Retinal surgery involves sensory and motor skills that approach or exceed the human physiological limits for steadiness, positional accuracy, and the tactile sensing of forces. Furthermore, retinal surgery involves the manipulation of target tissue that is delicate, transparent, and nonregenerative — all within the confines of a small, constrained intraocular surgical environment.
Naturally, these surgical difficulties have motivated engineers and health professionals to investigate how robotic technologies can benefit retinal surgery. For example, a robot’s stability and high-resolution motion control are well suited to performing intraocular procedures that demand high positional accuracy, such as subretinal treatments or retinal vein cannulation (RVC).
Despite these promises, the incorporation of robotics into intraocular surgery has lagged behind that of other fields. The first in-human, robot-assisted retinal procedures occurred in 2018 — almost 3 decades after the first journal paper on the subject.1-3 This delay can be attributed to at least 2 factors. First, the unique advantages of intraocular surgical procedures for human surgeons (such as direct visualization of the surgical workspace and unhindered maneuverability of intraocular instrumentation) have eclipsed the apparent benefits of incorporating robotics. Second, before robotic-assisted retinal surgical procedures could be demonstrated, it required significant time and effort to develop the necessary technological advances in robotic systems and imaging modalities specific to eye surgery.
Despite the underlying assumption that the integration of robotics will result in appreciable benefits to surgical outcomes — and despite substantial efforts in developing prototypes — many challenges remain. This article introduces some of the most successful systems and discusses the benefits and challenges of incorporating robotics into retinal surgery.
ROBOTIC SYSTEMS FOR RETINAL SURGERY
The first robotic system developed for intraocular procedures was reported in 1989.3 Since then, a dozen more systems have been developed. Initial devices were handheld tools that cancelled tremor and included depth-locking features, such as the Micron from Johns Hopkins University.4 Additional systems included articulated-arm robots, such as the Intraocular Robotic Interventional Surgical System (IRISS) from the University of California, Los Angeles (UCLA)5 or piezo-actuated devices, such as RAM!S from the Technical University of Munich.6
One of the first clinical successes on human patients was achieved by the Preceyes Surgical System in 2018.1 In this study, a teleoperated robotic system was used to perform membrane dissection in human patients. Surgical results with robotic assistance were equally successful compared to those without, albeit longer with use of the robotic system. In addition, the Preceyes robot was used to demonstrate injection of recombinant tissue plasminogen activator into the subretinal space, further demonstrating the degree of achievable precision.
Since then, the Preceyes Surgical System (Figure 1) has become the only intraocular robotic surgical system to become commercially available. With this system, a high degree of tool-tip positional precision can be obtained, and virtual boundaries can be imposed to restrict unwanted movement and prevent iatrogenic retinal trauma. The Preceyes group continues to publish research results, and at least 3 devices are currently being used in hospitals across Europe.
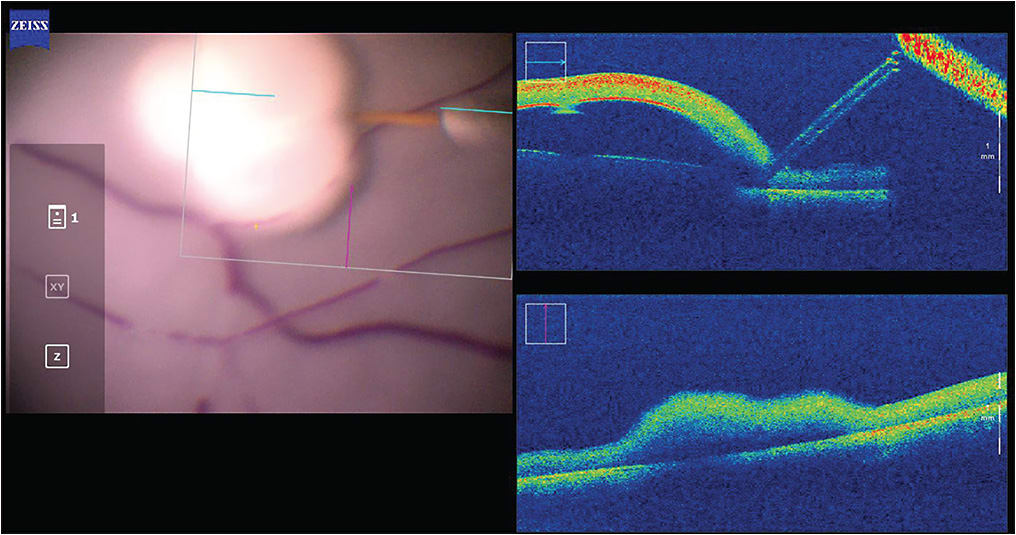
Another clinical success in human patients was demonstrated by the University of Leuven, Belgium, in 2018, in the context of a phase 1 clinical trial.2 The group used a co-manipulated robotic system to perform RVC on 4 patients, thereby demonstrating the technical feasibility of performing high-precision surgical techniques with the assistance of a robotic system (Figure 2). Development and commercialization of this system continues as Mynutia, a spinoff company of the university.
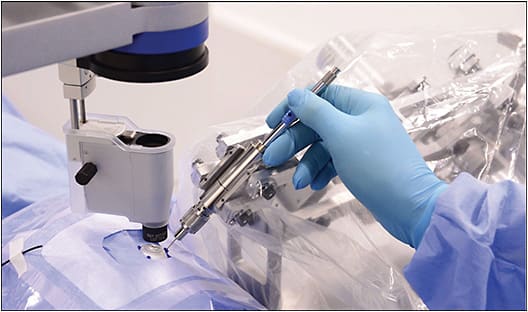
In terms of autonomous robotic systems, all reported studies exist at the research stage without any reported clinical trials. Perhaps the most advanced autonomous intraocular system is the IRISS from UCLA, primarily developed for cataract surgery.7 However, the IRISS has also been used to perform fully autonomous RVC on silicone eye models.8 Using a combination of OCT data and camera images, the system was shown to be capable of cannulating vein phantoms as small as 120 µm without input from a human operator (Figure 3). Important questions remain regarding the representativeness of the artificial vein model and how well this system could be applied to in vivo RVC, but the study’s success lends confidence to the idea that specific steps of surgical procedures can be autonomously performed.
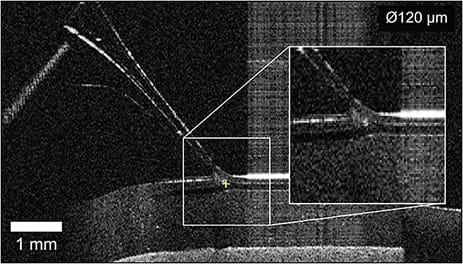
Areas of recent research in robotic retinal surgery include the following:
- Intraoperative identification of the location and pose of surgical instruments through image processing of microscope data and/or iOCT scans;9,10
- Development of force-sensing instruments capable of sensing minute forces below those of human tactile perception;11,12
- OCT-fiber-integrated surgical instruments capable of imaging microstructures or quantifying tool-to-tissue distances;13,14 and
- Chemical or bioimpedance-based tools capable of identifying tissue15 or indicating when a vein has been cannulated.16
These developments are interesting both for their potential to enhance the safety, efficacy, and functionality of manually performed surgical procedures, and also for their potential to provide feedback for guiding future autonomous robotic systems.
POTENTIAL BENEFITS OF ROBOTIC SYSTEMS
One clear advantage of robotic systems is their positional accuracy and precision. For example, the positional accuracy of a human surgeon during retinal surgery is, at best, 20 µm to 40 µm17 with mean peak-to-peak magnitude of 100 µm.18 In comparison, the positional precision reported by Preceyes is 3 µm,19 and state-of-the-art piezoelectric actuators are capable of nanometer-level resolutions (suggesting room for even greater accuracies). Furthermore, robotic systems are capable of holding a tool steady for an indefinite period of time — a feat that is infeasible for a human surgeon.
Robotic systems are also well suited to performing repetitive or routine tasks. For simple procedures, surgical requirements are clearly defined and metrics of success are readily available. In these cases, a robotic system can be used to perform a task repeatably within well-defined safety bounds, thereby reducing surgeon variability and ultimately standardizing the quality of care across all patients.
Finally, many surgeons, as part of their surgical training, use simulators to develop necessary skills. While these systems have proven invaluable in shortening the learning curve and reducing surgical mistakes, they are not a perfect analog to actual surgical procedures. However, if robotic systems become widely employed in the future, simulators can be designed to precisely mimic the performance characteristics of surgical robotic systems.
CHALLENGES AND LIMITATIONS
Despite the potential benefits, many obstacles remain in the way of incorporating robotics into standard clinical practice. Here, we highlight 3 challenges toward adoption of robotic systems.
First, a robotic system, like any new technology, can be expected to be accompanied by a steep learning curve for both the surgeon and surgical team. That learning curve will have an associated time and cost, and it will introduce a suite of potential procedural alterations.
Second, the financial implications of purchasing, integrating, and maintaining a robotic system into a surgical theater must be considered. The added financial burden must be justified through repeatable, positive surgical outcomes that are at least as safe as manually performed surgical procedures. In addition, a high price tag may be warranted if surgical procedures can be performed more efficiently (and increase patient throughput) or if routine procedures can be performed by the robotic system (thereby releasing surgeons to perform more complex surgeries). In contrast, a clinic may not have the minimum patient demand to justify the purchase of a robotic system, or the integration of a robotic system may have other, hidden costs associated with its use that must be considered.
Third, the safety concerns associated with using novel technology weighs heavily on the minds of patients, surgeons, and regulatory agencies. If we are to see robotic systems garner widespread use, the trust of early adopters and future patients must be won over. To build this trust, robust clinical trials must be performed to firmly establish safety in human patients and clearly demonstrate outcomes at least as good as those of current practice. These concerns, and others, can be expected to be the deciding factors in whether or not to incorporate and use a robotic system in retinal surgery.
CONCLUSION
The future of robotic systems in retinal surgery will most likely depend on the results of the clinical trials currently being performed by groups such as Preceyes and Mynutia, as well as the successes (and failures) of recent start-up companies. While these systems have already begun to show promising results, many challenges remain, and engineers and surgeons will continue to develop novel technologies to ensure future systems will improve surgical outcomes, enhance procedure efficacy, and extend surgical capability by bringing currently infeasible surgical procedures into the realm of treatment options.
In the immediate future, we envision the use of high-precision teleoperated robotic systems capable of autonomously performing specific, routine tasks. Such systems will undoubtedly include an essential role for a human surgeon, especially during early stages of development. In the far future, it is not unreasonable to suggest that entire surgical procedures could be performed by a fully autonomous robotic system, guided by artificial intelligence and relying on sensing technologies from multimodal imaging modalities. While engineering and adoption challenges remain, the future of robotic systems in retinal surgery is promising and the full potential has only recently begun to be realized. RP
REFERENCES
- Edwards TL, Xue K, Meenink HCM, et al. First-in-human study of the safety and viability of intraocular robotic surgery. Nat Biomed Eng. 2018;2:649-656. doi:10.1038/s41551-018-0248-4
- Gijbels A, Smits J, Schoevaerdts L, et al. In-Human Robot-Assisted Retinal Vein Cannulation, A World First. Ann Biomed Eng. 2018;46(10):1676-1685. doi:10.1007/s10439-018-2053-3
- Guerrouad A, Vidal P. SMOS: stereotaxical microtelemanipulator for ocular surgery. Annu Int Conf IEEE Eng Med Biol Soc. 1989. https://ieeexplore.ieee.org/document/96028
- Maclachlan RA, Becker BC, Tabarés JC, Podnar GW, Lobes LA Jr, Riviere CN. Micron: an Actively Stabilized Handheld Tool for Microsurgery. IEEE Trans Robot. 2012;28(1):195-212. doi:10.1109/TRO.2011.2169634
- Wilson JT, Gerber MJ, Prince SW, et al. Intraocular robotic interventional surgical system (IRISS): Mechanical design, evaluation, and master-slave manipulation. Int J Med Robot. 2018;14(1):10.1002/rcs.1842. doi:10.1002/rcs.1842
- Nasseri MA, Eder M, Nair S, et al. The introduction of a new robot for assistance in ophthalmic surgery. Annu Int Conf IEEE Eng Med Biol Soc. 2013;2013:5682-5685. doi:10.1109/EMBC.2013.6610840
- Chen CW, Francone AA, Gerber MJ, et al. Semiautomated optical coherence tomography-guided robotic surgery for porcine lens removal. J Cataract Refract Surg. 2019;45(11):1665-1669. doi:10.1016/j.jcrs.2019.06.020
- Gerber MJ, Hubschman JP, Tsao TC. Automated retinal vein cannulation on silicone phantoms using optical coherence tomography-guided robotic manipulations. IEEE/ASME Transactions on Mechatronics. 2020. https://ieeexplore.ieee.org/document/9298929
- Zhou M, Roodaki H, Eslami A, et al. Needle segmentation in volumetric optical coherence tomography images for ophthalmic microsurgery. Appl Sci. 2017;7(8):748. https://doi.org/10.3390/app7080748
- Gonenc B, Chamani A, Handa J, Gehlbach P, Taylor RH, Iordachita I. 3-DOF force-sensing motorized micro-forceps for robot-assisted vitreoretinal surgery. IEEE Sens J. 2017;17(11):3526-3541. doi:10.1109/JSEN.2017.2694965
- He C, Patel N, Ebrahimi A, Kobilarov M, Iordachita I. Preliminary study of an RNN-based active interventional robotic system (AIRS) in retinal microsurgery. Int J Comput Assist Radiol Surg. 2019;14(6):945-954. doi:10.1007/s11548-019-01947-9
- Smits J, Ourak M, Gijbels A, et al. Development and experimental validation of a combined fbg force and oct distance sensing needle for robot-assisted retinal vein cannulation. IEEE International Conference on Robotics and Automation (ICRA). 2018:129-124. doi:10.1109/ICRA.2018.8460983.
- Joos KM, Shen JH. Miniature real-time intraoperative forward-imaging optical coherence tomography probe. Biomed Opt Express. 2013;4(8):1342-1350. Published 2013 Jul 16. doi:10.1364/BOE.4.001342
- Asami T, Terasaki H, Ito Y, et al. Development of a fiber-optic optical coherence tomography probe for intraocular use. Invest Ophthalmol Vis Sci. 2016;57(9):OCT568-OCT574. doi:10.1167/iovs.15-18853
- Aghajanipedram S, Ferguson PW, Gerber MJ, Shin C, Hubschman JP, Rosen J. A novel tissue identification framework in cataract surgery using an integrated bioimpedance-based probe and machine learning algorithms [published online ahead of print, 2021 Sep 1]. IEEE Trans Biomed Eng. 2021;PP:10.1109/TBME.2021.3109246. doi:10.1109/TBME.2021.3109246
- Schoevaerdts L, Borghesan G, Ourak M, Reynaerts D, Vander Poorten E. Electrical bio-impedance proximity sensing for vitreo-retinal micro-surgery. IEEE Robotics and Automation Letters. 2019;4(4):4086-4093.
- Jagtap AS, Riviere CN. Applied force during vitreoretinal microsurgery with handheld instruments. Conf Proc IEEE Eng Med Biol Soc. 2004;2004:2771-2773. doi:10.1109/IEMBS.2004.1403792
- Singhy SPN, Riviere CN. Physiological tremor amplitude during retinal microsurgery. Proc IEEE Ann Northeast Bioeng Conf. 2002. DOI:10.1109/NEBC.2002.999520
- de Smet MD, de Jonge N, Iannetta D, et al. Human/robotic interaction: vision limits performance in simulated vitreoretinal surgery. Acta Ophthalmol. 2019;97(7):672-678. doi:10.1111/aos.14003