The current standard of care for patients with neovascular AMD (nAMD) and other retinal vascular diseases are intravitreal anti–vascular endothelial growth factor (VEGF) drugs administered at regular intervals. Patients usually maintain, and in about one-third of cases gain, visual function with proper and frequent treatment.1,2 The advent of anti-VEGF injections has revolutionized AMD treatment. However, there are still significant challenges to overcome: (1) the relatively rapid vitreous clearance of VEGF inhibitors requires injections every 1 to 4 months with worse visual outcomes when not maintaining a high enough injection frequency in real-world studies3,4; (2) adverse events are reported by >10% of subjects during 1 to 2 years of anti-VEGF treatment, including rare but vision-threatening complications, such as endophthalmitis, retinal occlusive vasculitis, and retinal detachment5; (3) treatment is associated with a physical and psychological burden for patients and their caregivers6,7; (4) reasons such as affordability (41.1%), lack of vision improvement (28.4%), and social factors can negatively impact adherence to treatment protocols.8 In one study, 22.2% of patients with nAMD were lost to follow-up within 12 months after starting anti-VEGF injections.9 Moreover, there is a considerable societal cost for the burden of care associated with anti-VEGF therapy on health care systems.10
Extending the durability of anti-VEGF therapy can be accomplished by different strategies. One is to develop sustained-release implants and depots, which are degradable polymers loaded with drug and injected into the eye to release the drug over a long period.11 Due to the fragility of biologic drugs, this approach is difficult and, to date, has not been tested in the clinic. Another is to use refillable reservoirs, which are nonbiodegradable ports surgically fixed to the sclera with an intraocular reservoir that can be refilled in the office at regular intervals.12 The Port Delivery System (PDS) with ranibizumab (Susvimo; Genentech) received FDA approval based on the phase 3 Archway trial, in which the primary endpoint of noninferior best corrected visual acuity (BCVA) in patients with PDS compared to monthly intravitreal ranibizumab injections.13 However, 2.0% of patients receiving an implant developed endophthalmitis during the trial, which represents a rate 3-fold higher than patients in the group treated with monthly intravitreal injections of ranibizumab.14 For context, the incidence of endophthalmitis after intravitreal anti-VEGF in large studies is approximately 0.03% to 0.05%.15 Finally, gene therapies, which use viral vectors to deliver anti-VEGF transgenes to target cells that results in continuous anti-VEGF production after a single administration, are being studied in clinical trials in phases 1 to 3.16
The pathophysiology of nAMD is a complex process requiring attention to other pathologic mechanisms than simply extracellular VEGF inhibition.11 Some patients still have poor clinical outcomes despite receiving regular anti-VEGF injections. For those, a new class of drugs with a novel mechanism of action that targets other complementary mediators involved in angiogenesis could potentially improve clinical efficacy.
TYROSINE KINASE INHIBITORS: A NOVEL MECHANISM OF ACTION
Nearly 60 different tyrosine kinase receptors (TKRs) regulate various aspects of cell biology. VEGF and platelet-derived growth factor (PDGF) receptors (VEGFR and PDGFR) are 2 types of TKRs that play an essential role in angiogenesis.17 Although current anti-VEGF drugs bind different isoforms of VEGF in the extracellular space, tyrosine kinase inhibitors (TKIs) bind to the intracellular domains of VEGFR, PDGFR, and other tyrosine kinase receptors, inhibiting downstream activation pathways irrespective of what is in the extracellular space (Figure 1).18 Given the vast number of TKRs in the body, selective TKIs are preferred, because they cause less off-target effects, potentially improving tolerability and theoretically maximizing efficacy.19 TKIs with higher affinity may also be preferred since they enable a similar clinical effect to be achieved with a lower drug dose, which may facilitate smaller or fewer implants and for a longer period of time.
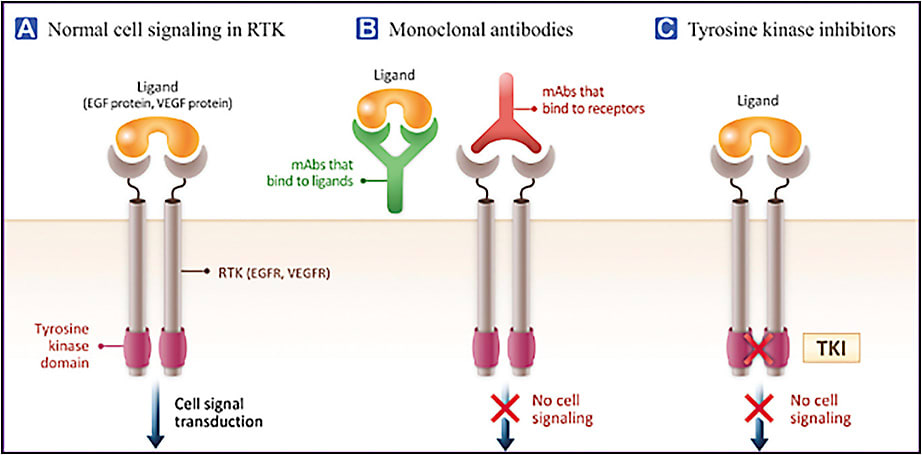
There are several TKIs in clinical development for the treatment of nAMD (Table 1). Sunitinib (GB-102; Graybug Vision) is a TKI that also targets VEGFR (1, 2, and 3) and PDGFR (α and β).17 It has been approved for the treatment of advanced renal cell carcinoma (RCC), gastrointestinal stromal tumor (GIST), and pancreatic neuroendocrine tumors (NETs). Vorolanib (EYP-1901; Eyepoint Pharmaceuticals) was developed on the same chemical scaffolding as sunitinib, also targeting all VEGFRs and PDGFRs.20 It is currently being investigated in patients with advanced solid tumors, such as RCC and lung cancer. When vorolanib was administered orally in a phase 2 trial for patients with nAMD, 36% of participants discontinued the study due to nonocular adverse events (AEs), mainly elevations in liver enzymes and gastrointestinal symptoms. Although the trial demonstrated clinical activity of oral vorolanib, the study was suspended due to systemic toxicity.21 Axitinib (OTX-TKI; Ocular Therapeutix and CLS-AX; Clearside Biomedical) is a selective inhibitor of VEGFR (1, 2, and 3) and PDGFR (α and β)22 approved for treating patients with advanced RCC.23 Of the TKIs being evaluated for retinal diseases, axitinib has the highest affinity for VEGFRs. The half maximal inhibitory concentration (IC50) for VEGFR2/KDR is 0.2 nM for axitinib, which is lower (higher affinity) than that for sunitinib (43 nM) or vorolanib (52 nM).24,25
GB-10217,23,45 1 mg | EYP-190124,24,46,47 0.44-3.09 mg | OTX-TKI22,23,29 0.2-0.6 mg | CLS-AX38 0.03-1 mg | |
Drug | Sunitinib | Vorolanib | Axitinib | Axitinib |
VEGFR affinity (IC50 in nM)a | VEGFR2/KDR: 43 | VEGFR2/KDR: 42 | VEGFR2/KDR: 0.2 | VEGFR2/KDR: 0.2 |
Delivery methodb | 27-gauge intravitreal | 22-gauge intravitreal | 27-gauge intravitreal | 30-gauge suprachoroidal |
Polymer | mPEG-PLGA microspheres | PVA/drug matrix plus a PVA-only coating | Cross-linked PEG hydrogel implant | Micronized suspension (no polymer) |
Degradation by-products | lactic acid, glycolic acid, and PEG | Erosion (solubilization, not degradable) | Water-soluble low-molecular-weight PEG | N/A |
Number of implantsb | N/A | 1-3 | 1 | N/A |
aA lower number means higher agonist activity.bCurrent state of development. Abbreviations: IC50, half maximal inhibitory concentration; KDR, kinase insert domain receptor; mPEG, methoxy-polyethylene glycol; nAMD, neovascular age-related macular degeneration; nM, nanomolar; PLGA, polylactic-co-glycolic acid; PVA, polyvinyl alcohol; PEG, polyethylene glycol; VEGFR2, vascular endothelial growth factor receptor 2. |
LONGER DURATION OF ACTION WITH SUSTAINED-RELEASE IMPLANTS
Characteristics of the drug, the type of polymer, the shape of the implant or microparticle, and specifics regarding their construction all impact the release rate of the drug. Polymer chemists have most commonly used either microparticles or implants for ocular studies. Implants can be made into different shapes and implanted through 22-gauge to 27-gauge needles or applicators. Microparticles, which range from 1 μm to 1,000 μm in size, can be delivered through even smaller needles but can be more prone to dispersion and migration, especially into the anterior chamber.26 Although solid implants are less likely to migrate, the release kinetics are dependent on polymer factors, including the degradation or erosion mechanism, the surface area of the implant, the solubility and diffusivity of the active agent in the implant matrix, the development of porosity as the matrix degradation proceeds, and implant dimensions.27 The implant size and polymer characteristics limit the amount of drug it can hold. Therefore, large or multiple implants may be needed for some drugs to achieve the release rate and kinetics required for efficacy.28 In the case of EYP-1901, a polyvinyl alcohol (PVA) coating covers the entire surface of the cylinder, aiming to deliver a consistent rate of diffusion of vorolanib out of the insert.28
Drug-release kinetics can also be dissolution-limited or solubility-limited and depend on the drug and polymer properties. Axitinib, in OTX-TKI and CLS-AX, has a lower water solubility than the other TKIs, allowing for dissolution-based controlled drug release.29 Absorbable polymers are temporary and ultimately break down as the drug is released. Biodegradation is a broad term encompassing biological and chemical-based processes that may involve human enzymes, microbial enzymes, and hydrolysis of biodegradable polymers that include hydrolytically and enzymatically susceptible bonds. Synthetic, absorbable polymers used in devices and drug-releasing implants are most commonly chemically (not enzymatically) hydrolyzed. Some implants are composed of bioerodible polymers (eg, PVA) instead, which are physically eroded by solubilization.30
EYP-1901 is a novel bioerodible intravitreal insert containing PVA. Polyvinyl alcohol has been used in FDA-approved commercial products using the Durasert drug delivery technology, including Vitrasert, Retisert, and Yutiq, and its intraocular safety is well established. Retisert contains microcrystalline cellulose, PVA, and magnesium stearate. Vitrasert contains magnesium stearate, PVA, and ethylene vinyl acetate (EVA). Yutiq contains a polyimide tube, PVA, silicone adhesive and water for injection. Neither Vitrasert, Retisert, nor Yutiq are fully erodible intravitreal products, because they contain nonerodable ingredients such as EVA, microcrystalline cellulose, and polyimide, respectively, whereas EYP-1901 contains only erodible components.
Polylactic-co-glycolic acid (PLGA) has been approved for the delivery of drugs in the dexamethasone implant (Ozurdex; Allergan) and bimatoprost implant (Durysta; Allergan). It is important to note that the degradation of polymers like polylactide and PLGA, which is used in GB-102, generates acidic monomers that can decrease the pH, resulting in a local acidic microenvironment that may potentially be harmful to intraocular structures.31 The bimatoprost implant is only approved for a single use. Poly(ethylene-glycol) (PEG) hydrogel is one of the most used polymers for drug delivery due to its water solubility, flexibility, and neutral surface charge. Hemophilia, phenylketonuria, lymphoblastic leukemia, and acromegaly, as well as hepatitis B and C, are just some of the clinical indications for commercially available PEG-based pharmaceutical systems.32 The biodegradation of the PEG hydrogel involves a change in its physical state from a cross-linked polymer hydrogel network, swollen with water, to a water-soluble low-molecular-weight polymer that facilitates clearance from the vitreous.30 The OTX-TKI uses PEG hydrogel. Additional details regarding the current development status of each sustained-release TKI are presented in Table 1.
CLINICAL TRIALS
All the sustained-release TKI candidates mentioned in this article are currently in clinical testing. As expected for early-stage clinical drugs, they have shown biological activity, minimal safety concerns (albeit the sample size is limited), and sustained concentrations in target tissues above the IC50 in preclinical animal studies.28,33-37 The study design and key baseline characteristics of clinical trials using sustained-release TKIs are summarized in Table 2, and efficacy and safety results are detailed in Table 3. Most are phase 1 trials designed to test mainly for safety and include a relatively small number of patients and, at times, different doses of the study drug. Given the early stage of clinical development and the fact that certain aspects of each study design and baseline characteristics vary, it is difficult to compare results directly. Nevertheless, it is encouraging to see that, overall, the investigational candidates seem well tolerated, and the efficacy results are promising.
GB-10240,48 1 mg | EYP-190128,46,49 0.44-3.09 mg | OTX-TKI25,43 0.2-0.6 mg | CLS-AX38 0.03-1 mg | ||
Design | Phase 2b, examiner-masked, randomized, parallel-arm design study compared with aflibercept | Phase 1, open-label, dose-escalating | Phase 1a, open-label, dose-escalating | Phase 1b, masked, randomized study compared with aflibercept | Phase 1, open-label, dose-escalating |
Inclusion criteria | Diagnosis of nAMD within 18 months; ≥3 prior anti-VEGF injections; last anti-VEGF treatment within the 21 days; demonstrated response to prior anti-VEGF therapy; BCVA 35-88 letters | Diagnosis of nAMD within 18 months; ≥3 prior anti-VEGF injections within 6 months; demonstrated response to prior anti-VEGF therapy; BCVA 25-75 letters | Phase 1a: Treatment naïve or previously treated nAMD; CST >300 μm; documented response to prior anti-VEGF therapy (if treated); no BCVA criteria | Phase 1b: ≥3 anti-VEGF within past year; last anti-VEGF within 1-4 weeks prior to the screening/baseline injection; demonstrated response to prior anti-VEGF; BCVA 24-83 letters | Diagnosis of nAMD within 18 months; active MNV; ≥2 prior anti-VEGF injections; BCVA 20-75 letters |
Primary endpoint(s) | Time to first rescue | Ocular and systemic TEAEs | Incidence of TEAEs | Incidence of TEAEs and SAEs | |
Rescue criteria | BCVA loss: ≥5 letters vs average of last 2 visits; ≥10 letters vs best on-study. increase in CST: ≥75 μm vs average of last 2 visits; ≥100 μm vs lowest on-study. | BCVA loss ≥10 letters from baseline; increase in CST >75 μm from baseline; new or worsening vision-threatening hemorrhage | Phase 1a; BCVA loss from previous best value: >15 letters; >10 letters on 2 consecutive visits, evidence of >75 µm CST from previous best value | Phase 1b; increase in CST >75 μm from best previous AND loss of ≥5 letters from best previous; loss of ≥10 letters from best VA; new or worsening hemorrhage | BCVA loss ≥10 letters from best measurement with exudation; increase in CST >75 µm; vision-threatening hemorrhage |
Average age | 78.5 (SD: 8.44) | 77.4 (range: 67-94) | 76.2 (SD: 5.3) | Cohort 1: 81.8 (range: 66-93); cohort 2: 78.2 (65-90) | |
Average BCVA at baseline, ETDRS letters | 70.5 (SD: 11.52) | 69 (range: 38-85) | Phase 1a: 51 + 4.7 | Cohort 1: 59.0 (29-74); cohort 2: 65.6 (52-75) | |
Baseline CFT, µm | 221.0 (SD: 47.42) | 299 (range: 204-441) | Phase 1a: 526 + 49 | Cohort 1: 231.2 (208-294); cohort 2: 209.4 (184-227) | |
Number of implants | N/A | 1-3 | 1-3 | 1 | N/A |
Abbreviations: BCVA, best-corrected visual acuity; CST, central subfield thickness; ETDRS, Early Treatment Diabetic Retinopathy Study; MNV, macular neovascularization; nAMD, neovascular age-related macular degeneration; SAE, serious adverse event; SD, standard deviation; TEAE, treatment-emergent adverse event; VA, visual acuity; VEGF, vascular endothelial growth factor. |
GB-10240,48 1 mg | EYP-190128,46,49 0.44-3.09 mg | OTX-TKI25,43 0.2-0.6 mg | |
Change in BCVA (ETDRS) from baseline (6/12 months) | -5.7/-11.5 letters | -2.5/-4.1 letters | +1.3/+1.1 lettersb |
Change in CST from baseline (6/12 months) | +44.3/+44.2 µm | -3.4/-2.8 µm | -103.9/-86.8 µmb |
Rescue-free patients at 6/12 months | 48%/30% | 53%/35% | 61%/ongoingb |
Dispersion/migration to the anterior chamber | 3/21 patients | None | None |
Vitreous floaters | 5/21 patients | None | None |
Ocular or systemic SAEs | None | None | None |
Time to complete implant disappearance | Unknownc | Essentially drug-depleted at 8 months, but it is unclear the length of time needed for the PVA coating to erode completely | Complete degradation at 9-10.5 months |
Note: Phase 1 trials (EYP-1901 & OTX-TKI) have multiple cohorts receiving different doses of the study drug. CLS-AX is not included in this table due to a lack of significant follow-up (>6 months).aRegulatory agencies have made no conclusions about the efficacy and safety results shown in this table. bInterim results as most patients have not reached 12 months of follow-up. cData from clinical trials are not available yet. In preclinical studies, the depot is fully resorbed by 12 to 14 weeks.50 Abbreviations: BCVA: best-corrected visual acuity; ETDRS: Early Treatment Diabetic Retinopathy Study; CST: central subfield thickness; PVA, polyvinyl alcohol; SAE: serious adverse event. |
CLS-AX has been investigated in a phase 1/2a trial (OASIS) in which 2 cohorts of patients received 0.03 mg to 0.1 mg of CLS-AX administered through suprachoroidal injection, following a single intravitreal aflibercept injection, with an additional cohort planned to receive 0.5 mg of CLS-AX. A preliminary safety overview revealed no serious adverse events (SAEs); no treatment-emergent adverse events (TEAEs) related to aflibercept, CLS-AX, or suprachoroidal injection procedure; no dispersion into the vitreous; and no AEs related to intraocular pressure, inflammation, or vasculitis. No study suspension or stopping rules were met. Efficacy analysis is limited given the absence of a follow-up longer than 3 months and other study limitations.38
GB-102 is currently the only candidate in phase 2b stage of development. The ALTISSIMO trial was initially designed to have 2 dose cohorts of 1 mg and 2 mg, injected every 6 months.39 Based on interim safety analysis, the 2 mg strength was discontinued after the first dose, and patients in that cohort received 1 mg for their second dose instead. In the 1 mg cohort, based on the interim analysis, there were no drug-related SAEs, no TEAEs leading to drug discontinuation, no AEs requiring surgical intervention, and no vision-threatening inflammation. However, drug dispersion was noticed in the clinical trial. In the 1 mg cohort, 4 of 21 patients had intraocular inflammation that resolved with a short course of topical steroids, 3 of 21 patients had transient particles in the anterior chamber, and 5 of 21 patients had vitreous floaters. It is suspected that the particle dispersion caused a decrease in BCVA in some patients. Following the trial results, a third formulation version of GB-102 is being designed to improve aggregation performance of the microparticles and stability.40
EYP-1901 has been studied in a phase 1 trial (DAVIO), with 4 cohorts receiving doses ranging from 0.44 to 3.09 mg. Patients received 1 to 3 intravitreal implants depending on the dose. At 6 and 12 months, 53% and 35% of patients did not require a rescue anti-VEGF injection, respectively, with stable mean BCVA and CSFT. There was a U-shaped dose-response curve with rates of treatment burden reduction in the low, mid, and high doses of 66%, 88%, and 47%, respectively. There were no significant safety findings, no drug-related ocular or systemic SAEs, dose-limiting toxicity, vitreous floaters, endophthalmitis, retinal detachment, implant migration to the anterior chamber, posterior-segment inflammation, occlusive events, or retinal vasculitis. Drug release from the PVA implant is essentially depleted past 8 months, but it is unclear the length of time needed for the implant coat to erode completely.41 Of note, in preclinical studies, the implants were still visible 12 months after injection.28 A phase 2 randomized study (DAVIO 2) of EYP-1901 2.06 mg (2 implants) and 3.09 mg (3 implants) compared to aflibercept in subjects with nAMD is ongoing.42
OTX-TKI was studied in 2 phase 1 studies. The first phase 1a trial included 4 cohorts receiving 0.2 (1 implant) to 0.6 mg (3 implants in early cohorts, 1 implant in later cohorts) of the drug. A notable difference in this study design compared to the other studies mentioned above was the inclusion of both previously treated and treatment-naïve nAMD patients. Similar to the other TKI candidates, OTX-TKI was generally well tolerated with no significant safety findings. There were no ocular SAEs, such as endophthalmitis or retinal detachment, no implant migration to the anterior chamber, no observation of elevated intraocular pressure, and no retinal vasculitis. A preliminary biologic signal of clinically meaningful decrease in the retinal fluid was observed by month 2 in the higher-dose cohorts. Of note, in the highest-dose cohort (0.6 mg), 5 of 6 patients were treatment-naïve, and 80% did not require rescue by month 6, with meaningful reductions in retinal fluid without any anti-VEGF injection. Figure 2 shows one of these participants showing an excellent clinical response without the need for rescue anti-VEGF injections for up to 15 months, demonstrating the potential of TKIs as monotherapy for nAMD patients, with complete resolution of the retinal fluid. The hydrogel matrix of the implant biodegraded by 7.5 to 8 months, and any remaining drug visually cleared by 9 to 10.5 months (single implant of 0.2 mg).25,43 A second phase 1b study with randomization of patients to an OTX-TKI 0.6 mg single implant versus intravitreal aflibercept dosed on label is expected to be reported at the American Academy of Ophthalmology meeting in 2022.44
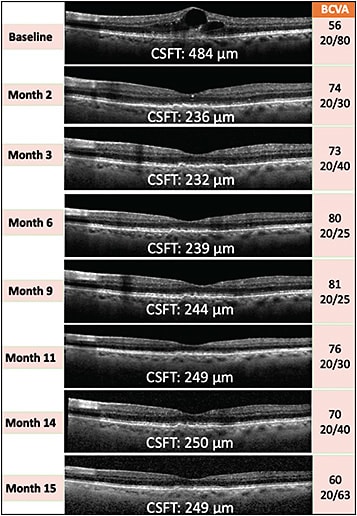
FUTURE CONSIDERATIONS
It is encouraging to see a class of drugs with a 2-pronged approach to address the unmet needs of nAMD patients: a novel mechanism of action and prolonged, consistent dosing. Sustained-release TKIs will likely also be helpful for the treatment of other vascular retinal diseases, such as diabetic retinopathy, diabetic macular edema, and retinal vein occlusion. An ideal sustained-release TKI may have the following characteristics: (1) pan-VEGF inhibition by a potent and selective TKI; (2) an implant with no dispersion-associated or inflammation-associated issues, that can be delivered with the smallest possible needle; (3) near-zero order drug release; (4) complete degradation of the implant into constituent components that are well tolerated by the eye at a known and reproducible rate; (5) durable treatment effect (≥6 months) with minimal rescue treatments required; (6) consistent retinal thickness reduction with minimal fluid fluctuations between treatments; (7) stable or improved BCVA (dependent on the enrolled population). The preliminary results from clinical trials show promise that one or more of these investigational candidates meet these criteria and will soon be available in our clinical practices. RP
References
- Solomon SD, Lindsley K, Vedula SS, Krzystolik MG, Hawkins BS. Anti-vascular endothelial growth factor for neovascular age-related macular degeneration. Cochrane Database Syst Rev. 2019;3(3):CD005139. doi:10.1002/14651858.CD005139.pub4
- Schmidt-Erfurth U, Chong V, Loewenstein A, et al. Guidelines for the management of neovascular age-related macular degeneration by the European Society of Retina Specialists (EURETINA). Br J Ophthalmol. 2014;98(9):1144-1167. doi:10.1136/bjophthalmol-2014-305702
- Spooner KL, Mhlanga CT, Hong TH, Broadhead GK, Chang AA. The burden of neovascular age-related macular degeneration: a patient’s perspective. Clin Ophthalmol Auckl NZ. 2018;12:2483-2491. doi:10.2147/OPTH.S185052
- Okada M, Mitchell P, Finger RP, et al. Nonadherence or nonpersistence to intravitreal injection therapy for neovascular age-related macular degeneration: a mixed-methods systematic review. Ophthalmology. 2021;128(2):234-247. doi:10.1016/j.ophtha.2020.07.060
- Holekamp NM. Review of neovascular age-related macular degeneration treatment options. Am J Manag Care. 2019;25(10 Suppl):S172-S181.
- Gualino V, Fourmaux E, Grenet T, Zerbib J, Wolff B. Patient experience of anti-vegf intravitreal injection. J Fr Ophtalmol. 2020;43(10):1047-1053. doi:10.1016/j.jfo.2020.02.006
- Thier A, Holmberg C. The patients’ view: age-related macular degeneration and its effects - a meta-synthesis. Disabil Rehabil. 2022;44(5):661-671. doi:10.1080/09638288.2020.1775901
- Kelkar A, Webers C, Shetty R, et al. Factors affecting compliance to intravitreal anti-vascular endothelial growth factor therapy in Indian patients with retinal vein occlusion, age-related macular degeneration, and diabetic macular edema. Indian J Ophthalmol. 2020;68(10):2143-2147. doi:10.4103/ijo.IJO_1866_19
- Obeid A, Gao X, Ali FS, et al. Loss to follow-up among patients with neovascular age-related macular degeneration who received intravitreal anti-vascular endothelial growth factor injections. JAMA Ophthalmol. 2018;136(11):1251-1259. doi:10.1001/jamaophthalmol.2018.3578
- Schippert AC, Jelin E, Moe MC, Heiberg T, Grov EK. The impact of age-related macular degeneration on quality of life and its association with demographic data: results from the NEI VFQ-25 questionnaire in a Norwegian population. Gerontol Geriatr Med. 2018;4:2333721418801601. doi:10.1177/2333721418801601
- Hussain RM, Shaukat BA, Ciulla LM, Berrocal AM, Sridhar J. Vascular endothelial growth factor antagonists: promising players in the treatment of neovascular age-related macular degeneration. Drug Des Devel Ther. 2021;15:2653-2665. doi:10.2147/DDDT.S295223
- Campochiaro PA, Marcus DM, Awh CC, et al. The port delivery system with ranibizumab for neovascular age-related macular degeneration: results from the randomized phase 2 Ladder clinical trial. Ophthalmology. 2019;126(8):1141-1154. doi:10.1016/j.ophtha.2019.03.036
- Holekamp NM, Campochiaro PA, Chang MA, et al. Archway randomized phase 3 trial of the Port Delivery System with ranibizumab for neovascular age-related macular degeneration. Ophthalmology. 2022;129(3):295-307. doi:10.1016/j.ophtha.2021.09.016
- SUSVIMOTM (ranibizumab injection) for intravitreal use via SUSVIMO ocular implant. Prescribing information. Published online April 2022. Accessed August 8, 2022. https://www.gene.com/download/pdf/susvimo_prescribing.pdf
- Pancholy M, Storey PP, Levin HJ, et al. Endophthalmitis following intravitreal anti-vascular endothelial growth factor therapy: changes in incidence and outcomes over a 9-year period. Curr Eye Res. 2021;46(9):1370-1377. doi:10.1080/02713683.2021.1874023
- Al-Khersan H, Hussain RM, Ciulla TA, Dugel PU. Innovative therapies for neovascular age-related macular degeneration. Expert Opin Pharmacother. 2019;20(15):1879-1891. doi:10.1080/14656566.2019.1636031
- Esteban-Villarrubia J, Soto-Castillo JJ, Pozas J, et al. Tyrosine Kinase Receptors in oncology. Int J Mol Sci. 2020;21(22):E8529. doi:10.3390/ijms21228529
- Kim K, Khang D. Past, present, and future of anticancer nanomedicine. Int J Nanomedicine. 2020;15:5719-5743. doi:10.2147/IJN.S254774
- Salgia R, Sattler M, Scheele J, Stroh C, Felip E. The promise of selective MET inhibitors in non-small cell lung cancer with MET exon 14 skipping. Cancer Treat Rev. 2020;87:102022. doi:10.1016/j.ctrv.2020.102022
- Bendell JC, Patel MR, Moore KN, et al. Phase I, first-in-human, dose-escalation study to evaluate the safety, tolerability, and pharmacokinetics of vorolanib in patients with advanced solid tumors. The Oncologist. 2019;24(4):455-e121. doi:10.1634/theoncologist.2018-0740
- Cohen MN, O’Shaughnessy D, Fisher K, et al. APEX: a phase II randomised clinical trial evaluating the safety and preliminary efficacy of oral X-82 to treat exudative age-related macular degeneration. Br J Ophthalmol. 2021;105(5):716-722. doi:10.1136/bjophthalmol-2020-316511
- Hu-Lowe DD, Zou HY, Grazzini ML, et al. Nonclinical antiangiogenesis and antitumor activities of axitinib (AG-013736), an oral, potent, and selective inhibitor of vascular endothelial growth factor receptor tyrosine kinases 1, 2, 3. Clin Cancer Res Off J Am Assoc Cancer Res. 2008;14(22):7272-7283. doi:10.1158/1078-0432.CCR-08-0652
- Gross-Goupil M, François L, Quivy A, Ravaud A. Axitinib: a review of its safety and efficacy in the treatment of adults with advanced renal cell carcinoma. Clin Med Insights Oncol. 2013;7:269-277. doi:10.4137/CMO.S10594
- Eyepoint Pharmaceuticals, Inc. Form 8-K. Published online September 15, 2020. Accessed August 11, 2022. https://sec.report/Document/0001564590-20-043596/
- Moshfeghi, Andrew A. Intravitreal hydrogel-based axitinib implant (OTX-TKI) for the treatment of neovascular AMD. Presented at: 19th Annual Angiogenesis, Exudation, and Degeneration Meeting, virtual edition; February 11, 2022. Accessed July 25, 2022. https://ocutx.gcs-web.com/static-files/836829a7-702f-4466-9c63-b1b5521e4309
- Yun S, Huang JJ. Routes for drug delivery: sustained-release devices. Dev Ophthalmol. 2016;55:84-92. doi:10.1159/000434692
- Cao Y, Samy KE, Bernards DA, Desai TA. Recent advances in intraocular sustained-release drug delivery devices. Drug Discov Today. 2019;24(8):1694-1700. doi:10.1016/j.drudis.2019.05.031
- EyePoint Pharmaceuticals, Inc. United States patent application publication: bioerodible ocular drug delivery insert and therapeutic method. Published online June 2, 2022. Accessed July 26, 2022. https://patentimages.storage.googleapis.com/ed/69/76/51c0b2c876fc9b/US20220168142A1.pdf
- National Center for Biotechnology Information. PubChem Compound summary for CID 6450551, Axitinib. https://pubchem.ncbi.nlm.nih.gov/compound/6450551 . Accessed August 2, 2022.
- Uhrich K, Abdelhamid D. Biodegradable and bioerodible polymers for medical applications. In: Poole-Warren L, Martens P, Green R, eds. Biosynthetic Polymers for Medical Applications. Elsevier; 2016:63-83. doi:10.1016/B978-1-78242-105-4.00003-1
- Keles H, Naylor A, Clegg F, Sammon C. Investigation of factors influencing the hydrolytic degradation of single PLGA microparticles. Polym Degrad Stab. 2015;119:228-241. doi:10.1016/j.polymdegradstab.2015.04.025
- Sanchez Armengol E, Unterweger A, Laffleur F. PEGylated drug delivery systems in the pharmaceutical field: past, present and future perspective. Drug Dev Ind Pharm. Published online July 21, 2022:1-11. doi:10.1080/03639045.2022.2101062
- Tsujinaka H, Fu J, Shen J, et al. Sustained treatment of retinal vascular diseases with self-aggregating sunitinib microparticles. Nat Commun. 2020;11(1):694. doi:10.1038/s41467-020-14340-x
- Ren C, Shi H, Jiang J, et al. The effect of CM082, an oral tyrosine kinase inhibitor, on experimental choroidal neovascularization in rats. J Ophthalmol. 2017;2017:6145651. doi:10.1155/2017/6145651
- Narvekar P, Bhatt P, Fnu G, Sutariya V. Axitinib-loaded poly(lactic-co-glycolic acid) nanoparticles for age-related macular degeneration: formulation development and in vitro characterization. Assay Drug Dev Technol. 2019;17(4):167-177. doi:10.1089/adt.2019.920
- Kahn E, Patel C, Priem M, et al. A safety and pharmacokinetic study of a novel hydrogel-based axitinib intravitreal implant (OTX-TKI) in non-human primates. Invest Ophthalmol Vis Sci. 2022;63(7):297-F0100-297-F0100.
- Elhayek RF, Jarrett T, Lattrell Z, et al. Efficacy of a 6 month sustained hydrogel delivery system for tyrosine kinase inhibitors in a vegf induced retinal leakage model. Invest Ophthalmol Vis Sci. 2017;58(8):1968-1968.
- Clearside Medical. OASIS phase 1/2a clinical trial safety results. 2021. Accessed July 26, 2022. https://ir.clearsidebio.com/static-files/73cbcd76-02e8-472b-88dd-c8985318c66e
- Graybug Vision Inc. A phase 2b multicenter dose-ranging study evaluating the safety and efficacy of a long-acting intravitreal sunitinib malate depot formulation (GB-102) compared to intravitreal aflibercept in subjects with neovascular (wet) age-related macular degeneration (ALTISSIMO study). Last updated January 19, 2022. Accessed July 31, 2022. https://clinicaltrials.gov/ct2/show/NCT03953079?term=gb-102&draw=2&rank=2
- ALTISSIMO full-data analysis 12-month treatment phase. News release. Accessed July 27, 2022. https://investors.graybug.vision/static-files/bb8f24d7-aef7-4d1b-8b27-09c0b5286c16
- Eyepoint Pharmaceuticals Inc. EyePoint pharmaceuticals investor day presentation. Accessed August 18, 2022. https://investors.eyepointpharma.com/static-files/2dbfaed2-4b51-428e-9640-a99414d24d97
- Study of EYP-1901 in subjects with wet age related macular degeneration (wAMD). ClinicalTrials.gov identifier: NCT05381948. Last updated July 26, 2022. Accessed August 8, 2022. https://clinicaltrials.gov/ct2/show/NCT05381948?term=EYP-1901&draw=2&rank=3
- Wong JG, Chang A, Guymer RH, et al. Phase 1 study of an intravitreal axitinib hydrogel-based implant for the treatment of neovascular age-related macular degeneration (nAMD). Invest Ophthalmol Vis Sci. 2021;62(8):218-218.
- Study evaluating the treatment of OTX-TKI for subjects with neovascular age-related macular degeneration. ClinicalTrials.gov identifier: NCT04989699. Last updated February 7, 2022. Accessed August 8, 2022. https://www.clinicaltrials.gov/ct2/show/NCT02116010
- Chuidian M. Update on GB-102 for neovascular age-related macular degeneration. News release. 2022. Accessed July 25, 2022. https://investors.graybug.vision/static-files/3323a8ed-30cc-48f1-ad4a-1dc451740305
- Delivering innovation to the eye: investor presentation. Published online May 13, 2022. Accessed July 26, 2022. https://sec.report/Document/0001564590-22-020081/
- Liang C, Yuan X, Shen Z, Wang Y, Ding L. Vorolanib, a novel tyrosine receptor kinase receptor inhibitor with potent preclinical anti-angiogenic and anti-tumor activity. Mol Ther Oncolytics. 2022;24:577-584. doi:10.1016/j.omto.2022.01.001
- A depot formulation of sunitinib malate (GB-102) compared to aflibercept in subjects with wet amD (ALTISSIMO). Clinicaltrials.gov identifier: NCT03953079. Last updated January 19, 2022. Accessed August 29, 2022. https://clinicaltrials.gov/ct2/show/results/NCT03953079
- Singh RP. 12 month results of a tyrosine kinase inhibitor (vorolanib) in a bioerodible Durasert insert for previously treated neovascular amd: the DAVIO trial. Presented at: 40th Annual Meeting of the American Society of Retina Specialists; New York, NY. July 15, 2022.
- Graybug Vision Inc. Clinical study protocol. GBV-102-002. Published online September 8, 2020. Accessed August 12, 2022. https://clinicaltrials.gov/ProvidedDocs/79/NCT03953079/Prot_000.pdf