Retinal pathologies, including diabetic retinopathy (DR), age-related macular degeneration (AMD), and glaucoma, are leading causes of ocular morbidity worldwide.1 Early detection and treatment of these pathologies has the potential to result in more positive visual outcomes. Currently, spectral domain optical coherence tomography (SD-OCT) is standard of care for imaging and diagnosis of retinal pathologies, visualizing structural change in disease state with high resolution and sensitivity.2 However, SD-OCT is primarily structural; it cannot provide insight into retinal oxidative stress and damage nor metabolic function.3
As a highly metabolic tissue, the retina is susceptible to stressors that limit energy supply. Inefficient mitochondria result in metabolic dysfunction, which has been implicated in the pathogenesis of DR, AMD, and glaucoma.5-7 Oxidative stress is a primary driver of mitochondrial dysfunction over time, leading to impaired cellular respiration organelle death.5,6 Oxidized flavoproteins are mitochondrial proteins that reflect oxidative stress. When in a pro-oxidative environment, these proteins display properties of autofluorescence. When excited by blue-spectrum light, they emit green-spectrum light, and this phenomenon is termed flavoprotein fluorescence (FPF).8,9 Flavoprotein fluorescence can be harnessed with retinal metabolic imaging devices, to detect fluorescence of these proteins and, by extension, the first signs of retinal pathology before it manifests in gross anatomic change.3 This, in turn, can lead to earlier treatment and positive visual outcomes. This review article will summarize FPF physiology and its application to retinal imaging by examining the role of mitochondrial dysfunction in retinal diseases, preclinical FPF studies, and recent clinical studies exploring FPF in patients with DR, AMD, and glaucoma.
MITOCHONDRIAL DYSFUNCTION AT THE CELLULAR LEVEL
Mitochondria play crucial roles in cellular function, including adenosine triphosphate (ATP) production, regulation of cellular metabolism, calcium signaling, inflammatory signaling, and control of apoptosis.3,10,11 Dysfunctional mitochondria can therefore lead to decreased energy production, increased inflammation, and apoptosis. Increased reactive oxidative species (ROS) combined with decreased host antioxidant defenses are known to generate a pro-oxidative state, which contributes to mitochondrial dysfunction through alteration of mtDNA, lipid oxidation, and protein oxidation. Alterations in mtDNA can produce defective electron transport chain proteins and protein oxidation compounds this effect, causing inefficient cellular respiration and further favors ROS production.12,13 Peroxidation of lipids can increase apoptosis and autophagy.14 Because of their crucial role in cellular energy production, highly metabolic tissues are susceptible to damage in the setting of mitochondrial dysfunction. The retina is a primary example of this due to high mitochondrial density in retinal pigment epithelium and photoreceptor layers.3,14 Mitochondrial dysfunction in these retinal layers has been implicated in retinal pathogenesis of AMD, DR, and glaucoma.4,15,16
DIABETIC RETINOPATHY AND MITOCHONDRIAL DYSFUNCTION
Hyperglycemia is central in DR pathogenesis because it functions to upregulate ROS in the form of mitochondrial superoxide and peroxide.17-19 As these species overwhelm oxidative defenses, mtDNA damage and membrane disruption occurs, releasing proapoptotic markers, such as cytochrome c (Figure 1).20 Retinal rat models of diabetes have also been shown to have diminished antioxidant defenses, such as manganese superoxidase dismutase, an inhibitor of ROS generation from complex III, correlate with increases in apoptosis of mitochondrially dense retinal ganglion cells.21 Accordingly, antioxidant supplementation with superoxide dismutase has been shown to be protective of these effects in mouse models.22
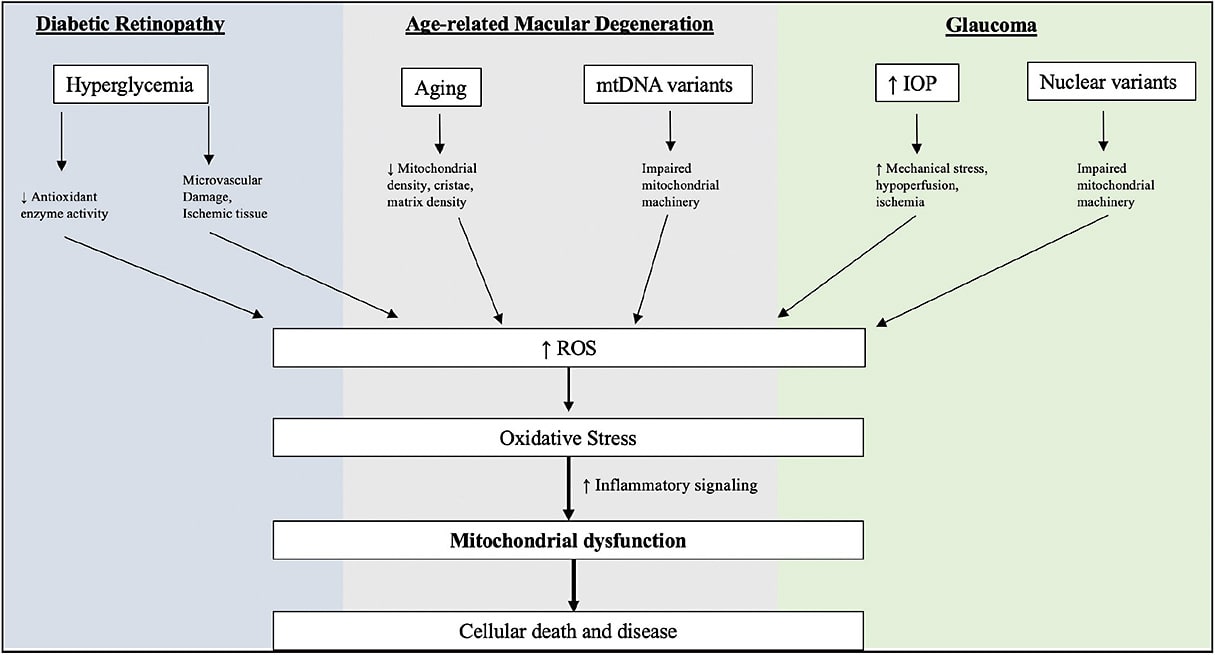
AMD AND MITOCHONDRIAL DYSFUNCTION
Oxidative stress is a known contributor to AMD.23 Mitochondria are exposed to increased burden of oxidative insult during aging, increasing cumulative insult to mtDNA and proteins (Figure 1).24 Certain mtDNA mutations are more susceptible than others and, for this reason, are associated with drusenoid phenotypes and retinal pigment epithelium (RPE) abnormalities.25 Scanning electron microscopic studies have noted morphologic changes in RPE mitochondria in AMD patients, including decreased cristae and matrix density. Patients with AMD have also been shown to have decreased RPE mitochondrial load compared to age-matched controls.26 Similarly to DR, antioxidant pathways are downregulated in nonexudative AMD models and this can offset with antioxidant supplementation.27,28
GLAUCOMA AND MITOCHONDRIAL DYSFUNCTION
Mitochondrial dysfunction has also been implicated in glaucoma. Human retinal ganglion cells (RGC) cultured with increased hydrostatic pressure results in abnormal mitochondrial fission, decreased cristae, and reduced ATP production.29 Decreases in RGC function that are seen in glaucoma have also been linked to mitochondrial mutations, defective mitophagy, release of mitochondrial derived molecular patterns, oxidative stress, and inflammatory signaling (Figure 1). Glaucoma models of rats with high intraocular pressure have been shown to have increased RGC death when fed antioxidant-deficient diets, offsetting the antioxidant depleting mechanisms described beforehand.30 Antioxidant supplementation has also been shown to reduce RGC loss and preserve retinal nerve fiber layer thickness.31
FLAVOPROTEINS AND MITOCHONDRIAL DYSFUNCTION
Mitochondrial flavoproteins are proteins containing a derivative of riboflavin, either flavin adenine dinucleotide or flavin mononucleotide. These proteins serve integral roles in mitochondrial function during electron transport.32 In 1979, two studies identified these flavoproteins to exhibit autofluorescence, termed FPF.8,9 Peak excitation ranges from 430 nm to 470 nm, and peak emission ranges from 520 nm to 540 nm.7,30 Without appropriate emission filters, other fluorophores confound this signal, such as vitamin A, nicotinamide adenine dinucleotide, melanin, and Bruch’s membrane. Lipofuscin, whose peak excitation is around 510 nm and peak emission is from 620 nm to 630 nm, is a primary example.34
FLAVOPROTEIN FLUORESCENCE PRECLINICAL STUDIES
Flavoprotein fluorescence has shown to be a marker of mitochondrial function in vitro. A study performed by Elner et al exposed human RPE cells to hydrogen peroxide and ceramide to induce oxidative stress, which resulted in significant increases in FPF. However, an antioxidant restored retinal FPF signals to control levels.35 Later studies done by Field et al demonstrated that dose-dependent increases in peroxide-induced oxidative stress increased FPF correspondingly. Additionally, mitochondrial membrane potential decreased and proapoptotic markers increased. Increases in FPF preceded the increase in proapoptotic markers, suggesting oxidation detected by FPF occurs before cascades, which would result in cellular death.36 This study also cocultured RPE cells with monocytes representing a proinflammatory environment, finding that FPF correlated with levels of proinflammatory cytokines.36 The initial data reviewed above demonstrated retinal FPF might be an in vivo marker for mitochondrial dysfunction (Table 1). Given the potential for this technique to detect change before structural retinal damage manifests, it was incorporated into a retinal metabolic imaging device.
TITLE | AUTHOR, DATE | STUDY MODEL | INTERVENTION | FLAVOPROTEIN FLUORESCENCE RESULTS | OTHER RESULTS | |
Sensitivity of flavoprotein fluorescence to oxidative state in single isolated heart cells | Koke et al, 1981 | Isolated rat cardiac cells | Cells exposed to no intervention, cyanide, 2-4 dinitrophenol, and hypoxia | Flavoprotein fluorescence is sensitive to redox state and distinct from NADH fluorescence | n/a | |
Fluorescence spectroscopy and imaging of myocardial apoptosis | Ranji et al, 2006 | Open chest rabbit model | Myocardial ischemia reperfusion injury | Fluorescence intensity increases after ischemic event in infarct zone compared to noninfarcted zone. | Fluorescence spatially correlates with infarcted tissue region. | |
In vivo imaging of flavoprotein fluorescence during hypoxia reveals the importance of direct arterial oxygen supply to cerebral cortex tissue | Chisholm et al, 2016 | Exopsed cerebral mouse cortex | Manipulation of inspired ocygen concentration | Fluorescence intensity decreased in response to decreased FiO2 in room air (P<.001) | n/a | |
Retinal flavoprotein autofluorescence as a measure of retinal health | Elner et al, 2008 | Cultured human retinal pigment epithelial cells | Exposed to H2O2 or C2 ceramide in presence of either N-acetylcysteine or dihydroceramide C2 | Mean retinal fluorescence was significantly increased in H2O2 treated cells compared to no treatment and H2O2 + NAC (P<.001, P<.01). Mean retinal fluorescence was increased in cells treated with C2 ceramide compared to control cells and cells treated with C2 ceramide + dihydroceramide C2 (P<.05). | n/a | |
Retinal flavoprotein fluorescence correlates with mitochondrial stress, apoptosis, and chemokine expression | Field et al, 2011 | Cultured human retinal pigment epithelial cells and samples of human and rat retina ex vivo | Cells exposed to H2O2 with and without N-acetyl-cysteine OR cocultured with monocytes | Samples treated with higher H2O2 doses displayed significantly increased levels of realtive FPF intensity than did lower doses (P<.001) | Samples treated with increased H2O2 concentration also displayed decreased mitochondrial membrane potential (P<.001), upregulation of IL-8 (P<.001), increased apoptosis (P<.05). |
FLAVOPROTEIN FLUORESCENCE CLINICAL STUDIES
Nine peer reviewed papers report on retinal FPF imaging in human subjects (Table 2). Flavoprotein fluorescence is reported as intensity, a cumulative value reflecting global signal strength, and/or curve width or heterogeneity, which are measures of variation of the relative intensity of points in the image. Elner et al’s first study with human subjects compared the FPF intensity of 6 patients with newly diagnosed pseudotumor cerebri (PTC) to 6 age-matched healthy control patients. The study found that patients with PTC had significantly greater FPF intensity and curve width scores when compared to control patients, suggesting that FPF could be useful in detection of early optic nerve pathology.37
TITLE | AUTHOR, DATE | STUDY TYPE | NUMBER OF EYES | INTERVENTION | FPF INTENSITY | FPF HETEROGENEITY/CURVE WIDTH (CW) | ADDITIONAL RESULTS | |
Flavoprotein autofluorescence detection of early ocular dysfunction | Elner et al, 2008 | Case control | 12 eyes with pseudotumor cerebri (PTC), 12 control eyes | n/a | Average intensity of PTC patients 1.6 times greater than control (P<.01) | Average curve width of PTC patients was 1.62 times greater than control (P<.01). | PTC patients displayed higher FPF intensity and curve width scores than did control patients. | |
Rapid, noninvasive detection of diabetes-induced retinal metabolic stress | Field et al, 2008 | Case control | 42 eyes with diabetes mellitus (DM), 42 control eyes | n/a | Diabetic cases had significantly greater intensity than controls (P≤.004) | Diabetic cases had significantly greater CW than controls (P<.001). | Patients with retinopathy displayed higher intensity (P=.002) and curve width values (P=.005) than patients with DM but no retinopathy. | |
Retinal flavoprotein autofluorescence as a measure of retinal health | Elner et al, 2008 | Case control | 28 eyes with DM, 14 control eyes, 2 eyes with advanced age-related macular degeneration (AMD), 2 eyes with retinitis pigmentosa (RP), 2 eyes with central serous chorioretinopathy (CSCR) | n/a | Diabetic cases without retinopathy diaplayed increased average intensity compared to controls (59.5,36.1, P=.001). Diabetic cases with retinopathy diaplayed increased average intensity compared to diabetic cases without retinopathy (76.6, 59.5, P=.04). | Diabetic cases without retinopathy diaplayed increased average CW to controls (58.7,35.7, P=.001). Diabetic cases with retinopathy diaplayed increased average CW compared to diabetic cases without retinopathy (72.1, 58.7, P=.08). | Individual patients with AMD, RP, and CSCR also displayed increased FPF intensity and CW values compared to an age-matched control. | |
Detection of retinal metabolic stress resulting from central serous retinopathy | Field et al, 2009 | Case control | 3 eyes with CSCR, 3 fellow eyes from CSCR patients, 6 control eyes. | n/a | Three eyes with CSCR had increased intensity compared to control eyes (P<.05). Two of 3 affected CSCR eyes had significantly increased FPF intensity when compared to fellow eye (P<.001) | n/a | n/a | |
Noninvasive imaging of mitochondrial dysfunction in dry age-related macular degeneration | Field et al, 2012 | Case control | 3 eyes with non exudative AMD, 3 eyes with non exudative AMD and geographic atrophy (GA), 6 control eyes. | n/a | Average intensity of AMD patients without GA was significantly greater than that of control eyes (P=.044). Average intensity of AMD patients with GA was significantly greater than that of control eyes (P<.001) | FPF heterogeneity was increased in eyes with AMD compared to control eyes (P=.20). | n/a | |
Randomized, double-masked, placebo-controlled trial of the efficacy of a novel neuroprotective combination for reversing mitochondrial dysfunction in glaucoma | Ritch et al, 2018 | Randomized controlled trial | 28 eyes with glaucoma, treated with either placebo or antioxidant supplement. | antioxidant supplement | Mean decrese of 39.78 in experimental group (P=.003). Mean decrese of 15.54 in control group (P=.470) | Mean decrese of 25.07 in experimental group (P=.010); mean decrese of 11.90 in control group (P=.235) | n/a | |
Noninvasive detection of mitochondrial dysfunction in ocular hypertension and primary open-angle glaucoma | Geyman et al, 2018 | Case control | 16 eyes with ocular hypertension (OHTN), 40 eyes with glaucoma, 36 control eyes | n/a | OHTN patients displayed increased FPF intensity when compared to control patients (437, 327, P<.05). FPF intensity was not significantly elevated in glaucoma patients compared to controls (P=.24). | n/a | FPF/retina ganglion cell ratio was assessed: FPF/RGC ratio was significantly increased in OHTN and glaucoma patients (P<.01, P<.001) | |
Flavoprotein fluorescence correlation with visual acuity response in patients receiving anti-VEGF injection for diabetic macular edema | Andrade Romo et al, 2018 | Case control | 8 eyes with diabetic retinopathy and macular edema | anti-VEGF treatment | FPF intensity values decreased in 5 of 8 patients after treatment with anti-VEGF. | n/a | All eyes that showed improvements in BCVA also displayed decreases in FPF intensity. Significant correlation was found between FPF change and BCVA (r=0.98, P=.000015). | |
Functional imaging of mitochondria in retinal diseases using glavoprotein fluotescence | Chen et al, 2021 | Case control | 151 control eyes, 117 diabetic eyes | n/a | FPF intensity in diabetic cases was 5.1 units higher compared to control cases (P=.002). | FPF heterogeneity in diabetic cases was 0.15 units higher compared to control cases (P<.001). | In eyes with DM, increased FPF heterogeneity was associated with decreased visual acuity (P<.001). |
Two studies were published in 2008 examining FPF in diabetic patient populations. Field et al examined 21 patients with diabetes mellitus (DM) and compared their FPF values to those of healthy age-matched controls. Patients with DM had on average significantly greater intensity and curve width values than did the control patients.38 Around this time, Elner et al published a study that examined a cohort of 14 diabetic patients and 7 controls, also finding significant increases in intensity and curve width values when comparing diabetics to controls.35 Elner et al also examined patients with diabetic retinopathy (DR), and compared them to diabetic patients without retinopathy. Patients with DR were found to have increased intensity and curve width than did patients with diabetes alone.35
Work in DR demonstrated the real-world application of the principles that FPF might reflect oxidative stress. Multiple disease states sharing a common mechanism of oxidative stress and mitochondrial dysfunction were also then examined. Elner et al examined individual cases of patients with AMD, retinitis pigmentosa, and central serous chorioretinopathy (CSCR). These cases were identified to have increased FPF intensity and curve width values in each of these patient populations compared to a control patient.35 Field et al published a study in 2009 in which they examined 3 patients with CSCR, finding that these patients had increased FPF intensity compared to age-matched controls.39 These pilot studies highlighted that other disease states that share mitochondrial dysfunction and oxidative stress could be promising areas of investigation for years to come.
Field et al published a study in 2012 examining the relationship between FPF and stages of AMD. This study compared 6 control eyes to 3 eyes with nonexudative AMD, and 3 eyes with nonexudative AMD and geographic atrophy (GA). It was found that average FPF intensity was increased in AMD eyes compared to control eyes. Eyes with GA had higher heterogeneity than did AMD eyes without GA.40
Studies have also been performed assessing FPF in patients with glaucoma. Geyman et al studied a cohort of 40 glaucomatous patients and 15 ocular hypertensive patients. Increases in FPF intensity were noted in patients in ocular hypertension eyes compared to control eyes. Interestingly, patients with primary open angle glaucoma did not display increased FPF values when compared to controls, possibly due to increases in cell death in glaucomatous patients decreasing detectable FPF signal.41 This suggests that FPF could be useful in early detection of retinal damage before structural damage is seen on OCT imaging.
Flavoprotein fluorescence as a marker of oxidative stress in vivo across multiple disease states is a plausible technique. Most importantly, recent work has been conducted that highlights this tool as relevant to the practicing clinician by correlating FPF imaging with real world clinical outcomes. In 2018, Andrade Romo et al observed FPF values before and after treatment with anti-VEGF injection in 8 patients with proliferative diabetic retinopathy. It was found that decreased FPF significantly correlated with improvements in BCVA letters. This finding suggested that FPF imaging may be able to detect improvements in retinal metabolic function before changes are seen on OCT imaging.42
A randomized crossover trial conducted by Ritch et al observed a trial of an antioxidant supplement in 14 patients with glaucoma. It was found that participants taking the supplement experienced significant decreases in optic nerve FPF intensity and curve width after 1 month of supplementation, whereas the placebo arm did not experience such benefits.42 However, no further details were specified.
Most recently, in 2020, Chen et al examined the largest cohort reported to date, which included 268 eyes, of which 117 were diabetic, and 151 were healthy control eyes. It was found that FPF intensity and heterogeneity was elevated in diabetic eyes compared to age-matched control eyes (Figure 2).3 Higher FPF heterogeneity was also found to be associated with worsened visual acuity in these eyes. Independent predictors of FPF intensity that were found in this study included age and female gender, whereas patients’ age was also a predictor of heterogeneity.
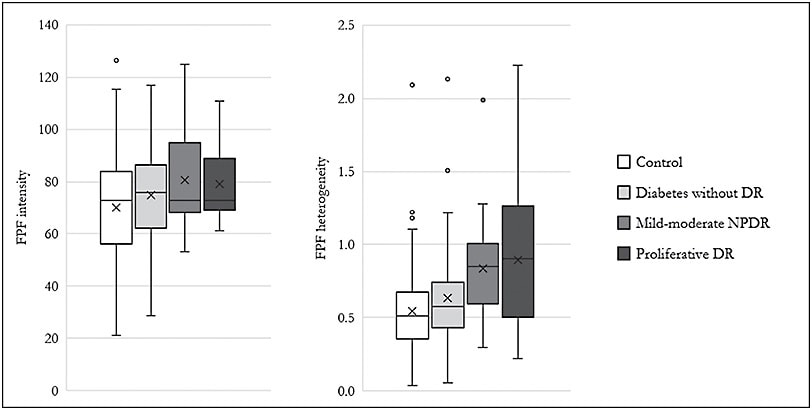
SUMMARY
The above studies have shown retinal FPF might be of utility in assessing ocular hypertension, glaucoma, DR, and AMD. However, many of the studies examined are hindered by their collective small sample size, which limits external validity, a lack of a normative database of controls, and a lack of standardized imaging methodology between studies. In the future, larger scale, longitudinal studies need to be performed to assess FPF imaging as a reliable measure of retinal health across various disease states and treatment modalities to further examine the utility of FPF imaging in routine clinical practice. RP
REFERENCES
- Steinmetz JD, Bourne RRA, Briant PS, et al. Causes of blindness and vision impairment in 2020 and trends over 30 years, and prevalence of avoidable blindness in relation to VISION 2020: the Right to Sight: an analysis for the Global Burden of Disease Study. The Lancet Global Health. 2021;9(2):e144-e160. doi:10.1016/S2214-109X(20)30489-7
- Gabriele ML, Wollstein G, Ishikawa H, et al. Optical coherence tomography: history, current status, and laboratory work. Invest Ophthalmol Vis Sci. 2011;52(5):2425-2436. doi:10.1167/iovs.10-6312
- Chen AX, Conti TF, Hom GL, et al. Functional imaging of mitochondria in retinal diseases using flavoprotein fluorescence. Eye. 2021;35(1):74-92. doi:10.1038/s41433-020-1110-y
- Barot M, Gokulgandhi MR, Mitra AK. Mitochondrial dysfunction in retinal diseases. Curr Eye Res. 2011;36(12):1069-1077. doi:10.3109/02713683.2011.607536
- Schrier SA, Falk MJ. Mitochondrial disorders and the eye. Curr Opin Ophthalmol. 2011;22(5):325-331. doi:10.1097/ICU.0b013e328349419d
- Chrysostomou V, Rezania F, Trounce IA, Crowston JG. Oxidative stress and mitochondrial dysfunction in glaucoma. Current Opinion in Pharmacology. 2013;13(1):12-15. doi:10.1016/j.coph.2012.09.008
- Wu MY, Yiang GT, Lai TT, Li CJ. The Oxidative stress and mitochondrial dysfunction during the pathogenesis of diabetic retinopathy. Oxid Med Cell Longev. 2018;2018:e3420187. doi:10.1155/2018/3420187
- Chance B, Schoener B, Oshino R, Itshak F, Nakase Y. Oxidation-reduction ratio studies of mitochondria in freeze-trapped samples. NADH and flavoprotein fluorescence signals. J Biol Chem. 1979;254(11):4764-4771.
- Benson RC, Meyer RA, Zaruba ME, McKhann GM. Cellular autofluorescence--is it due to flavins? J Histochem Cytochem. 1979;27(1):44-48. doi:10.1177/27.1.438504
- Missiroli S, Genovese I, Perrone M, Vezzani B, Vitto VAM, Giorgi C. The role of mitochondria in inflammation: from cancer to neurodegenerative disorders. J Clin Med. 2020;9(3):740. doi:10.3390/jcm9030740
- Bhatti JS, Bhatti GK, Reddy PH. Mitochondrial dysfunction and oxidative stress in metabolic disorders — A step towards mitochondria based therapeutic strategies. Biochim Biophys Acta Mol Basis Dis. 2017;1863(5):1066-1077. doi:10.1016/j.bbadis.2016.11.010
- Guo C, Sun L, Chen X, Zhang D. Oxidative stress, mitochondrial damage and neurodegenerative diseases. Neural Regen Res. 2013;8(21):2003-2014. doi:10.3969/j.issn.1673-5374.2013.21.009
- Zorov DB, Juhaszova M, Sollott SJ. Mitochondrial reactive oxygen species (ROS) and ROS-induced ROS release. Physiol Rev. 2014;94(3):909-950. doi:10.1152/physrev.00026.2013
- Su LJ, Zhang JH, Gomez H, et al. Reactive oxygen species-induced lipid peroxidation in apoptosis, autophagy, and ferroptosis. Oxid Med Cell Longev. 2019;2019:e5080843. doi:10.1155/2019/5080843
- Eells JT. Mitochondrial dysfunction in the aging retina. Biology (Basel). 2019;8(2):31. doi:10.3390/biology8020031
- Brown EE, DeWeerd AJ, Ildefonso CJ, Lewin AS, Ash JD. Mitochondrial oxidative stress in the retinal pigment epithelium (RPE) led to metabolic dysfunction in both the RPE and retinal photoreceptors. Redox Biol. 2019;24:101201. doi:10.1016/j.redox.2019.101201
- Kim HU, Park SP, Kim YK. Long-term HbA1c variability and the development and progression of diabetic retinopathy in subjects with type 2 diabetes. Sci Rep. 2021;11(1):4731. doi:10.1038/s41598-021-84150-8
- Kowluru RA, Mishra M. Oxidative stress, mitochondrial damage and diabetic retinopathy. Biochim Biophys Acta. 2015;1852(11):2474-2483. doi:10.1016/j.bbadis.2015.08.001
- Du Y, Miller CM, Kern TS. Hyperglycemia increases mitochondrial superoxide in retina and retinal cells. Free Radic Biol Med. 2003;35(11):1491-1499. doi:10.1016/j.freeradbiomed.2003.08.018
- Trudeau K, Molina AJA, Guo W, Roy S. High glucose disrupts mitochondrial morphology in retinal endothelial cells: implications for diabetic retinopathy. Am J Pathol. 2010;177(1):447-455. doi:10.2353/ajpath.2010.091029
- Li X, Zhang M, Zhou H. The morphological features and mitochondrial oxidative stress mechanism of the retinal neurons apoptosis in early diabetic rats. J Diabetes Res. 2014;2014:678123. doi:10.1155/2014/678123
- Kanwar M, Chan PS, Kern TS, Kowluru RA. Oxidative damage in the retinal mitochondria of diabetic mice: possible protection by superoxide dismutase. Invest Ophthalmol Vis Sci. 2007;48(8):3805-3811. doi:10.1167/iovs.06-1280
- Bellezza I. Oxidative stress in age-related macular degeneration: nrf2 as therapeutic target. Front Pharmacol. 2018;9:1280. doi:10.3389/fphar.2018.01280
- Lin H, Xu H, Liang FQ, et al. Mitochondrial DNA damage and repair in RPE associated with aging and age-related macular degeneration. Invest Ophthalmol Vis Sci. 2011;52(6):3521-3529. doi:10.1167/iovs.10-6163
- Jones MM, Manwaring N, Wang JJ, Rochtchina E, Mitchell P, Sue CM. Mitochondrial DNA haplogroups and age-related maculopathy. Arch Ophthalmol. 2007;125(9):1235-1240. doi:10.1001/archopht.125.9.1235
- Feher J, Kovacs I, Artico M, Cavallotti C, Papale A, Balacco Gabrieli C. Mitochondrial alterations of retinal pigment epithelium in age-related macular degeneration. Neurobiol Aging. 2006;27(7):983-993. doi:10.1016/j.neurobiolaging.2005.05.012
- Jarrett SG, Boulton ME. Consequences of oxidative stress in age-related macular degeneration. Mol Aspects Med. 2012;33(4):399-417. doi:10.1016/j.mam.2012.03.009
- Age-Related Eye Disease Study Research Group. A randomized, placebo-controlled, clinical trial of high-dose supplementation with vitamins C and E, beta carotene, and zinc for age-related macular degeneration and vision loss: AREDS report no. 8 [published correction appears in Arch Ophthalmol. 2008 Sep;126(9):1251]. Arch Ophthalmol. 2001;119(10):1417-1436. doi:10.1001/archopht.119.10.1417
- Ju WK, Liu Q, Kim KY, et al. Elevated hydrostatic pressure triggers mitochondrial fission and decreases cellular ATP in differentiated RGC-5 cells. Invest Ophthalmol Vis Sci. 2007;48(5):2145-2151. doi:10.1167/iovs.06-0573
- Ko ML, Peng PH, Hsu SY, Chen CF. Dietary deficiency of vitamin e aggravates retinal ganglion cell death in experimental glaucoma of rats. Curr Eye Res. 2010;35(9):842-849. doi:10.3109/02713683.2010.489728
- Garcia-Medina JJ, Rubio-Velazquez E, Lopez-Bernal MD, et al. Glaucoma and antioxidants: review and update. Antioxidants (Basel). 2020;9(11):1031. doi:10.3390/antiox9111031
- Turner NJ. 7.12 Oxidation: Oxidases. In: Carreira EM, Yamamoto H, eds. Comprehensive Chirality. Elsevier; 2012:256-274. doi:10.1016/B978-0-08-095167-6.00715-1
- Kuznetsov AV, Mayboroda O, Kunz D, Winkler K, Schubert W, Kunz WS. Functional imaging of mitochondria in saponin-permeabilized mice muscle fibers. J Cell Biol. 1998;140(5):1091-1099. doi:10.1083/jcb.140.5.1091
- Sparrow JR, Duncker T. Fundus autofluorescence and RPE lipofuscin in age-related macular degeneration. J Clin Med. 2014;3(4):1302-1321. doi:10.3390/jcm3041302
- Elner SG, Elner VM, Field MG, Park S, Heckenlively JR, Petty HR. Retinal flavoprotein autofluorescence as a measure of retinal health. Trans Am Ophthalmol Soc. 2008;106:215-222; discussion 222-224.
- Field MG, Yang D, Bian ZM, Petty HR, Elner VM. Retinal flavoprotein fluorescence correlates with mitochondrial stress, apoptosis, and chemokine expression. Exp Eye Res. 2011;93(4):548-555. doi:10.1016/j.exer.2011.06.023
- Elner VM, Park S, Cornblath W, Hackel R, Petty HR. Flavoprotein autofluorescence detection of early ocular dysfunction. Arch Ophthalmol. 2008;126(2):259-260. doi:10.1001/archophthalmol.2007.44
- Field MG, Elner VM, Puro DG, et al. Rapid, noninvasive detection of diabetes-induced retinal metabolic stress. Arch Ophthalmol. 2008;126(7):934-938. doi:10.1001/archopht.126.7.934
- Field MG, Elner VM, Park S, et al. Detection of retinal metabolic stress due to central serous retinopathy. Retina. 2009;29(8):1162-1166. doi:10.1097/IAE.0b013e3181a3b923
- Field Matthew G., Comer Grant M., Kawaji Takahiro, Petty Howard R., Elner Victor M. Noninvasive imaging of mitochondrial dysfunction in dry age-related macular degeneration. Ophthalmic Surg Lasers Imaging. 2012;43(5):358-365. doi:10.3928/15428877-20120712-02
- Geyman LS, Suwan Y, Garg R, et al. Noninvasive detection of mitochondrial dysfunction in ocular hypertension and primary open-angle glaucoma. J Glaucoma. 2018;27(7):592-599. doi:10.1097/IJG.0000000000000980
- Andrade Romo JS, Lynch G, Liu K, et al. Flavoprotein fluorescence correlation with visual acuity response in patients receiving anti-VEGF injection for diabetic macular edema. Oxid Med Cell Longev. 2018;2018:e3567306. doi:10.1155/2018/3567306