Vision degrading myodesopsia (VDM) refers to clinically significant vitreous floaters that merit therapeutic consideration. Article 1 of this 3-part series described etiology and diagnostics, while article 2 addressed surgical management. In this article, nonsurgical options will be reviewed.
ND:YAG LASER VITREOLYSIS
Nd:YAG lasers have increasingly been used for vitreous opacities. Unfortunately, no scientific studies have explored the molecular effects of YAG laser on vitreous. Clinically, only opacities relatively far from the fundus (2 mm to 4 mm) and crystalline lens (5 mm) have been treated, to avoid collateral damage.1,2 It is unclear how these distances are measured and with what degree of accuracy. Lastly, it can be quite challenging to know which specific vitreous opacities are most disturbing to the patient, and to visualize/target these for treatment.
The clinical efficacy of YAG laser vitreolysis has been difficult to determine due to the lack of studies employing objective, quantitative outcome measures.2 Multiple (1 to 6) sessions have been used, with variable success.2,3 Heterogeneity in treatment protocols and different criteria for case selection in various reports make it difficult, if not impossible, to draw conclusions about the efficacy of Nd:YAG laser vitreolysis. Indeed, a recent Cochrane review3 determined that randomized clinical trials are needed with sham controls and comparisons to pars plana vitrectomy.
One randomized prospective study used YAG laser to treat visible Weiss rings (Figure 1) in 52 subjects, comparing results to sham treatment.1 The study design was flawed, however, because it was not truly masked, the sham techniques were not properly validated, and there were biases.4 While the YAG laser group reported more frequent symptomatic improvement (54%) than controls (9%; P<.001), this only occurred in about half of the subjects.5 Furthermore, although VFQ-25 had statistical improvements, the findings had little clinical significance, because the magnitude for dependency, role difficulties, near vision, color vision, peripheral vision, and distance vision ranged from only 2.8% to 11.5%. In fact, general vision actually worsened by 3.5%.5
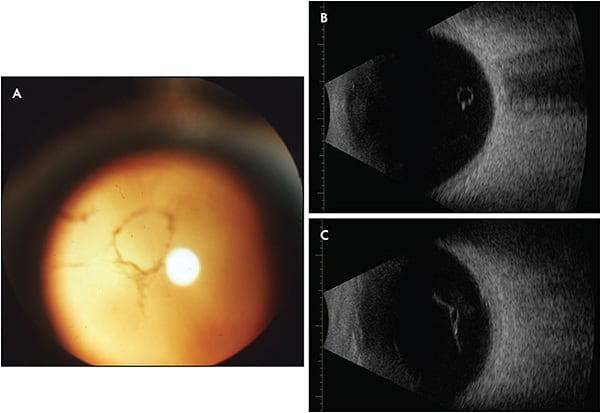
A recent study using objective indices of vitreous structure and visual function to compare patients who had previously undergone YAG laser to controls found that compared to 60 untreated subjects with vitreous floaters, 37 patients previously treated with YAG had 17% less vitreous echodensity (P=.003), but no differences in VFQ-39, best corrected visual acuity, or contrast sensitivity function (CSF).6 The investigators postulated that because YAG lasers have a photo-disruptor effect but cannot photoablate,5 they produce smaller vitreous opacities but a larger number of them, and the net effect on vision was the same; in other words, there was degradation in CSF and unhappiness with vision and quality of life. Interestingly, a small subgroup of individuals who were happy with the results of YAG laser had less vitreous density and better CSF than the unhappy patients. Thus, although evaluations were not performed prior to laser, it is a possible that Nd:YAG is appropriate for some patients, but it is still unclear which patients. Thus, a prospective randomized study of Nd:YAG laser treatment of vitreous is warranted, using uniform laser treatment parameters/techniques and objective quantitative outcome measures, as previously recommended.1-6
COMBINED NANOPARTICLE/LASER VITREOLYSIS
A challenge in any laser therapy of VDM is localizing and targeting the offending vitreous opacities. An alternative to an imaging solution is the use of nanoparticles with an affinity to vitreous opacities. Gold nanoparticles coated with hyaluronan (HA) were found to adhere in vitro to synthetic collagen membranes as well as human vitreous opacities excised at surgery.7 Positively charged carbon quantum dots were also tested with similar findings.8 The plasmon properties of these nanoparticles tremendously enhanced laser light absorption so that low energy (1000-fold lower than YAG) laser nanosecond pulsed-laser (561 nm) illumination resulted in the formation of nanobubbles that generate high-pressure shockwaves (Figure 2). In vitro studies demonstrated efficacy7,8 and subsequently confirmed in vivo in rabbits with no untoward effects (electrophysiology and histopathology).9 Human trials are being planned.
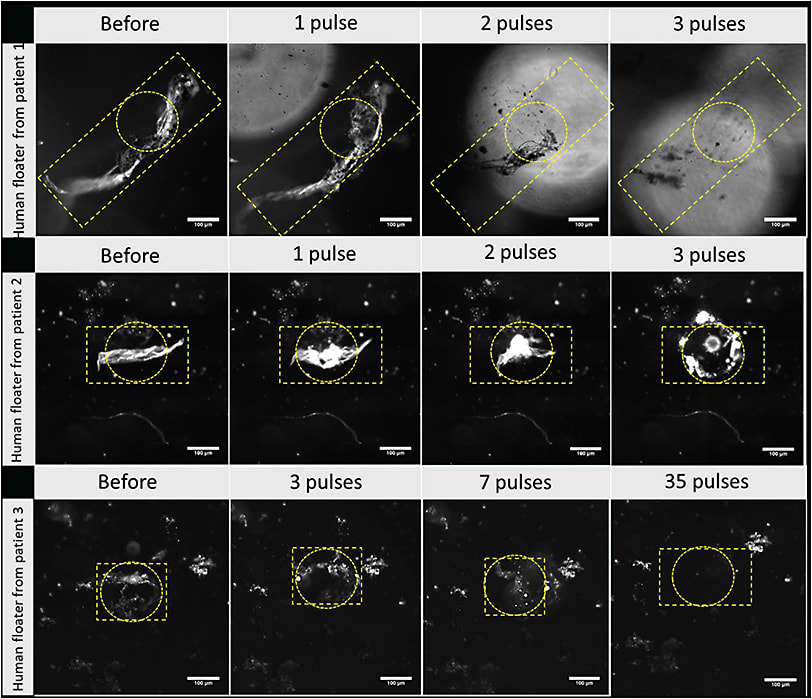
PHARMACOLOGIC VITREOLYSIS
Another nonsurgical approach to treat VDM is pharmacologic vitreolysis.10,11 To date, several agents have been tested in both laboratory and clinical settings, classified as liquefactants (drugs that liquefy gel vitreous) and interfactants (drugs that lyse vitreoretinal adhesion).12 Ocriplasmin is a nonspecific protease that has both liquefactant and interfactant properties, which has received United States and European approval for clinical use.13 However, neither this nor any other agents have been tested to dissolve vitreous opacities that cause VDM. The availability of various collagenases and matrix metalloproteinases14 would seem fertile ground for future research and development efforts toward an injectable drug that would dissolve vitreous opacities and cure VDM.
HIGH-INTENSITY FOCUSED ULTRASOUND
Ultrasound is intricately woven into ophthalmology with B-scan ultrasonography, phacoemulsification, and most recently ultrasound-powered vitrectomy.15 Because ultrasound is a mechanical wave phenomenon whereby heating results when ultrasonic energy is absorbed by tissue, HIFU may be a useful approach to treat VDM. By focusing ultrasound, tissue heating can be restricted to a well-defined zone, the length and width of which depends on ultrasound frequency and the aperture and focal range of the transducer (Figure 3). As early as 1980, HIFU was explored to treat vitreous membranes,16 but is best known for treating glaucoma via thermal ablation of the ciliary body.17
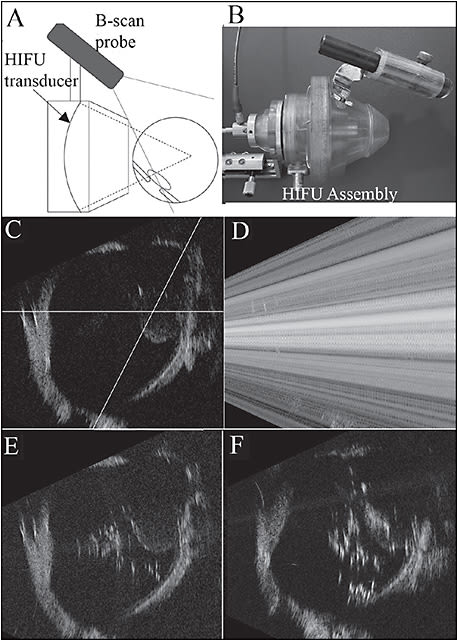
The challenge of treating VDM with the thermal effects of HIFU relates to localization of the ultrasound energy. This problem was addressed by using a diagnostic ultrasound probe attached externally to the HIFU assembly, calibrated so that the treatment zone could be imaged during treatment, as shown in Figure 3.16,18,19 Disadvantages of this system are that it requires a water bath for coupling to the eye and that it is based primarily upon a thermal mechanism of action.
Thermal effects, however, are not the sole means by which HIFU can alter tissue. Focused ultrasound produces a radiation force, which is mechanical and unrelated to cancer-causing ionizing radiation.20 High-intensity focused ultrasound can also induce “streaming,” in which a fluid medium (such as vitreous) will flow in response to the ultrasound beam.21 Another potential treatment mechanism is cavitation.22 Histotripsy23 is a form of cavitation in which short, repeated HIFU pulses cause rapid oscillation of microbubbles that can break down soft tissue without significant heating, similar to what has been done using laser-induced nanobubbles.7,9 In addition, lipid-coated perfluorocarbon (PFC) nanodroplets with diameters of a few hundred nanometers have been developed to serve as a diagnostic ultrasound contrast agent and as cavitation nuclei in therapeutic applications,24 including in ophthalmology.25 Figure 4 demonstrates activation of PFC nanodroplets in vitreous. The use of targeting PFC nanodroplets could thus substantially reduce required ultrasound intensity to produce the desired bioeffect, similar to the use of nanoparticles and laser.
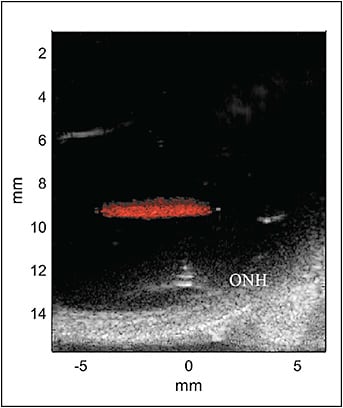
MYDRIATIC THERAPY
Patients with VDM sometimes describe decreased symptoms and better vision after pupil dilation. Although this phenomenon has not been formally investigated, Alfonso et al26 showed that in patients with diffractive intraocular lenses, increased pupil size was associated with improved CSF. In VDM, increased pupil size may be associated with not only improved CSF but also other aspects of visual function, such as reading accuracy and speed. Thus, there may be a potential role for 0.01% atropine drops, now FDA-approved to treat myopia progression in children.27
OPTICAL THERAPIES
A variety of optical methods have been tried to reduce suffering from the symptoms of VDM. Dark glasses can be limiting and at times worsen vision. Polarized lenses have not been shown to help with glare, nor have yellow lenses.28 However, optical correction of VDM might be possible if the specific optical aberrations can be characterized. Recent studies29 modeled human vitreous opacities that induce the visual phenomenon of floaters using optical software and image processing to study the Fresnel diffraction pattern. Nonsequential software based on Mie scattering theory was applied, determining that the distance between the vitreous opacities and the retina is inversely proportional to the sharpness of the edge of the shadow that will be cast. Characterizing and modulating light scattering30-32 would seem very relevant to nonsurgical management of VDM. RP
REFERENCES
- Shah CP, Heier JS. YAG laser vitreolysis vs sham YAG vitreolysis for symptomatic vitreous floaters: a randomized clinical trial. JAMA Ophthalmol. 2017;135:918-923. doi:10.1001/jamaophthalmol.2017.2388
- Milston R, Madigan MC, Sebag J. Vitreous floaters - etiology, diagnostics, and management. Surv Ophthalmol. 2016;61:211-227. doi:10.1016/j.survophthal.2015.11.008
- Kokavec J, Wu Z, Sherwin JC, Ang AJS, Ang GS: Nd:YAG laser vitreolysis versus pars plana vitrectomy for vitreous floaters. Cochrane Database Syst Rev. 2017;2017(6). doi:10.1002/14651858.CD011676.pub2
- Kokavec J, Ang GS, Sherwin JC. Methodological and efficacy issues in a randomized clinical trial investigating vitreous floater treatment. JAMA Ophthalmol. 2018;136(4):447-448. doi:10.1001/jamaophthalmol.2018.0026
- Sebag J. Methodological and efficacy issues in a randomized clinical trial investigating vitreous floater treatment. JAMA Ophthalmol. 2018;136(4):448. doi:10.1001/jamaophthalmol.2018.0212
- Nguyen JH, Nguyen-Cuu J, Yu F, et al. Assessment of vitreous structure and visual function after Nd:YAG laser vitreolysis. Ophthalmology. 2019;126(11):1517-1526. doi:10.1016/j.ophtha.2019.06.021
- Sauvage F, Fraire JC, Remaut K, et al. Photoablation of human vitreous opacities by light-induced vapor nanobubbles. ACS Nano. 2019;13(7):8401-8416. doi:10.1021/acsnano.9b04050
- Barras A, Sauvage F, De Hoon I, et al. Carbon quantum dots as a dual platform for the inhibition and light-based destruction of collagen fibers: implications for the treatment of eye floaters. Nanoscale Horizons. 2021;6(6):449-461. doi:10.1039/d1nh00157d
- Sauvage F, Nguyen VP, Li Y, et al. Laser-induced nanobubbles safely ablate vitreous opacities in vivo. Nat Nanotechnol. 2022;17(5):552-559. doi:10.1038/s41565-022-01086-4
- Sebag J. Pharmacologic vitreolysis. Retina. 1998;18(1):1-3.
- Sebag J. Is pharmacologic vitreolysis brewing? Retina. 2002;22(1):1-3.
- Sebag J. Pharmacologic vitreolysis - premise and promise of the first decade. Retina. 2009;29(7):871-874. doi:10.1097/IAE.0b013e3181ac7b3c
- Khoshnevis M, Sebag J. Pharmacologic vitreolysis with Ocriplasmin - rationale for use and therapeutic potential in vitreo-retinal disorders. BioDrugs. 2015;29(2):103-112. doi:10.1007/s40259-015-0120-y
- Apte SS, Parks WC. Metalloproteinases - a parade of functions in matrix biology and an outlook for the future. Matrix Biol. 2015;44-46:1-6. doi:10.1016/j.matbio.2015.04.005
- Stanga PE, Pastor-Idoate S, Zambrano I, Carlin P, McLeod D. Performance analysis of a new hypersonic vitrector system. PLoS One. 2017;12(6):1-15. doi:10.1371/journal.pone.0178462
- Coleman DJ, Lizzi FL, El-Mofty AAM, Driller J, Franzen LA. Ultrasonically accelerated resorption of vitreous membranes. Am J Ophthalmol. 1980;89(4):490-499. doi:10.1016/0002-9394(80)90056-2
- Burgess SEP, Silverman RH, Coleman DJ, et al. Treatment of glaucoma with high-intensity focused ultrasound. Ophthalmology. 1986;93(6):831-838. doi:10.1016/S0161-6420(86)33672-8
- Coleman DJ, Silverman RH, Iwamoto T, et al. Histopathologic effects of ultrasonically induced hyperthermia in intraocular malignant melanoma. Ophthalmology. 1988;95:970-981. doi:10.1016/S0161-6420(88)33088-5
- Lucas BC, Driller J, Iwamoto T, Silverman RH, Lizzi FL, Coleman DJ. Ultrasonically induced disruption and hemolysis of vitreous hemorrhages. Ultrasound Med Biol. 1989;15(1):29-37. doi:10.1016/0301-5629(89)90129-4
- Urban M. Production of acoustic radiation force using ultrasound: methods and applications. Expert Rev Med Devices. 2018;15(11):819-834. doi:10.1007/0-387-21518-2_4
- Nyborg WLM: Acoustic Streaming. Vol 2. Academic Press Inc; 1965. doi:10.1016/B978-0-12-395662-0.50015-1
- Miller DL: Overview of experimental studies of biological effects of medical ultrasound caused by gas body activation and inertial cavitation. Prog Biophys Mol Biol. 2007;93(1-3):314-330. doi:10.1016/j.pbiomolbio.2006.07.027
- Parsons JE, Cain CA, Abrams GD, Fowlkes JB. Pulsed cavitational ultrasound therapy for controlled tissue homogenization. Ultrasound Med Biol. 2006;32(1):115-129. doi:10.1016/j.ultrasmedbio.2005.09.005
- Dayton PA, Zhao S, Bloch SH, et al. Application of ultrasound to selectively localize nanodroplets for targeted imaging and therapy. Mol Imaging. 2006;5:160-74. PMID: 16954031; PMCID: PMC1752274.
- Silverman RH, Urs R, Burgess M, Ketterling JA, Tezel G. High-frequency ultrasound activation of perfluorocarbon nanodroplets for treatment of glaucoma. IEEE Trans Ultrason Ferroelectr Freq Control. 2022;69(6):1910-1916. doi:10.1109/TUFFC.2022.3142679
- Alfonso JF, Fernández-Vega L, Baamonde MB, Montés-Micó R. Correlation of pupil size with visual acuity and contrast sensitivity after implantation of an apodized diffractive intraocular lens. J Cataract Refract Surg. 2007;33(3):430-438. doi:10.1016/j.jcrs.2006.10.051
- Wei S, Li SM, An W, et al. Safety and efficacy of low-dose atropine eyedrops for the treatment of myopia progression in Chinese children a randomized clinical trial. JAMA Ophthalmol. 2020;138(11):1178-1184. doi:10.1001/jamaophthalmol.2020.3820
- Hwang AD, Tuccar-Burak M, Peli E. Comparison of pedestrian detection with and without yellow-lens glasses during simulated night driving with and without headlight glare. JAMA Ophthalmol. 2019;137(10):1147-1153. doi:10.1001/jamaophthalmol.2019.2893
- Lee CH, Woo GC, Huang SY, Sun HY, Yeh SM. Imaging vitreous floaters and cataracts with optical simulations. Optik (Stuttg). 2019;194:163075. doi:10.1016/j.ijleo.2019.163075
- Ansari RR, Dunker S, Suh K, Kitaya N, Sebag J. Quantitative molecular characterization of bovine vitreous and lens with non-invasive dynamic light scattering. Exp Eye Res. 2001;73:859-866.
- Arias A, Ginis H, Artal P. Light scattering in the human eye modelled as random phase perturbations. Biomed Opt Exp. 2018;9:2664-2670 doi:10.1364/BOE.9.002664
- Harmer SW, Luff AJ, Gini G. Optical scattering from vitreous floaters. Bioelectromagnetics. 2021. Published online ahead of print. doi: 10.1002/bem.22386