Imaging modalities have long been integral to ophthalmology in clinical and research settings. In 1991, the introduction of optical coherence tomography (OCT) became a pivotal milestone in noninvasive imaging of the retina and optic nerve in vivo.1 Its utility has been critical in both studying and managing pathology throughout nearly all layers of the anterior2-6 and posterior7-10 segments. In 2006, another milestone was achieved in ocular imaging as Makita et al described optical coherence tomography angiography (OCTA), which built off of the OCT platform and thus provided a new modality to perform noninvasive angiography of the eye with macular images comparable to dye-based angiography (Figures 1 and 2).11 Optical coherence tomography angiography is a functional extension of OCT technology to visualize the retinal and choroidal vasculature. The end result is a full-thickness retinal angiogram with images segmented into the superficial retinal plexus, deep retinal plexus, outer retina, and choriocapillaris.12 It is not only fast (acquiring 3-dimensional scans in a matter of seconds),13 but also, in being noninvasive, it avoids the rare but potentially serious adverse reactions ranging from nausea to anaphylaxis associated with dyes used in angiography, such as fluorescein or indocyanine green.14-16
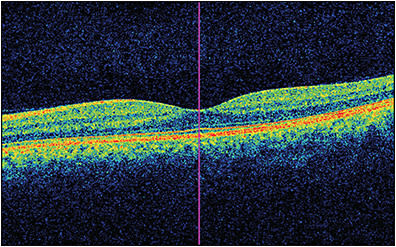
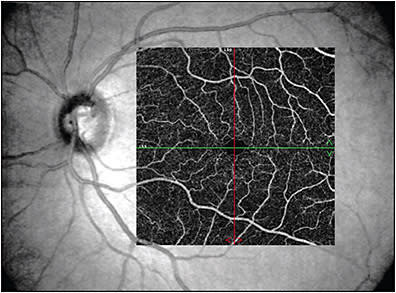
A number of studies have investigated the role of OCT and OCTA in primary retinal diseases such as diabetic retinopathy, macular degeneration, and retinal vascular occlusions.17-23 However, recently, there has been growing interest in the role of these imaging technologies in neurodegenerative diseases based on some exciting initial studies.24
Alzheimer disease (AD) is the most common cause of dementia, most notably characterized by progressive cognitive impairment leading to memory decline and deficits in learning and executive functioning, and its incidence continues to increase worldwide.25 Because the retina is a peripheral extension of the central nervous system, it is believed that significant homology may exist between the changes that affect both the brain and retina in AD. Accordingly, OCT and OCTA studies may serve as valuable tools in better understanding these processes.
OPTICAL COHERENCE TOMOGRAPHY AND ALZHEIMER DISEASE
Optical coherence tomography has become a widely used technology to quantitatively evaluate structural axonal damage in optic nerve and neurologic processes. This is done through OCT measurement of peripapillary retinal nerve fiber layer (RNFL) loss to estimate retinal ganglion cell (RGC) impairment along with OCT measurements of macular thickness, because the RGC layer and its axons contribute a significant portion of normal total retinal thickness. In terms of RNFL thickness, numerous studies have shown that most RNFL parameters were reduced in patients with AD, especially those with cognitive impairment.26-29 Although there is some variation in which and how many quadrants are reduced, there has been consistency in the results, even across different OCT machines, that show a degree of RNFL thinning associated with AD patients when compared to healthy, nondiseased controls.
In terms of total macular thickness, there are similar results as well, although fewer studies have analyzed this specific finding. In 2006, Iseri et al was the first group to show that retinal thickness in all macular quadrants along with mean total macular volume was lower in AD patients than in control subjects.28 This has been corroborated as well by multiple other studies using different OCT machines.26,27,30
One clinically relevant area of study has included correlating RNFL and macular thickness measurements with cognitive impairment based on neuropsychologic testing with Mini-Mental State Examination (MMSE) scores. Findings in these studies have been somewhat mixed. Nonetheless, a few studies notably by Iseri et al and Ascaso et al have shown a significant correlation between OCT parameters (including RNFL and macular thickness parameters) and MMSE scores in AD patients.28,31
OPTICAL COHERENCE TOMOGRAPHY ANGIOGRAPHY AND ALZHEIMER DISEASE
OCTA has been useful in identifying changes in retinal capillary densities in those with known AD. Bulut et al32 discovered that when comparing the retinal and choroidal vasculature of individuals with known AD, the vascular density was significantly lower in all zones of the superficial vascular plexus (SRCP) in those with AD, a result which was also seen in a similar study by Lahme et al.33 Additionally, Yoon et al demonstrated that this finding existed even when comparing patients with AD to those with mild cognitive impairment and that there was no significant relationship when comparing those with mild cognitive impairment to cognitively intact controls.34 Although Wu et al only corroborated the SRCP differences in 1 quadrant, they showed that the deep retinal capillary plexus (DRCP) microvascular density at all quadrants was significantly lower in patients with AD compared to healthy controls.35 Jiang et al demonstrated significant differences in both SRCP and DRCP densities in AD patients compared to healthy controls.36 Interestingly, Bulut et al also found a significant increase in the foveal avascular zone (FAZ) — the region within the fovea devoid of retinal blood vessels in those with AD. Additionally, they discovered a significant correlation between the SRCP density and FAZ with the MMSE score.32
Although these results were suggestive of retinal vascular alterations in known AD patients, none of the aforementioned studies involved patients with preclinical AD (ie, biomarker-positive and cognitively intact). Two studies thus far have had differing results when utilizing OCTA to detect preclinical AD in such individuals. O’Bryhim et al studied 14 individuals who were biomarker positive by PET and/or CSF Aβ42 protein level with clinical dementia rating score of 0 (ie, no evidence of dementia on neuropsychometric testing) and found a statistically significant increase in the FAZ compared to those individuals without preclinical AD, suggesting a possible role of FAZ enlargement in identifying preclinical AD.37 Furthermore, in a 3-year follow up study, they found a stably enlarged FAZ.38 Nonetheless, it should be noted that although O’Bryhim et al excluded those with diabetes and uncontrolled hypertension, they acknowledged the potential confounding of FAZ enlargement from other causes and the lack of specificity in this finding.37 Additionally, another similar study of 13 cognitively healthy, biomarker positive (by PET) individuals conversely found no difference in FAZ size and instead found a statistically significant increase in vessel density in those with preclinical AD. The authors attributed this increase in vessel density to an initial inflammatory state of the retina in the setting of early amyloid accumulation, resulting in hypoxia causing an increase in the number of microvessels detected by OCTA.39 The differing results may be due to the specific population of participants studies or other reasons; however, these findings suggest a relationship between altered retinal microvasculature in preclinical AD.
Alzheimer disease pathophysiology has largely been connected to the accumulation of beta-amyloid peptides and hyperphosphorylated tau proteins leading to amyloid plaque formation and neurofibrillary tangles.40 It has been suggested that retinal deposition of amyloid beta plays a mechanistic role for RGC degeneration in AD. From a microvascular standpoint, a commonly discussed theory is that these vascular changes in AD are the result of beta-amyloid deposition and plaque formation throughout the microvasculature of the retina. Accordingly, there is resultant decreased blood flow along with trapping of vascular endothelial growth factor causing decreased angiogenesis. Not only has this process been studied in the cerebral circulation of patients with known AD,41-44 but also it has also been explored in studies of retinal vasculature as well.45,46 From our review, there is still no temporal relationship that exists to suggest whether retinal microvascular changes precede cerebral microvascular changes. Nonetheless, OCT and OCTA biomarkers may have a role in diagnosis and management of AD, although more studies are needed to further elucidate their relationship. Future studies will explore which retinal findings may be more appropriate as biomarkers of disease onset and progression and whether they can be used to evaluate therapeutic response. RP
REFERENCES
- Huang D, Swanson EA, Lin CP, et al. Optical coherence tomography. Science. 1991;254(5035):1178-1181. doi:10.1126/science.1957169
- Han SB, Liu YC, Noriega KM, Mehta JS. Applications of anterior segment optical coherence tomography in cornea and ocular surface diseases. J Ophthalmol. 2016;2016:4971572. doi:10.1155/2016/4971572
- Lim SH. Clinical applications of anterior segment optical coherence tomography. J Ophthalmol. 2015;2015:605729. doi:10.1155/2015/605729
- Sridhar MS, Martin R. Anterior segment optical coherence tomography for evaluation of cornea and ocular surface. Indian J Ophthalmol. 2018;66(3):367-372. doi:10.4103/ijo.IJO_823_17
- Venkateswaran N, Galor A, Wang J, Karp CL. Optical coherence tomography for ocular surface and corneal diseases: a review. Eye Vis (Lond). 2018;5:13. Published 2018 Jun 12. doi:10.1186/s40662-018-0107-0
- Wang SB, Cornish EE, Grigg JR, McCluskey PJ. Anterior segment optical coherence tomography and its clinical applications. Clin Exp Optom. 2019;102(3):195-207. doi:10.1111/cxo.12869
- Dong ZM, Wollstein G, Schuman JS. Clinical utility of optical coherence tomography in glaucoma. Invest Ophthalmol Vis Sci. 2016;57(9):OCT556-OCT567. doi:10.1167/iovs.16-19933
- Medeiros FA, Zangwill LM, Alencar LM, et al. Detection of glaucoma progression with stratus OCT retinal nerve fiber layer, optic nerve head, and macular thickness measurements. Invest Ophthalmol Vis Sci. 2009;50(12):5741-5748. doi:10.1167/iovs.09-3715
- Murthy RK, Haji S, Sambhav K, Grover S, Chalam KV. Clinical applications of spectral domain optical coherence tomography in retinal diseases. Biomed J. 2016;39(2):107-120. doi:10.1016/j.bj.2016.04.003
- Rosenfeld PJ. Optical coherence tomography and the development of antiangiogenic therapies in neovascular age-related macular degeneration. Invest Ophthalmol Vis Sci. 2016;57(9):OCT14-OCT26. doi:10.1167/iovs.16-19969
- Makita S, Hong Y, Yamanari M, Yatagai T, Yasuno Y. Optical coherence angiography. Opt Express. 2006;14(17):7821-7840. doi:10.1364/oe.14.007821
- de Carlo TE, Romano A, Waheed NK, Duker JS. A review of optical coherence tomography angiography (OCTA). Int J Retina Vitreous. 2015;1:5.
- Matsunaga D, Yi J, Puliafito CA, Kashani AH. OCT angiography in healthy human subjects. Ophthalmic Surg Lasers Imaging Retina. 2014;45(6):510-515. doi:10.3928/23258160-20141118-04
- Kornblau IS, El-Annan JF. Adverse reactions to fluorescein angiography: a comprehensive review of the literature. Surv Ophthalmol. 2019;64(5):679-693. doi:10.1016/j.survophthal.2019.02.004
- Meira J, Marques ML, Falcão-Reis F, Rebelo Gomes E, Carneiro Â. Immediate reactions to fluorescein and indocyanine green in retinal angiography: review of literature and proposal for patient’s evaluation. Clin Ophthalmol. 2020;14:171-178. Published 2020 Jan 20. doi:10.2147/OPTH.S234858
- Obana A, Miki T, Hayashi K, et al. Survey of complications of indocyanine green angiography in Japan. Am J Ophthalmol. 1994;118(6):749-753. doi:10.1016/s0002-9394(14)72554-1
- Bandello F, Corbelli E, Carnevali A, Pierro L, Querques G. Optical coherence tomography angiography of diabetic retinopathy. Dev Ophthalmol. 2016;56:107-112. doi:10.1159/000442801
- Bonini Filho MA, Adhi M, de Carlo TE, et al. Optical coherence tomography angiography in retinal artery occlusion. Retina. 2015;35(11):2339-2346. doi:10.1097/IAE.0000000000000850
- Choi W, Moult EM, Waheed NK, et al. Ultrahigh-speed, swept-source optical coherence tomography angiography in nonexudative age-related macular degeneration with geographic atrophy. Ophthalmology. 2015;122(12):2532-2544. doi:10.1016/j.ophtha.2015.08.029
- Choi W, Waheed NK, Moult EM, et al. Ultrahigh speed swept source optical coherence tomography angiography of retinal and choriocapillaris alterations in diabetic patients with and without retinopathy. Retina. 2017;37(1):11-21. doi:10.1097/IAE.0000000000001250
- Ghasemi Falavarjani K, Iafe NA, Hubschman JP, Tsui I, Sadda SR, Sarraf D. Optical coherence tomography angiography analysis of the foveal avascular zone and macular vessel density after anti-VEGF therapy in eyes with diabetic macular edema and retinal vein occlusion. Invest Ophthalmol Vis Sci. 2017;58(1):30-34. doi:10.1167/iovs.16-20579
- Salz DA, de Carlo TE, Adhi M, et al. Select features of diabetic retinopathy on swept-source optical coherence tomographic angiography compared with fluorescein angiography and normal eyes. JAMA Ophthalmol. 2016;134(6):644-650. doi:10.1001/jamaophthalmol.2016.0600
- Tsuboi K, Ishida Y, Kamei M. Gap in Capillary Perfusion on Optical Coherence Tomography Angiography Associated With Persistent Macular Edema in Branch Retinal Vein Occlusion. Invest Ophthalmol Vis Sci. 2017;58(4):2038-2043. doi:10.1167/iovs.17-21447
- Shah A, Apte RS. Optical coherence tomography angiography: a window into central nervous system neurodegeneration. Trends Mol Med. 2020;26(10):892-895. doi:10.1016/j.molmed.2020.08.003
- Blennow K, de Leon MJ, Zetterberg H. Alzheimer’s disease. Lancet. 2006;368(9533):387-403. doi:10.1016/S0140-6736(06)69113-7
- Cunha LP, Lopes LC, Costa-Cunha LV, et al. Macular thickness measurements with frequency domain-oct for quantification of retinal neural loss and its correlation with cognitive impairment in Alzheimer’s disease. PLoS One. 2016;11(4):e0153830. doi:10.1371/journal.pone.0153830
- Gao L, Liu Y, Li X, Bai Q, Liu P. Abnormal retinal nerve fiber layer thickness and macula lutea in patients with mild cognitive impairment and Alzheimer’s disease. Arch Gerontol Geriatr. 2015;60(1):162-167. doi:10.1016/j.archger.2014.10.011
- Iseri PK, Altinaş O, Tokay T, Yüksel N. Relationship between cognitive impairment and retinal morphological and visual functional abnormalities in Alzheimer disease. J Neuroophthalmol. 2006;26(1):18-24. doi:10.1097/01.wno.0000204645.56873.26
- Kromer R, Serbecic N, Hausner L, Froelich L, Aboul-Enein F, Beutelspacher SC. Detection of retinal nerve fiber layer defects in Alzheimer’s disease using SD-OCT. Front Psychiatry. 2014;5:22. doi:10.3389/fpsyt.2014.00022
- Polo V, Garcia-Martin E, Bambo MP, et al. Reliability and validity of Cirrus and Spectralis optical coherence tomography for detecting retinal atrophy in Alzheimer’s disease. Eye (Lond). 2014;28(6):680-690. doi:10.1038/eye.2014.51
- Ascaso FJ, Cruz N, Modrego PJ, et al. Retinal alterations in mild cognitive impairment and Alzheimer’s disease: an optical coherence tomography study. J Neurol. 2014;261(8):1522-1530. doi:10.1007/s00415-014-7374-z
- Bulut M, Kurtuluş F, Gözkaya O, et al. Evaluation of optical coherence tomography angiographic findings in Alzheimer’s type dementia. Br J Ophthalmol. 2018;102(2):233-237. doi:10.1136/bjophthalmol-2017-310476
- Lahme L, Esser EL, Mihailovic N, et al. Evaluation of ocular perfusion in Alzheimer’s disease using optical coherence tomography angiography. J Alzheimers Dis. 2018;66(4):1745-1752. doi:10.3233/JAD-180738
- Yoon SP, Grewal DS, Thompson AC, et al. Retinal microvascular and neurodegenerative changes in Alzheimer’s disease and mild cognitive impairment compared with control participants. Ophthalmol Retina. 2019;3(6):489-499. doi:10.1016/j.oret.2019.02.002
- Wu J, Zhang X, Azhati G, Li T, Xu G, Liu F. Retinal microvascular attenuation in mental cognitive impairment and Alzheimer’s disease by optical coherence tomography angiography. Acta Ophthalmol. 2020;98(6):e781-e787. doi:10.1111/aos.14381
- Jiang H, Wei Y, Shi Y, et al. Altered macular microvasculature in mild cognitive impairment and Alzheimer disease. J Neuroophthalmol. 2018;38(3):292-298. doi:10.1097/WNO.0000000000000580
- O’Bryhim BE, Apte RS, Kung N, Coble D, Van Stavern GP. Association of preclinical Alzheimer disease with optical coherence tomographic angiography findings. JAMA Ophthalmol. 2018;136(11):1242-1248. doi:10.1001/jamaophthalmol.2018.3556
- O’Bryhim BE, Lin JB, Van Stavern GP, Apte RS. OCT Angiography findings in preclinical Alzheimer’s disease: 3-year follow-up. Ophthalmology. 2021;S0161-6420(21)00128-7. doi:10.1016/j.ophtha.2021.02.016
- van de Kreeke JA, Nguyen HT, Konijnenberg E, et al. Optical coherence tomography angiography in preclinical Alzheimer’s disease. Br J Ophthalmol. 2020;104(2):157-161. doi:10.1136/bjophthalmol-2019-314127
- Karran E, Mercken M, De Strooper B. The amyloid cascade hypothesis for Alzheimer’s disease: an appraisal for the development of therapeutics. Nat Rev Drug Discov. 2011;10(9):698-712. Published 2011 Aug 19. doi:10.1038/nrd3505
- Brown WR, Thore CR. Review: cerebral microvascular pathology in ageing and neurodegeneration. Neuropathol Appl Neurobiol. 2011;37(1):56-74. doi:10.1111/j.1365-2990.2010.01139.x
- Hunter JM, Kwan J, Malek-Ahmadi M, et al. Morphological and pathological evolution of the brain microcirculation in aging and Alzheimer’s disease. PLoS One. 2012;7(5):e36893. doi:10.1371/journal.pone.0036893
- Dorr A, Sahota B, Chinta LV, et al. Amyloid-β-dependent compromise of microvascular structure and function in a model of Alzheimer’s disease. Brain. 2012;135(Pt 10):3039-3050. doi:10.1093/brain/aws243
- Zlokovic BV. Neurovascular pathways to neurodegeneration in Alzheimer’s disease and other disorders. Nat Rev Neurosci. 2011;12(12):723-738. Published 2011 Nov 3. doi:10.1038/nrn3114
- Cheung CY, Ong YT, Ikram MK, et al. Microvascular network alterations in the retina of patients with Alzheimer’s disease. Alzheimers Dement. 2014;10(2):135-142. doi:10.1016/j.jalz.2013.06.009
- Koronyo Y, Biggs D, Barron E, et al. Retinal amyloid pathology and proof-of-concept imaging trial in Alzheimer’s disease. JCI Insight. 2017;2(16):e93621. doi:10.1172/jci.insight.93621