIVS was introduced, these light sources only provided 50% or less of the brightness obtained in 20-gauge systems. Another important concern was the issue of phototoxicity, which had been very well described since the 1980s. To address this, all available illumination systems incorporate lower wavelength filters to block potentially harmful ultraviolet and blue light.16,17 Numerous advances have been made over the years to improve not only the safety and brightness but also the durability and versatility of these instruments.
One such advancement was the introduction of the Photon illumination device by Synergetics in 2004, which used a xenon bulb and delivered 8 times more light at safer levels.18 Later, they released their Photon II Ex model, which used a mercury vapor bulb and provided up to 2 times more illumination.15 Currently, the Constellation system uses a xenon bulb and the Stellaris PC (Bausch + Lomb) comes with both, a xenon and a mercury vapor lamp. Lastly, the light-emitting diode light source was introduced on the Eva platform and the Versavit (Synergetics).19
These newer light sources have benefits such as more uniform illumination, lower heat emission, longer lifespan, and better color rendering. Light filters are also used to improve safety and enhance tissue visualization. Manufacturers also offer various options for focal, midfield, and widefield illumination (Figure 8).
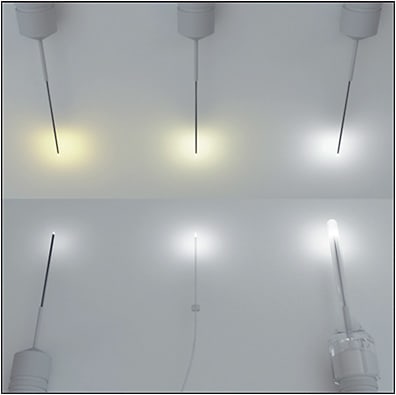
ADVANCED INSTRUMENTATION
In 1974, Machemer introduced the use of a bent needle for membrane peeling.20 Subsequently, several instruments were developed to facilitate manipulation of intraocular tissues. Conor O’Malley designed the pic, which had a rounded, blunt end to avoid retinal penetration, followed by Steve Charles’s end-grasping forceps and Yasuo Tano’s diamond-dusted membrane scraper.21 Although these instruments remain in wide use, they have undergone numerous technological advancements. Most are now available in small gauges (23, 25, 27) and as disposable, single-use instruments, eliminating handling and sterilization costs, reducing the risk of toxic anterior segment syndrome–like inflammation, and providing greater precision and sharpness than reusable instruments.11
Now, all major manufacturers offer a wide variety of instruments, each with its own unique designs and features. Alcon’s Grieshaber line has some of the more commonly used forceps, such as the internal limiting membrane forceps, serrated forceps, and end-grasping forceps (Figure 9). Scissors are also frequently used during segmentation and delamination techniques to cut tractional bands close to the retinal surface. These come with the blades aligned either horizontally or vertically to the retina. Membrane scrapers are often used to lift an edge of the membrane prior to peeling. Some examples include Alcon’s nitinol flex loop, Bausch + Lomb’s adjustable tano with pick, and DORC’s Extendible Diamond Dusted Sweeper.
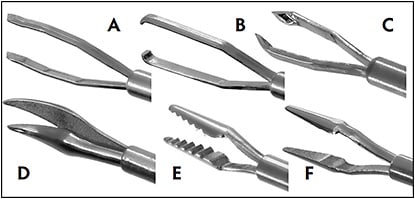
Lastly, many of these instruments now feature illumination, reducing the need for chandelier placement in bimanual surgery. These instruments have become critical tools for performing delicate maneuvers during complex surgeries.
CONCLUSION
The field of vitreoretinal surgery is constantly evolving and improving. The development of advanced MIVS equipment and better visualization techniques have made once daunting procedures more commonplace. These advancements have resulted in shorter surgery times, smaller incisions, and higher levels of surgical safety, ultimately leading to improved patient outcomes. The technology underlying modern vitrectomy surgery has progressed in leaps and bounds in recent years, and thanks to the contributions of many innovators, vitreoretinal surgeons have access to a variety of tools and techniques unprecedented in their precision and efficacy. As technology continues to progress, we can expect even more innovations in vitrectomy surgery that will further enhance the quality of life and outcomes for patients. RP
REFERENCES
- Blodi CF. David Kasner, MD, and the road to pars plana vitrectomy. Ophthalmol Eye Dis. 2016;8(Suppl 1):1-4. doi:10.4137/OED.S40424
- Fujii GY, De Juan E Jr, Humayun MS, et al. A new 25-gauge instrument system for transconjunctival sutureless vitrectomy surgery [published correction appears in Ophthalmology. 2003;110(1):9.]. Ophthalmology. 2002;109(10):1807-1813. doi:10.1016/s0161-6420(02)01179-x
- Eckardt C. Transconjunctival sutureless 23-gauge vitrectomy. Retina. 2005;25(2):208-211. doi:10.1097/00006982-200502000-00015
- Magalhães O Jr, Maia M, Rodrigues EB, et al. Perspective on fluid and solid dynamics in different pars plana vitrectomy systems. Am J Ophthalmol. 2011;151(3):401-5.e1. doi:10.1016/j.ajo.2010.11.009
- Teixeira A, Chong L, Matsuoka N, et al. An experimental protocol of the model to quantify traction applied to the retina by vitreous cutters. Invest Ophthalmol Vis Sci. 2010;51(8):4181-4186. doi:10.1167/iovs.09-4852
- Sadda SVR, Wilkinson CP, Wiedemann P, et al. Principles and techniques of vitreoretinal surgery. In: Ryan’s Retina. 7th ed. Elsevier; 2023:2040-2056.
- Magalhaes O Jr, Chong L, DeBoer C, et al. Guillotine performance: duty cycle analysis of vitrectomy systems. Retin Cases Brief Rep. 2009;3(1):64-67. doi:10.1097/ICB.0b013e31815657a7
- Hubschman JP, Gupta A, Bourla DH, Culjat M, Yu F, Schwartz SD. 20-, 23-, and 25-gauge vitreous cutters: performance and characteristics evaluation. Retina. 2008;28(2):249-257. doi:10.1097/IAE.0b013e31815ec2b3
- Abulon DJ. Vitreous flow rates through dual pneumatic cutters: effects of duty cycle and cut rate. Clin Ophthalmol. 2015;9:253-261. doi:10.2147/OPTH.S71387
- Oravecz R, Uthoff D, Schrage N, Dutescu RM. Comparison of modern high-speed vitrectomy systems and the advantages of using dual-bladed probes. Int J Retina Vitreous. 2021;7(1):8. doi:10.1186/s40942-020-00277-2
- Charles S. Fluidics and cutter dynamics. Dev Ophthalmol. 2014;54:31-37. doi:10.1159/000360446
- Moorhead LC, Gardner TW, Lambert HM, et al. Dynamic intraocular pressure measurements during vitrectomy. Arch Ophthalmol. 2005;123(11):1514-1523. doi:10.1001/archopht.123.11.1514
- Sugiura Y, Okamoto F, Okamoto Y, Hiraoka T, Oshika T. Intraocular pressure fluctuation during microincision vitrectomy with Constellation vision system. Am J Ophthalmol. 2013;156(5):941-947.e1. doi:10.1016/j.ajo.2013.06.016
- Lussenburg K, Scali M, Sakes A, Breedveld P. Additive manufacturing of a miniature functional trocar for eye surgery. Front Med Technol. 2022;4:842958. doi:10.3389/fmedt.2022.842958
- Sakaguchi H, Oshima Y. Considering the illumination choices in vitreoretinal surgery. Retin Phys. 2012;9:26-31.
- Matsumoto CS, Shibuya M, Makita J, et al. Heads-up 3D surgery under low light intensity conditions: new high-sensitivity hd camera for ophthalmological microscopes. J Ophthalmol. 2019;2019:5013463. doi:10.1155/2019/5013463
- Oshima Y, Chow DR, Awh CC, Sakaguchi H, Tano Y. Novel mercury vapor illuminator combined with a 27/29-gauge chandelier light fiber for vitreous surgery. Retina. 2008;28(1):171-173. doi:10.1097/IAE.0b013e31814fb0d3
- Daily S. Synergetics USA Inc. Retina Today. May/June 2011. Accessed June 12, 2023. https://retinatoday.com/articles/2011-may-june/retina-on-wall-street-synergetics-usa-inc
- de Oliveira PR, Berger AR, Chow DR. Vitreoretinal instruments: vitrectomy cutters, endoillumination and wide-angle viewing systems. Int J Retina Vitreous. 2016;2:28. doi:10.1186/s40942-016-0052-9
- Machemer R. A new concept for vitreous surgery. 7. Two instrument techniques in pars plana vitrectomy. Arch Ophthalmol. 1974;92(5):407-412. doi:10.1001/archopht.1974.01010010419009
- Charles S. Vitrectomy techniques for complex retinal detachments. Taiwan J Ophthalmol. 2012;2(3):81-84. doi:10.1016/j.tjo.2012.06.002