Optical coherence tomography (OCT) has revolutionized ophthalmic care. It is integral to the preoperative and postoperative evaluation of patients. Yet, intraoperatively, most surgeons are without this tool and are wholly reliant on the surgical microscope’s illuminated en face view for surgical maneuvers and decision making.
Intraoperative OCT (iOCT) serves to provide surgeons with the same cross-sectional and tomographic data that proves so critical in clinic.1 Since its advent in 2005, there have been notable advances in iOCT technology, ranging from early stand-alone time-domain systems to modern microscope-integrated systems equipped with heads-up diplays.2 The technology enables surgeons in real time to discern between retinal structures and evaluate for the presence or absence of subtle but key anatomic features, which can guide surgical decision-making. In fact, 2 large prospective clinical trials, PIONEER and DISCOVER, demonstrated that iOCT may alter intraoperative decision-making in a significant percentage of anterior-segment and posterior-segment surgeries.3,4 This article will discuss the evolution over time, various applications, and role in modern vitreoretinal surgery of iOCT.
HISTORY
The first use of iOCT was in 2005, when a time-domain OCT was used for real-time visualization of anterior-segment structures during lamellar keratoplasty and trabeculectomy.5 This initial experimental system worked by coupling a commercially available optical coherence tomograph to the front lens of a standard surgical microscope using a beam splitter.5
In 2007, the first portable OCT probe was developed for use intraoperatively using standard domain technology.6 These handheld systems allowed for high-quality 2-dimensional OCT imaging, but the handheld probes came with several limitations. Firstly, they were separate from the surgical microscope, and thus surgery had to be paused for image acquisition. Image reproducibility and optical aiming were limited. Motion artifact was common due to difficulty with imaging stabilization. Moreover, it was often difficult to maintain a sterile surgical field.
Thus, mounted systems were developed to provide improved stability. External mounts increased the system footprint and required additional operating room space. Microscope-mounted systems fastened the OCT probe directly to the microscope body. This not only improved stability but also allowed the foot pedal to control probe location and improve image reproducibility.4,7 However, all these systems required pausing surgery for image acquisition.4
The integration of OCT and microscope optics was the next major advancement in iOCT systems, providing real-time OCT-based visualization of instrument-tissue interaction and immediate imaging with surgeon feedback.8 Z-tracking and focus controls were incorporated into the device to improve the stability and quality of the captured images.2,9 There are currently 3 FDA-approved systems available: Haag-Streit iOCT, Zeiss Rescan 700, and Leica EnFocus, all of which employ spectral-domain OCT technology. Furthermore, newer systems are integrating swept-source technology and 3D heads-up display for improved functionality.
VITREORETINAL APPLICATIONS
Macular Surgery
The utility of iOCT has been extensively described in macular surgery. Intraoperative OCT can reveal residual membranes or completion of the membrane peel (Figure 1), potentially avoiding incomplete treatment or unnecessary surgical maneuvers.10 Studies have shown that iOCT can identify occult residual membranes in 12% of cases and are useful in confirming a completed membrane peel in 9% of cases.4 The DISCOVER trial showed a discrepancy between what surgeons perceived and what was actually present during the surgery. In 40% of cases where the surgeon believed there were residual membranes, iOCT showed complete removal of the membrane.3 Other studies have demonstrated that iOCT-assisted macular hole surgery achieved a single-surgery success rate of 97.6%, which was comparable to outcomes without iOCT.10-12
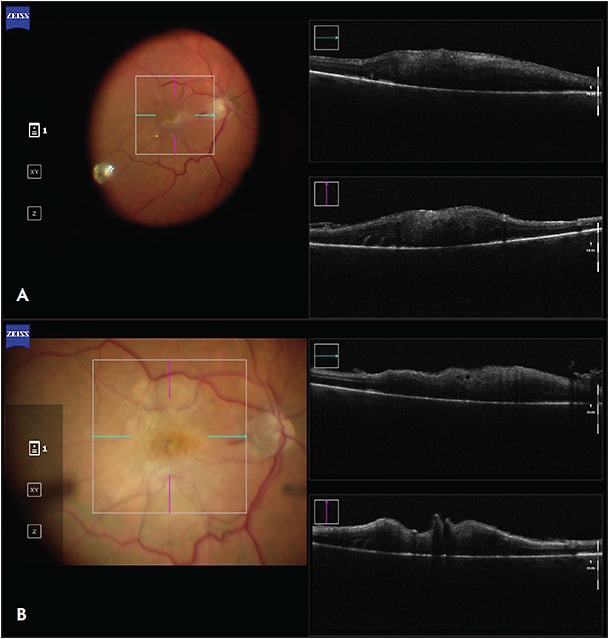
Furthermore, iOCT reduces reliance on intraocular dyes for peeling epiretinal membranes, potentially saving time, cost, and resources.13-15 According to 1 study, iOCT-guided membrane peeling was feasible in 40% of cases without the need for surgical staining agents.16 Intraoperative OCT has other benefits as well, such as determining the presence or absence of macular holes or evaluating for vitreous schisis.
Retinal Detachment
Intraoperative OCT during retinal detachment repair has proven to have many advantages, such as detecting residual subretinal fluid following perfluorocarbon liquid placement, identifying retinal breaks, and evaluating for proliferative vitreoretinopathy membranes. The DISCOVER trial revealed that, in RD repair, iOCT provided valuable feedback to surgeons in 36% of cases and altered surgical decision-making in 12% of cases.17 Intraoperative OCT provided valuable insight in complex redetachment cases compared to primary cases (50% vs 22%, P<.01).17
Proliferative Diabetic Retinopathy
Intraoperative OCT can be particularly insightful in cases with nonclearing vitreous hemorrhage where OCT cannot be used preoperatively (Figure 2).18,19 In these cases, media opacity can hinder preoperative surgical planning, but iOCT can identify surgical dissection planes intraoperatively.18 Intraoperative OCT has also been used to visualize cannula placement during viscodissection, to identify peripheral or macular holes/breaks, and to guide the selection of tamponade agents.18 A review of the DISCOVER study found that in surgery for proliferative diabetic retinopathy, surgeons considered iOCT to be valuable in 50.6% of cases and it influenced surgical decision-making in 26% of cases.18
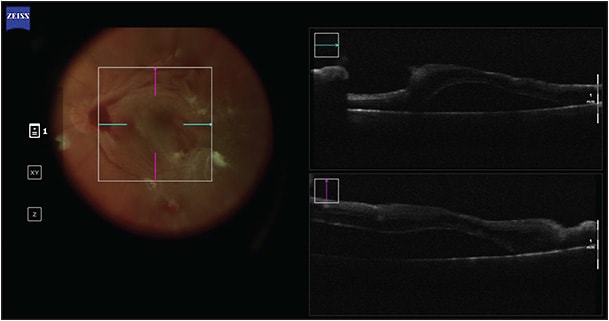
Uveitis
In uveitis-related vitreoretinal surgery, iOCT serves several roles. It can provide valuable feedback during complex uveitic membrane peels.20 Intraoperative OCT can confirm the integrity of scleral wound closure during Retisert fluocinolone acetonide implant placement (Bausch + Lomb) in 84.6% of cases.20 Additionally, it is useful in 81.2% of chorioretinal biopsies by guiding biopsy site selection and ascertaining whether there is sufficient volume of subretinal material to enable a successful biopsy.20,21
Subretinal and Submacular Surgery
Subretinal gene therapy delivery risks complications such as hemorrhage, cannula tip obstruction, retinal pigment epithelium damage, or suprachoroidal delivery if the needle penetrates too deeply, and intraretinal hydration or retinoschisis if too shallowly.22 Several attempts to create a subretinal bleb can result in localized neurosensory retinal trauma and retinotomy enlargement. Intraoperative OCT can aid by evaluating for proper needle depth and guide bleb location by avoiding areas with high-risk features such as retinal atrophy or retinoschisis.22,23 Future iOCT systems may quantify bleb volume to more accurately dose subretinal therapies.24 Similarly, iOCT has also been used to administer subretinal injections of tissue plasminogen activator for submacular hemorrhage.25
LIMITATIONS AND CHALLENGES
Intraoperative OCT has shown promise in enhancing surgical outcomes in a range of vitreoretinal surgeries. However, there are several limitations to its use that warrant consideration. The light-scattering and shadowing effects of metal present a challenge for current iOCT systems when using metallic surgical instruments.1 Image acquisition can be affected by patient movement, eye movements, or instrument motion, leading to motion artifacts, image distortion, and reduced image quality. Current iOCT systems face difficulties in capturing images of the peripheral retina. Also, adoption is limited by cost, availability, and the fact that many ophthalmic surgeons simply do not perceive it as an essential tool for their routine clinical practice.26
FUTURE DIRECTIONS
As iOCT continues to gain traction, research and development efforts are focused on advancing its capabilities and expanding its applications. Ehlers et al in 2017 proposed the development of several OCT-compatible surgical instruments designed to facilitate real-time, image-guided surgeries and instrument stabilization.27
Recent advances include development of a 3D iOCT digital surgical system, which purports to improve surgical precision and enrich surgical education and training.28,29 Additionally, iOCT could be integrated into the Clarity visualization system developed by Beyeonics Surgical, whereby surgeons wear a head-mounted display that uses augmented reality to generate an image.29
Duke University has developed a prototype of iOCT technology that uses swept-source and 4-dimensional iOCT to provide real-time volumetric imaging. This latest technology allows for better visualization of tissue deformation and instrument motion, reducing the need for constant object tracking.30,31
Ongoing technological developments are investigating OCT-guided robotic systems.32 Cereda et al examined the utility of an instrument-integrated OCT-based distance sensor in vitreoretinal surgery, assessing its potential to improve accuracy and efficiency during surgical procedures.33
CONCLUSION
Over the past decade, the capabilities of iOCT have advanced significantly. There is mounting evidence that iOCT can guide surgical maneuvers and intraoperative decision making in various cases. It may reduce unnecessary surgical steps, shorten surgical time, and lead to improved and safer outcomes. While further research is needed to continue improving iOCT applications and analyzing its long-term impacts, ongoing advances in iOCT technology are likely to lead to more widespread acceptance and usage among vitreoretinal surgeons. RP
REFERENCES
- Ehlers JP, Tao YK, Farsiu S, Maldonado R, Izatt JA, Toth CA. Integration of a spectral domain optical coherence tomography system into a surgical microscope for intraoperative imaging. Invest Ophthalmol Vis Sci. 2011;52(6):3153-3159. doi:10.1167/iovs.10-6720
- Ehlers JP. Intraoperative optical coherence tomography: past, present, and future. Eye (Lond). 2016;30(2):193-201. doi:10.1038/EYE.2015.255
- Ehlers JP, Modi YS, Pecen PE, et al. The DISCOVER study 3-year results: feasibility and usefulness of microscope-integrated intraoperative OCT during ophthalmic surgery. Ophthalmology. 2018;125(7):1014-1027. doi:10.1016/J.OPHTHA.2017.12.037
- Ehlers JP, Dupps WJ, Kaiser PK, et al. The Prospective Intraoperative and Perioperative Ophthalmic ImagiNg with Optical CoherEncE TomogRaphy (PIONEER) study: 2-year results. Am J Ophthalmol. 2014;158(5):999-1007.e1. doi:10.1016/J.AJO.2014.07.034
- Geerling G, Müller M, Winter C, et al. Intraoperative 2-dimensional optical coherence tomography as a new tool for anterior segment surgery. Arch Ophthalmol. 2005;123(2):253-257. doi:10.1001/ARCHOPHT.123.2.253
- Dayani PN, Maldonado R, Farsiu S, Toth CA. Intraoperative use of handheld spectral domain optical coherence tomography imaging in macular surgery. Retina. 2009;29(10):1457-1468. doi:10.1097/IAE.0B013E3181B266BC
- Ray R, Baraano DE, Fortun JA, et al. Intraoperative microscope-mounted spectral domain optical coherence tomography for evaluation of retinal anatomy during macular surgery. Ophthalmology. 2011;118(11):2212-2217. doi:10.1016/J.OPHTHA.2011.04.012
- Tao YK, Ehlers JP, Toth CA, Izatt JA. Intraoperative spectral domain optical coherence tomography for vitreoretinal surgery. Opt Lett. 2010;35(20):3315. doi:10.1364/OL.35.003315
- Pfau M, Michels S, Binder S, Becker MD. Clinical experience with the first commercially available intraoperative optical coherence tomography system. Ophthalmic Surg Lasers Imaging Retina. 2015;46(10):1001-1008. doi:10.3928/23258160-20151027-03
- Yee P, Sevgi DD, Abraham J, et al. iOCT-assisted macular hole surgery: outcomes and utility from the DISCOVER study. Br J Ophthalmol. 2021;105(3):403-409. doi:10.1136/BJOPHTHALMOL-2020-316045
- Jaycock PD, Bunce C, Xing W, et al. Outcomes of macular hole surgery: implications for surgical management and clinical governance. Eye (Lond). 2005;19(8):879-884. doi:10.1038/SJ.EYE.6701679
- Bae K, Kang SW, Kim JH, Kim SJ, Kim JM, Yoon JM. Extent of internal limiting membrane peeling and its impact on macular hole surgery outcomes: a randomized trial. Am J Ophthalmol. 2016;169:179-188. doi:10.1016/J.AJO.2016.06.041
- Ehlers JP, Khan M, Petkovsek D, et al. Outcomes of intraoperative OCT-assisted epiretinal membrane surgery from the PIONEER study. Ophthalmol Retina. 2018;2(4):263. doi:10.1016/J.ORET.2017.05.006
- Lorusso M, Micelli Ferrari L, Gisotti EN, et al. Success of iOCT in surgical management of ERM peeling. Eur J Ophthalmol. 2022;32(5):3116-3120. doi:10.1177/11206721221085383
- Rodrigues EB, Meyer CH, Mennel S, Farah ME. Mechanisms of intravitreal toxicity of indocyanine green dye: implications for chromovitrectomy. Retina. 2007;27(7):958-970. doi:10.1097/01.IAE.0000253051.01194.AB
- Falkner-Radler CI, Glittenberg C, Gabriel M, Binder S. Intrasurgical microscope-integrated spectral domain optical coherence tomography-assisted membrane peeling. Retina. 2015;35(10):2100-2106. doi:10.1097/IAE.0000000000000596
- Abraham JR, Srivastava SK, K Le T, et al. Intraoperative OCT-assisted retinal detachment repair in the DISCOVER study: impact and outcomes. Ophthalmol Retina. 2020;4(4):378. doi:10.1016/J.ORET.2019.11.002
- Khan M, Srivastava SK, Reese JL, Shwani Z, Ehlers JP. Intraoperative OCT-assisted surgery for proliferative diabetic retinopathy in the DISCOVER study. Ophthalmol Retina. 2018;2(5):411. doi:10.1016/J.ORET.2017.08.020
- Tao J, Wu H, Chen Y, et al. Use of iOCT in Vitreoretinal surgery for dense vitreous hemorrhage in a chinese population. Curr Eye Res. 2019;44(2):219-224. doi:10.1080/02713683.2018.1533982
- Kumar JB, Ehlers JP, Sharma S, Srivastava SK. Intraoperative OCT for uveitis-related vitreoretinal surgery in the DISCOVER study. Ophthalmol Retina. 2018;2(10):1041-1049. doi:10.1016/J.ORET.2018.02.013
- Browne AW, Ehlers JP, Sharma S, Srivastava SK. Intraoperative OCT-assisted chorioretinal biopsy in the DISCOVER study. Retina. 2017;37(11):2183. doi:10.1097/IAE.0000000000001522
- Vasconcelos HM, Lujan BJ, Pennesi ME, Yang P, Lauer AK. Intraoperative optical coherence tomographic findings in patients undergoing subretinal gene therapy surgery. Int J Retina Vitreous. 2020;6(1). doi:10.1186/S40942-020-00216-1
- Gregori NZ, Lam BL, Davis JL. Intraoperative use of microscope-integrated optical coherence tomography for subretinal gene therapy delivery. Retina. 2019;39(Suppl 1):S9-S12. doi:10.1097/IAE.0000000000001646
- Hsu ST, Gabr H, Viehland C, et al. Volumetric measurement of subretinal blebs using microscope-integrated optical coherence tomography. Transl Vis Sci Technol. 2018;7(2). doi:10.1167/TVST.7.2.19
- Ehlers JP, Petkovsek DS, Yuan A, Singh RP, Srivastava SK. Intrasurgical assessment of subretinal tPA injection for submacular hemorrhage in the PIONEER study utilizing intraoperative OCT. Ophthalmic Surg Lasers Imaging Retina. 2015;46(3):327. doi:10.3928/23258160-20150323-05
- Pujari A, Agarwal D, Chawla R, Kumar A, Sharma N. Intraoperative optical coherence tomography guided ocular surgeries: critical analysis of clinical role and future perspectives. Clin Ophthalmol. 2020;14:2427. doi:10.2147/OPTH.S270708
- Ehlers JP, Uchida A, Srivastava SK. Intraoperative optical coherence tomography-compatible surgical instruments for real-time image-guided ophthalmic surgery. Br J Ophthalmol. 2017;101(10):1306-1308. doi:10.1136/BJOPHTHALMOL-2017-310530
- Ehlers JP, Uchida A, Srivastava SK. The integrative surgical theater: combining intraoperative OCT and 3D digital visualization for vitreoretinal surgery in the DISCOVER study. Retina. 2018;38(Suppl 1):S88. doi:10.1097/IAE.0000000000001999
- Loewestein A, Schneider R, Barak A. First look: a head-mounted OR display. Accessed March 25, 2023. https://www.reviewofophthalmology.com/article/first-look-a-headmounted-or-display
- Carrasco-Zevallos OM, Keller B, Viehland C, et al. Optical coherence tomography for retinal surgery: perioperative analysis to real-time four-dimensional image-guided surgery. Invest Ophthalmol Vis Sci. 2016;57(9):OCT37-OCT50. doi:10.1167/IOVS.16-19277
- Carrasco-Zevallos OM, Keller B, Viehland C, et al. Live volumetric (4D) visualization and guidance of in vivo human ophthalmic surgery with intraoperative optical coherence tomography. Sci Rep. 2016;6:31689. doi:10.1038/srep31689
- Ahronovich EZ, Simaan N, Joos KM. A review of robotic and OCT-aided systems for vitreoretinal surgery. Adv Ther. 2021;38(5):2114. doi:10.1007/S12325-021-01692-Z
- Cereda MG, Parrulli S, Douven YGM, et al. Clinical evaluation of an instrument-integrated OCT-based distance sensor for robotic vitreoretinal surgery. Ophthalmol Sci. 2021;1(4):100085. doi:10.1016/J.XOPS.2021.100085