Optical coherence tomography (OCT) is a quick and noninvasive imaging modality capable of discerning high-resolution morphologic features of the retina and surrounding structures.1 Over the past 20 years, OCT has evolved to become an integral component of ophthalmic diagnosis, management, and therapeutic monitoring, with an estimated tens of millions of images captured per year.2 During this time, improvements in OCT technology have enabled the transition from an outpatient imaging modality to the operating room.
Integration of OCT into the operating room was initially limited by the bulky equipment and design of clinical systems that required patients to be cooperative and positioned upright. This hurdle was overcome through the development of the handheld OCT, introduced to the surgical suite in 2009, which allowed imaging of patients who were supine and under anesthesia.3 This system was further updated to a microscope-mounted OCT configuration, which served to ameliorate previous critical limitations in scan reproducibility and precision. The use of these microscope-mounted external intraoperative OCT (iOCT) systems enabled extensive study and clinical evaluation of iOCT during vitreoretinal surgery, including study of the potential positive impact on surgical decision-making, with key findings from the PIONEER study highlighting the potential role in confirming achievement of surgical objectives and guiding decisions regarding need for tamponade choice or restaining.4-6
However, it was not until the advent of microscope-integrated OCT systems that widespread utilization became feasible.7,8 Following their initial development, the next major milestone was the FDA clearance of the first microscope-integrated system,9 which provided broad availability of efficient, real-time visualization of surgical manipulations and the cross-sectional surgical anatomy.10-14 Current commercially available iOCT options include the Leica Envisu handheld system and Enfocus microscope-integrated system, the Zeiss Rescan 700, and the Haag-Streit Surgical iOCT system. This review will focus on recent advancements in iOCT technology and its current utility in retinal surgery.
INNOVATION IN THE FIELD
Novel Visualization of the Surgical Field and OCT Datastream
There has been significant advancement from the conventional microscope-integrated systems that first made the commercialization and adaptation of iOCT to a wider audience possible.14-16 One key initial advance of iOCT was the injection of the OCT datastream into the microscope ocular that enabled simultaneous visualization of the surgical field and OCT imaging.13,15 Although a major innovative improvement, limitations to image quality and contrast with current systems have often resulted in the use of external monitors for OCT review.12,17
Emerging technological evolution in surgical microscopes and the surgical theater has brought new digitally enabled options for high-definition, 3D stereoscopic visualization during posterior-segment surgery. The integration of iOCT with these digitally enabled viewing systems frees the surgeon from the confines of the microscope oculars and allows for simultaneous viewing of the surgical field and OCT datastream on a heads-up display.17-20 These microscope systems, combined with next-generation OCT engines, have even allowed for real-time 3D OCT visualization, which has been termed 4D MIOCT.21
With certain systems, datafusion software allows for additional overlay of surgeon-chosen functions onto the 4K display screen. This integration allows real-time surgical parameters (eg, flow rates, infusion pressure, laser power) to be visualized on the active surgical image without blocking the surgeon’s view of the field. Surgical efficiency, ergonomics, patient safety, and new surgeon education in vitreoretinal surgery may be enhanced with this technology, but the literature in this area remains in its infancy.22-25
Currently, two 3D digital surgical visualization systems with capacity for iOCT integration are commercially available: Ngenuity (Alcon) combined with a microscope-integrated OCT system (Rescan 700; Zeiss) and the Artevo 800 (Zeiss) combined with the Rescan 700. One of the largest prospective studies to date comparing a digitally enabled iOCT surgical platform (Ngenuity with Rescan 700) with a conventional microscope-integrated iOCT platform (Rescan 700) demonstrated several advantages of the digital display system.17 Simultaneous surgical field-based visualization iOCT datastream was significantly higher in the digitally enabled cases compared to conventional. Importantly, the feasibility and utility of the heads-up, digitally enabled iOCT platform was similar to the conventional iOCT system.17 Similar results were also found when evaluating a newer digitally enabled iOCT system (Artevo 800 with Rescan 700).20 Whether this provides increased surgical safety by maintaining focus on the surgical field at all times remains to be elucidated, and future studies are under way.
Potential benefits of digital systems extend beyond intraoperative visualization. The ergonomic advantage of a 3D display system has been demonstrated in a few studies, with noted reduction in fatigue, back pain, and headaches.8,10-12 Another potential benefit is the amount of light necessary for a given case. In a recent study, surgeons who performed vitreoretinal surgical intervention with the digitally enabled platforms needed significantly less endoillumination throughout the case.13 Theoretical benefits to this exist, but future studies are needed to determine if there is translatable patient benefit.
Compatible Tools for Real-Time iOCT Utilization
Limitations in iOCT visualization during posterior-segment surgery exist in part due to a lag in compatible surgical instruments. Standard metallic instrumentation causes shadowing and light scattering that limits use and image acquisition during real-time surgical maneuvers. These limitations are likely demonstrated in studies that have shown that most surgeons preferred to pause surgery momentarily to utilize iOCT in a static fashion with only 37% utilizing iOCT in real-time during intrasurgical maneuvers.12 Instruments with semitransparent materials reduce light-scattering and improve viewing of the instrument tips and tissue.15 The development of iOCT-compatible instrument tips may provide greater utility during surgical manipulation of posterior-segment structures. In cadaver eyes, these instruments, which included forceps, membrane scrapers, and a surgical pick, demonstrated better iOCT visualization.26
Another limitation in real-time surgical application of iOCT is the fast frame rate, high sampling density, and image stabilization required to produce the highest quality image. To achieve this, current technology necessitates a small field of view that may hinder the performance of certain surgical maneuvers. Instrument tracking may solve this issue by automatically recentering the OCT scan in real time on the tip of the instrument. Current iterations of microscope-integrated iOCT platforms have a reference arm tracking that improves image stabilization.14 Research into improved methods for tracking is ongoing and includes using microscopic tip tracking and prediction via machine-learning algorithms10 and instrument handle tracking using stereo cameras.27
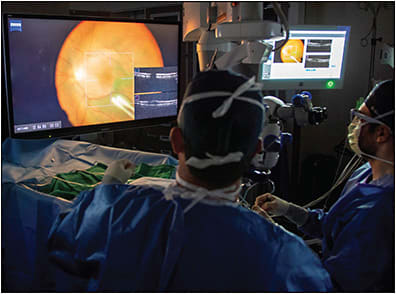
Next-generation OCT Engines and Software Platforms
Another highly impactful area of research that may propel the flexibility and utility of this technology forward is the integration of high-speed systems that provide volumetric real-time OCT capability. Currently, spectral-domain systems are limited to B-scans of variable orientation, which does not allow for live volumetric imaging. The development of new graphics-processing technologies and microscope-integrated high-speed swept-source iOCT systems can produce live volumetric 4D imaging in real time.21,28
Advanced image analysis platforms are greatly lacking in all commercial iOCT systems. New work in retinal segmentation and pathology feature extraction, including volumetric analysis of fluid and macular holes, provides new opportunities for understanding surgical pathology and the impact of surgical maneuvers.15,29,30 In combination with next-generation precision medicine, this technology may help guide surgical decision-making (eg, confirming optimal subretinal dose delivery or predicting macular hole closure time).31,32 iOCT volumetric analysis has already been shown to be useful in macular hole surgery15 and may prove to be critical in newer procedures aimed at delivering therapies into the subretinal space for gene therapy, stem cell therapy, and pharmacotherapy.32-35 Intraoperative imaging has also identified quantitative alterations in EZ-RPE height and subretinal hyporeflectivity following membrane peeling that are associated with foveal normalization speed, possibly suggesting a surrogate between these variables and intrinsic tissue characteristics.36 Quantification of these alterations may be translated into prediction models for postoperative behavior.31
UTILITY OF IOCT IN THE OPERATING ROOM
Membrane Peeling and Epiretinal Membranes
Over the past 20 years, vital dyes such as indocyanine green and brilliant blue G have played a critical role in identifying the internal limiting membrane (ILM) to facilitate membrane peeling in a variety of vitreoretinal disorders. However, these stains, especially indocyanine green, have phototoxic potential at high concentrations and may increase procedure length.37 Intraoperative OCT has been shown to be a feasible alternative to dye, producing comparable clinical outcomes by providing noninvasive, granular visualization of the retinal surface. Key areas of clinical utility include identifying an area to initiate the peel, recognizing areas of residual membrane that require further focus, and confirming completion of the peel.5,6 The PIONEER trial, a prospective multisurgeon study examining the feasibility of iOCT, demonstrated that intraoperative imaging findings prevented unnecessary surgical maneuvers in 9.2% of cases while identifying residual areas of peel in 12% of epiretinal membrane (ERM) surgeries.5
Previous studies have demonstrated that visualization of ERM intraoperatively is possible in both normal and highly myopic eyes.38,39 The DISCOVER trial built on this foundation to determine the feasibility of iOCT-guided ERM surgery. Compared to the conventional treatment option of vitrectomy with ILM peeling, iOCT-guided ERM removal without mandated ILM peeling resulted in no significant difference in surgical or visual outcomes, including reoperations over 12 months.40
Intraoperative OCT has also been utilized in outcomes research as a way of identifying potential risk factors for intraretinal architectural changes after ERM surgery. Leisser et al investigated subfoveal and extrafoveal hyporeflective zones on iOCT during membrane peel, reporting that these findings were not associated with postoperative OCT changes or visual outcomes.41 The same group also concluded that intraoperative characteristics were not associated with postoperative intraretinal cystoid changes.42 Similar results were noted in the PIONEER study where intraoperative expansion of the subretinal hyporeflective zones was not clearly linked to postoperative OCT changes. Macroarchitectural intraoperative changes (eg, inner retinal elevation, full-thickness retinal elevations) were noted to have postoperative OCT alterations in only 15% of macroarchitectural alterations cases, which were primarily noted in areas of intraoperative inner retinal elevation. Most of these transitioned to focal atrophy (64%) compared to inner retinal thickening in 36%.42
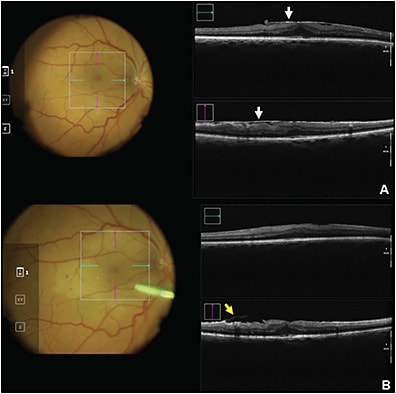
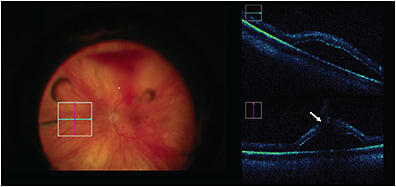
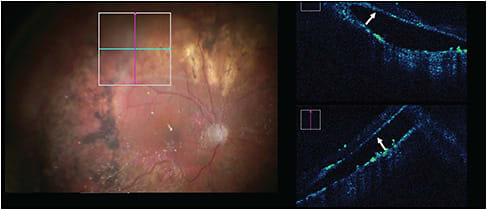
Macular Hole
The DISCOVER trial confirmed that iOCT is a feasible option for closure of macular holes,44 as demonstrated by a high closure rate corresponding with no intraoperative adverse events. Aside from visualization, iOCT has been used in research to investigate prognostic factors and identify predictive biomarkers for clinical outcomes. Inoue et al concluded that individuals with residual fragments seen on iOCT had worse postoperative visual improvement at 3 and 6 months than those without residual membrane,45 whereas Kumar et al identified an iOCT finding termed the “hole-door sign” that correlates with closure of type 1 macular holes, although not with clinical vision findings.46 Other research has been directed at identifying iOCT variables to aid in prediction of macular hole closure speed — an outcome that has implications for patient postoperative quality of life because it influences the length of time a patient has to maintain facedown positioning.31 This study found that changes in iOCT tissue measurements after ILM peeling may be a proxy for tissue elasticity and mobility that may influence how the retina will approximate.31 Intraoperative OCT is particularly helpful in this instance due to the difficulty of postoperative transtamponade imaging.44 Dynamic iOCT has also been reported to be useful in the inverted internal limiting membrane flap technique used for large macular holes (>400 µm).47
Retinal Detachment
The PIONEER study investigated 15 eyes with primary macula-involving retinal detachments (RDs) to explore iOCT characteristics during RD repair and their impact on postoperative anatomic and functional outcomes.48 Although all patients were found to have subclinical subretinal fluid during surgery on iOCT following perfluorocarbon tamponade, the fluid spontaneously resolved by the time of postoperative imaging assessment.48 In the DISCOVER trial, when specifically looking at outcomes of RD repair and impact on surgical decision making, surgeons claimed that iOCT feedback was valuable in 36% of cases. Useful information for RD included confirmation of vitreous detachment or retinal reattachment, identification and evaluation of residual fluid post-perfluorocarbon liquid or occult macular hole, necessity for retinectomy, presence of preretinal/subretinal membranes, and identification of retinal cysts.49 Intraoperative OCT data provided greater value in complex repairs than in uncomplicated primary cases.49
Therapeutic Delivery
With the emergence of subretinal gene therapy and artificial vision devices (eg, Argus by Second Sight), the role for iOCT-guided feedback may be even more critical in the future. Utilizing iOCT, multiple factors that guide successful therapeutic delivery can be ascertained: bleb location, volumetric delivery of therapeutic, and potential sequelae (eg, macular hole). One of the first described cases of visualization of subretinal therapeutics involved the delivery of subretinal tissue plasminogen activator in a subretinal hemorrhage case that provided excellent visualization of tissue plasminogen activator delivery and bleb expansion.34 Intraoperative OCT has been used to determine subretinal injection dosing by volume32 and can provide essential anatomic information during subretinal maneuvers,50 potentially working to decrease surgical technique-related complications and aiding in the delivery of stem cell and gene therapies, which require crucial precision.33,51 In addition, iOCT has been reported to aid in visualization of retinal tacking for the electrode array during placement of the Argus II retinal prosthesis for retinitis pigmentosa.52,53
CONCLUSIONS
Intraoperative OCT has been an exciting and rapidly advancing area of technology that has meaningful applications in vitreoretinal surgery and patient outcomes. Promising areas of improvement include virtual reality visualization, robot-assisted surgical techniques, automated segmentation software, automated tracking and compatible instrumentation, and overall improvement in image acquisition and quality. An integrative, digital surgical outfit that integrates seamless utilization of iOCT in real time at a highly reliable level is the ultimate goal, which is closer to realization every day. RP
REFERENCES
- Bhende M, Shetty S, Parthasarathy MK, Ramya S. Optical coherence tomography: A guide to interpretation of common macular diseases [published correction appears in Indian J Ophthalmol. 2018 Mar;66(3):485]. Indian J Ophthalmol. 2018;66(1):20-35. doi:10.4103/ijo.IJO_902_17
- Swanson EA, Fujimoto JG. The ecosystem that powered the translation of OCT from fundamental research to clinical and commercial impact [Invited]. Biomed Opt Express. 2017;8(3):1638-1664. Published 2017 Feb 21. doi:10.1364/BOE.8.001638
- Dayani PN, Maldonado R, Farsiu S, Toth CA. Intraoperative use of handheld spectral domain optical coherence tomography imaging in macular surgery [published correction appears in Retina. 2011 Jun;31(6):1236]. Retina. 2009;29(10):1457-1468. doi:10.1097/IAE.0b013e3181b266bc
- Ehlers JP, Dupps WJ, Kaiser PK, et al. The Prospective Intraoperative and Perioperative Ophthalmic ImagiNg with Optical CoherEncE TomogRaphy (PIONEER) study: 2-year results. Am J Ophthalmol. 2014;158(5):999-1007. doi:10.1016/j.ajo.2014.07.034
- Ehlers JP, Khan M, Petkovsek D, et al. Outcomes of intraoperative OCT-assisted epiretinal membrane surgery from the PIONEER study. Ophthalmol Retina. 2018;2(4):263-267. doi:10.1016/j.oret.2017.05.006
- Ray R, Barañano DE, Fortun JA, et al. Intraoperative microscope-mounted spectral domain optical coherence tomography for evaluation of retinal anatomy during macular surgery. Ophthalmology. 2011;118(11):2212-2217. doi:10.1016/j.ophtha.2011.04.012
- Ehlers JP, Tao YK, Farsiu S, Maldonado R, Izatt JA, Toth CA. Integration of a spectral domain optical coherence tomography system into a surgical microscope for intraoperative imaging. Invest Ophthalmol Vis Sci. 2011;52(6):3153-3159. Published 2011 May 16. doi:10.1167/iovs.10-6720
- Binder S, Falkner-Radler CI, Hauger C, Matz H, Glittenberg C. Feasibility of intrasurgical spectral-domain optical coherence tomography. Retina. 2011;31(7):1332-1336. doi:10.1097/IAE.0b013e3182019c18
- Pfau M, Michels S, Binder S, Becker MD. Clinical experience with the first commercially available intraoperative optical coherence tomography system. Ophthalmic Surg Lasers Imaging Retina. 2015;46(10):1001-1008. doi:10.3928/23258160-20151027-03
- Carrasco-Zevallos OM, Viehland C, Keller B, et al. Review of intraoperative optical coherence tomography: technology and applications [Invited]. Biomed Opt Express. 2017;8(3):1607-1637. Published 2017 Feb 21. doi:10.1364/BOE.8.001607
- Runkle A, Srivastava SK, Ehlers JP. Microscope-integrated OCT feasibility and utility with the Enfocus system in the DISCOVER study. Ophthalmic Surg Lasers Imaging Retina. 2017;48(3):216-222. doi:10.3928/23258160-20170301-04
- Ehlers JP, Modi YS, Pecen PE, et al. The DISCOVER study 3-year results: feasibility and usefulness of microscope-integrated intraoperative OCT during ophthalmic surgery. Ophthalmology. 2018;125(7):1014-1027. doi:10.1016/j.ophtha.2017.12.037
- Ehlers JP, Kaiser PK, Srivastava SK. Intraoperative optical coherence tomography using the RESCAN 700: preliminary results from the DISCOVER study. Br J Ophthalmol. 2014;98(10):1329-1332. doi:10.1136/bjophthalmol-2014-305294
- Ehlers JP, Goshe J, Dupps WJ, et al. Determination of feasibility and utility of microscope-integrated optical coherence tomography during ophthalmic surgery: the DISCOVER Study RESCAN Results. JAMA Ophthalmol. 2015;133(10):1124-1132. doi:10.1001/jamaophthalmol.2015.2376
- Ehlers JP, Srivastava SK, Feiler D, Noonan AI, Rollins AM, Tao YK. Integrative advances for OCT-guided ophthalmic surgery and intraoperative OCT: microscope integration, surgical instrumentation, and heads-up display surgeon feedback. PLoS One. 2014;9(8):e105224. Published 2014 Aug 20. doi:10.1371/journal.pone.0105224
- Hahn P, Migacz J, O’Donnell R, et al. Preclinical evaluation and intraoperative human retinal imaging with a high-resolution microscope-integrated spectral domain optical coherence tomography device. Retina. 2013;33(7):1328-1337. doi:10.1097/IAE.0b013e3182831293
- Figueiredo N, Talcott KE, Srivastava SK, et al. Conventional microscope-integrated intraoperative OCT versus digitally enabled intraoperative OCT in vitreoretinal surgery in the DISCOVER study. Ophthalmic Surg Lasers Imaging Retina. 2020;51(4):S37-S43. doi:10.3928/23258160-20200401-05
- Ehlers JP, Uchida A, Srivastava SK. The integrative surgical theater: combining intraoperative optical coherence tomography and 3D digital visualization for vitreoretinal surgery in the DISCOVER study. Retina. 2018;38 Suppl 1(Suppl 1):S88-S96. doi:10.1097/IAE.0000000000001999
- Shen L, Carrasco-Zevallos O, Keller B, et al. Novel microscope-integrated stereoscopic heads-up display for intrasurgical optical coherence tomography. Biomed Opt Express. 2016;7(5):1711-1726. Published 2016 Apr 6. doi:10.1364/BOE.7.001711
- Knapp AN, Talcott KE, Srivastava SK, et al. Three dimensional digitally-enabled intraoperative optical coherence tomography compared with conventional microscope-integrated intraoperative optical coherence tomography in vitreoretinal surgery: a post hoc analysis of the DISCOVER study. Paper presentation at Euretina; September 9-12 2021; Virtual.
- Carrasco-Zevallos OM, Keller B, Viehland C, et al. Live volumetric (4D) visualization and guidance of in vivo human ophthalmic surgery with intraoperative optical coherence tomography. Sci Rep. 2016;6:31689.
- Eckardt C, Paulo EB. Heads-up surgery for vitreoretinal procedures: an experimental and clinical study. Retina. 2016;36(1):137-147. doi:10.1097/IAE.0000000000000689
- Adam MK, Thornton S, Regillo CD, Park C, Ho AC, Hsu J. Minimal endoillumination levels and display luminous emittance during three-dimensional heads-up vitreoretinal surgery. Retina. 2017;37(9):1746-1749. doi:10.1097/IAE.0000000000001420
- Romano MR, Cennamo G, Comune C, et al. Evaluation of 3D heads-up vitrectomy: outcomes of psychometric skills testing and surgeon satisfaction. Eye (Lond). 2018;32(6):1093-1098. doi:10.1038/s41433-018-0027-1
- Talcott KE, Adam MK, Sioufi K, et al. Comparison of a three-dimensional heads-up display surgical platform with a standard operating microscope for macular surgery. Ophthalmol Retina. 2019;3(3):244-251. doi:10.1016/j.oret.2018.10.016
- Ehlers JP, Uchida A, Srivastava SK. Intraoperative optical coherence tomography-compatible surgical instruments for real-time image-guided ophthalmic surgery. Br J Ophthalmol. 2017;101(10):1306-1308. doi:10.1136/bjophthalmol-2017-310530
- El-Haddad MT, Tao YK. Automated stereo vision instrument tracking for intraoperative OCT guided anterior segment ophthalmic surgical maneuvers. Biomed Opt Express. 2015;6(8):3014-3031. Published 2015 Jul 23. doi:10.1364/BOE.6.003014
- Carrasco-Zevallos OM, Keller B, Viehland C, et al. Optical coherence tomography for retinal surgery: perioperative analysis to real-time four-dimensional image-guided surgery. Invest Ophthalmol Vis Sci. 2016;57(9):OCT37-OCT50. doi:10.1167/iovs.16-19277
- Xu D, Yuan A, Kaiser PK, et al. A novel segmentation algorithm for volumetric analysis of macular hole boundaries identified with optical coherence tomography. Invest Ophthalmol Vis Sci. 2013;54(1):163-169. Published 2013 Jan 7. doi:10.1167/iovs.12-10246
- Itoh Y, Vasanji A, Ehlers JP. Volumetric ellipsoid zone mapping for enhanced visualisation of outer retinal integrity with optical coherence tomography. Br J Ophthalmol. 2016;100(3):295-299. doi:10.1136/bjophthalmol-2015-307105
- Ehlers JP, Uchida A, Srivastava SK, Hu M. Predictive model for macular hole closure speed: insights from intraoperative optical coherence tomography. Transl Vis Sci Technol. 2019;8(1):18. Published 2019 Feb 6. doi:10.1167/tvst.8.1.18
- Hsu ST, Gabr H, Viehland C, et al. Volumetric measurement of subretinal blebs using microscope-integrated optical coherence tomography. Transl Vis Sci Technol. 2018;7(2):19. Published 2018 Apr 5. doi:10.1167/tvst.7.2.19
- Gregori NZ, Lam BL, Davis JL. Intraoperative use of microscope-integrated optical coherence tomography for subretinal gene therapy delivery. Retina. 2019;39 Suppl 1:S9-S12. doi:10.1097/IAE.0000000000001646
- Ehlers JP, Petkovsek DS, Yuan A, Singh RP, Srivastava SK. Intrasurgical assessment of subretinal tPA injection for submacular hemorrhage in the PIONEER study utilizing intraoperative OCT. Ophthalmic Surg Lasers Imaging Retina. 2015;46(3):327-332. doi:10.3928/23258160-20150323-05
- Song Z, Xu L, Wang J, et al. Lightweight learning-based automatic segmentation of subretinal blebs on microscope-integrated optical coherence tomography images. Am J Ophthalmol. 2021;221:154-168. doi:10.1016/j.ajo.2020.07.020
- Ehlers JP, Itoh Y, Xu LT, Kaiser PK, Singh RP, Srivastava SK. Factors associated with persistent subfoveal fluid and complete macular hole closure in the PIONEER study. Invest Ophthalmol Vis Sci. 2014;56(2):1141-1146. Published 2014 Dec 18. doi:10.1167/iovs.14-15765
- Gandorfer A, Haritoglou C, Kampik A. Toxicity of indocyanine green in vitreoretinal surgery. Dev Ophthalmol. 2008;42:69-81.
- Leisser C, Hackl C, Hirnschall N, et al. Visualizing macular structures during membrane peeling surgery with an intraoperative spectral-domain optical coherence tomography device. Ophthalmic Surg Lasers Imaging Retina. 2016;47(4):328-332. doi:10.3928/23258160-20160324-04
- Bruyère E, Philippakis E, Dupas B, Nguyen-Kim P, Tadayoni R, Couturier A. Benefit of intraoperative optical coherence tomography for vitreomacular surgery in highly myopic eyes. Retina. 2018;38(10):2035-2044. doi:10.1097/IAE.0000000000001827
- Tuifua TS, Sood AB, Abraham JR, et al. Epiretinal membrane surgery using intraoperative oct-guided membrane removal in the DISCOVER study versus conventional membrane removal [published online ahead of print, 2021 Feb 27]. Ophthalmol Retina. 2021;S2468-6530(21)00067-1. doi:10.1016/j.oret.2021.02.013
- Leisser C, Hackl C, Hirnschall N, Findl O. Effect of subfoveal and extrafoveal hyporeflective zones due to iatrogenic traction during membrane peeling for epiretinal membranes on postoperative outcomes. Ophthalmologica. 2020;243(4):297-302. doi:10.1159/000505214
- Leisser C, Hirnschall N, Hackl C, et al. Risk factors for postoperative intraretinal cystoid changes after peeling of idiopathic epiretinal membranes among patients randomized for balanced salt solution and air-tamponade. Acta Ophthalmol. 2018;96(4):e439-e444. doi:10.1111/aos.13635
- Ehlers JP, Han J, Petkovsek D, Kaiser PK, Singh RP, Srivastava SK. Membrane peeling-induced retinal alterations on intraoperative OCT in vitreomacular interface disorders from the PIONEER study. Invest Ophthalmol Vis Sci. 2015;56(12):7324-7330. doi:10.1167/iovs.15-17526
- Yee P, Sevgi DD, Abraham J, et al. iOCT-assisted macular hole surgery: outcomes and utility from the DISCOVER study. Br J Ophthalmol. 2021;105(3):403-409. doi:10.1136/bjophthalmol-2020-316045
- Inoue M, Itoh Y, Koto T, Kurimori HY, Hirakata A. Intraoperative OCT Findings May Predict Postoperative Visual Outcome in Eyes with Idiopathic Macular Hole. Ophthalmol Retina. 2019;3(11):962-970. doi:10.1016/j.oret.2019.05.022
- Kumar V, Yadav B. Hole-door sign: a novel intraoperative optical coherence tomography feature predicting macular hole closure. Retina. 2018;38(10):2045-2050. doi:10.1097/IAE.0000000000001791
- Lytvynchuk LM, Falkner-Radler CI, Krepler K, et al. Dynamic intraoperative optical coherence tomography for inverted internal limiting membrane flap technique in large macular hole surgery. Graefes Arch Clin Exp Ophthalmol. 2019;257(8):1649-1659. doi:10.1007/s00417-019-04364-5
- Abraham JR, Srivastava SK, Reese JL, Ehlers JP. Intraoperative OCT features and postoperative ellipsoid mapping in primary macula-involving retinal detachments from the PIONEER study. Ophthalmol Retina. 2019;3(3):252-257. doi:10.1016/j.oret.2018.10.006
- Abraham JR, Srivastava SK, K Le T, et al. Intraoperative OCT-assisted retinal detachment repair in the DISCOVER study: impact and outcomes. Ophthalmol Retina. 2020;4(4):378-383. doi:10.1016/j.oret.2019.11.002
- Pasricha ND, Bhullar PK, Shieh C, et al. Four-dimensional microscope-integrated optical coherence tomography to enhance visualization in glaucoma surgeries. Indian J Ophthalmol. 2017;65(1):57-59. doi:10.4103/ijo.IJO_412_16
- Davis JL. The blunt end: surgical challenges of gene therapy for inherited retinal diseases. Am J Ophthalmol. 2018;196:xxv-xxix. doi:10.1016/j.ajo.2018.08.038
- Rachitskaya A, Lane L, Ehlers J, DeBenedictis M, Yuan A. Argus II retinal prosthesis implantation using three-dimensional visualization system. Retina. 2019;39 Suppl 1:S199-S200. doi:10.1097/IAE.0000000000002296
- Grewal DS, Carrasco-Zevallos OM, Gunther R, Izatt JA, Toth CA, Hahn P. Intra-operative microscope-integrated swept-source optical coherence tomography guided placement of Argus II retinal prosthesis. Acta Ophthalmol. 2017;95(5):e431-e432. doi:10.1111/aos.13123