Fundus autofluorescence (FAF) is a noninvasive tool to characterize the structure and function of the retinal pigment epithelium (RPE)/RPE complex. As an imaging modality, it may often detect abnormalities not seen on clinical examination. Clinical applications of FAF continue to expand, and this article provides an overview of the basics, some prominent clinical uses, and recent developments for FAF.
PRINCIPLES AND BASIS FOR IMAGING
Fundus autofluorescence relies on the property of the RPE to emit certain wavelengths in response to excitation by short-wavelength light, with the dominant fluorophore being RPE lipofuscin, which contains the fluorescent pigment A2E.1 Accumulation of lipofuscin within the RPE is most likely a byproduct of the phagocytosis of discs shed by retinal photoreceptor outer segments, a process integral to the vision cycle.2-4
The major categories of devices used to obtain FAF images are confocal scanning laser ophthalmoscopy (cSLO), modified conventional fundus camera (mFC), and ultrawidefield ophthalmoscopic devices.5 The 3 devices use a variety of excitation wavelengths and record autofluorescence (AF) emissions in a certain range.2,5-7 The mFC uses a single bright flash of light to image the entire retinal field, which can lead to an inferior signal-to-noise ratio and autofluorescence signal originating from extraretinal structures (ie, the lens), which may make diagnostic interpretation difficult.8,9 The cSLO uses a low excitation intensity and records the average of several emission images, for an improved signal-to-noise ratio.9
In the normal fundus, the center of the macula shows decreased AF due to blockage by the light-absorbing pigments lutein and zeaxanthin, which are abundant in the fovea. The optic disc appears dark because of the absence of lipofuscin, and blood vessels appear dark because light is absorbed by blood. The remainder of the macula shows a homogeneous signal, which tends to increase with age due to gradual accumulation of RPE lipofuscin.2
In addition to increased signal associated with aging, lipofuscin accumulation caused by RPE dysfunction can lead to increased AF. Reduced AF can be caused by pigment or reduced lipofuscin density, as discussed above, as well as fibrosis, intraretinal fluid, and RPE loss or atrophy.
FUNDUS AUTOFLUORESCENCE IN VARIOUS DISEASE CONDITIONS
Below is a review of some common and important conditions for which FAF has been demonstrated to be useful diagnostically and prognostically.
DRUG TOXICITY AND MONITORING
Hydroxychloroquine and Chloroquine
Retinopathy due to toxicity from chloroquine and hydroxychloroquine has been well recognized and can lead to severe and irreversible vision loss. These medications, most commonly hydroxychloroquine, are used in the treatment of systemic inflammatory disorders, such as systemic lupus erythematosus, rheumatoid arthritis, dermatologic conditions, malaria, and, most recently, experimentally in the acute setting for treatment or postexposure prophylaxis of coronavirus disease (COVID-19) caused by the severe acute respiratory syndrome coronavirus 2 (SARS-CoV-2).10,11
Screening guidelines for toxic retinopathy due to chloroquine and hydroxychloroquine have changed in recent years as a result of evolving data, but they involve evaluation of the visual field, spectral-domain optical coherence tomography (SD-OCT), FAF, and multifocal electroretinogram (mfERG). Risk of toxicity increases with increased daily dose, total duration of use, concomitant renal disease, and use of tamoxifen. Recommended dosage to reduce the risk of retinopathy is <5.0 mg/kg/day based on real body weight.12
Damage from hydroxychloroquine and chloroquine toxicity appears to primarily affect the perifoveal photoreceptors, with secondary damage to the RPE. Early on, changes are noted on SD-OCT and mfERG, and increased AF is seen with FAF, likely due to early RPE dysfunction. Late findings include atrophy and decreased AF. In Asian patients, toxicity may show extramacular findings; thus, for these patients, wide-angle FAF may be useful (Figure 1).12
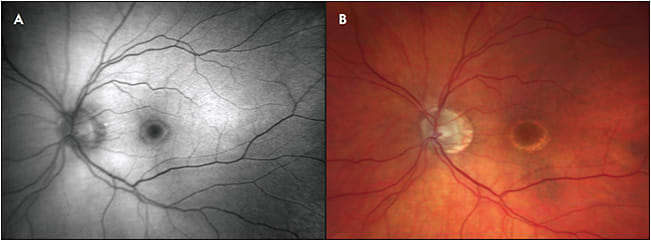
Pentosan Polysulfate Sodium
In 2018, a pigmentary maculopathy was described in the setting of chronic exposure to the drug pentosan polysulfate sodium (PPS), which is used in the treatment of interstitial cystitis. Visual acuity appeared to be preserved initially, with patients reporting difficulty reading and prolonged dark adaptation.13,14 This toxic maculopathy has been thought to progress after discontinuation of PPS (Figure 2).15 On fundus examination, there are often subtle hyperpigmented macular spots with interspersed pale yellow deposits. Fundus autofluorescence images may demonstrate large confluent areas of mottled increased and decreased AF spots, in most cases involving the fovea.16 Spectral-domain OCT shows elevation and thickening of the RPE. These RPE findings have been noted to be dynamic over time.16 Some reports have demonstrated more widespread changes correlating to the cumulative dose of PPS, with attempts to quantify the imaging changes.17 With increased awareness of this likely drug toxicity, we can expect to continue to learn more about the natural history, risk factors, and management of this condition. Recent data have led some urologists to question the role of PPS in treating interstitial cystitis: a randomized, double-blind controlled trial showed no benefit of the drug over placebo in controlling symptoms of interstitial cystitis.18
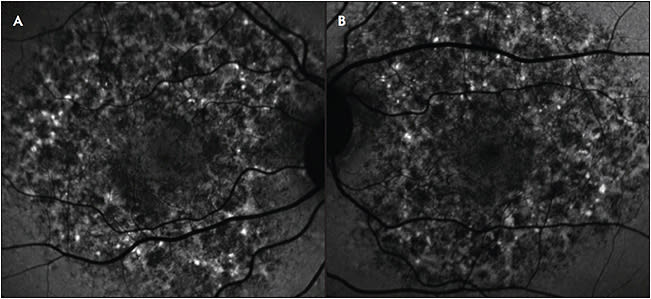
AGE-RELATED MACULAR DEGENERATION
Fundus autofluorescence has been used to detect changes in eyes that have age-related macular degeneration (AMD), including identification of intermediate and large drusen, reticular pseudodrusen, cuticular drusen, and geographic atrophy (GA).8,9,19 Intermediate (63 µm to 125 µm diameter) and large (>125 µm diameter) drusen appear as foci of increased AF (Figure 3), and drusenoid pigment epithelial detachments (PEDs) appear as increased AF lesions surrounded by a halo of decreased AF. A higher risk of disease progression has been associated with drusenoid PEDs and increased number and size of drusen.
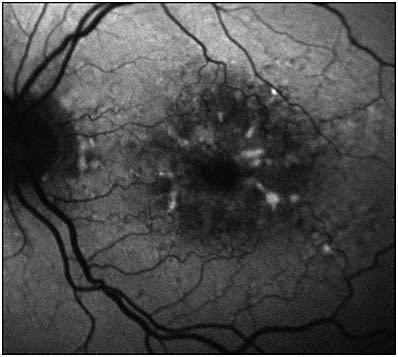
Cuticular drusen appear as foci of decreased AF. Reticular pseudodrusen, which are accumulations of material above the RPE, appear as round foci of decreased AF with interspersed areas of increased AF.19 Reticular pseudodrusen are also associated with a high risk of progression to advanced AMD.
GEOGRAPHIC ATROPHY
Geographic atrophy, sharply delineated round areas of hypopigmentation or depigmentation measuring at least 175 µm in diameter, is a manifestation of late-stage non-neovascular AMD, as well as other retinal degenerations and inherited retinal dystrophies.20 These atrophic lesions of RPE, choroid, and outer neurosensory retina tend to enlarge over time and cause absolute scotomas in the corresponding visual field, eventually leading to profound vision loss if the fovea becomes involved.21-24 On FAF, GA appears as decreased AF, often with increased AF at the borders of atrophy in a variety of different phenotypes. Classification of these distinct hyperautofluorescent patterns has been done by the Fundus Autofluorescence in Age-related Macular Degeneration (FAM) study group, among others, and suggests that different phenotypic FAF patterns are associated with different rates of GA progression.25 Thus, FAF may serve a prognostic role in the future management of patients who have GA. Changes in FAF may be observable prior to visual acuity changes, allowing for monitoring of disease progression (Figure 4).26
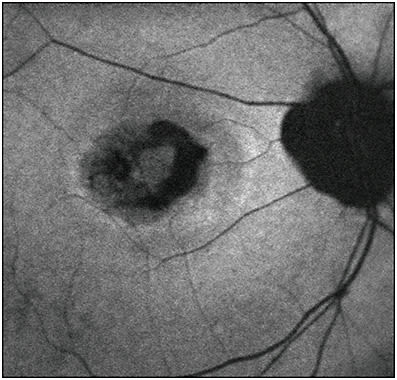
PATTERN DYSTROPHIES
A number of retinal dystrophies were classically described by Gass as pattern dystrophies and include adult-onset foveomacular vitelliform dystrophy (AFVD), butterfly macular dystrophy, reticular dystrophy, multifocal pattern dystrophy simulating fundus flavimaculatus, and fundus pulverulentus. These are bilateral, symmetric, and associated with autosomal dominant mutations in PRPH2 on chromosome 6.27 In AFVD, early subfoveal vitelliform lesions demonstrate increased AF, and progression of possible atrophic disease demonstrates decreased AF (Figure 5). In multifocal pattern dystrophy simulating fundus flavimaculatus, a “dot and halo” pattern of AF is seen with yellowish flecks, demonstrating decreased AF surrounded by a halo of increased AF.27 The other pattern dystrophies demonstrate a variety of FAF morphologies, including ring-like, spoke-like, and reticular changes in the macula and posterior pole. Fundus autofluorescence findings in these subtypes have not been as well characterized.
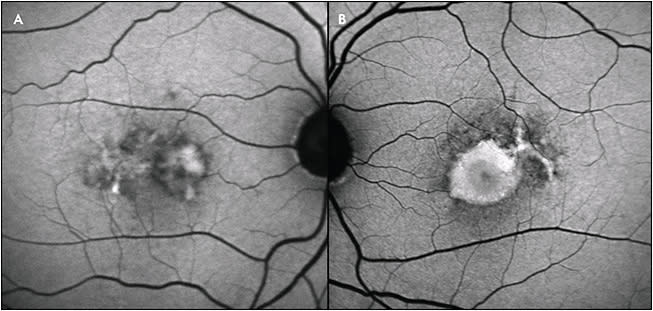
OTHER INHERITED RETINAL DISEASES
Fundus autofluorescence may be used to monitor progression of a variety of inherited retinal diseases. For example, in retinitis pigmentosa, a disease predominantly of rod dysfunction, FAF may demonstrate a parafoveal ring of increased AF correlating to rod photoreceptor outer segment and secondary RPE dysfunction.28,29 Cone dystrophies may demonstrate increased AF of the fovea or a ring of increased AF similar to that seen in retinitis pigmentosa, and these patterns may be seen in rod dystrophies with cone involvement as well.30,31 The areas of abnormal AF correspond to areas of decreased visual function as measured on perimetry and electroretinogram (Figure 6).32
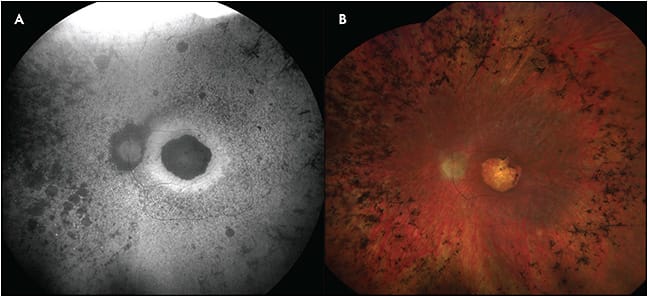
Maculopathies such as Stargardt disease may manifest with initial increased AF and progressive decreased AF over areas of classic “yellow flecks” that may be present. These classic fleck lesions expand centrifugally with time, sparing the peripapillary area.33 Several recent prospective studies evaluating FAF in Stargardt disease demonstrated the utility of serial FAF in following disease progression.34,35
Best disease, which classically presents at a young age with bilateral yolk-like submacular deposits, is thought to be caused by RPE dysfunction, which leads to accumulation of photoreceptors, lipofuscin, and debris in the submacular space.
The disease classically progresses through 5 stages, as initially described, based on biomicroscopic appearance, and FAF demonstrates unique findings at each stage.36-38 In the vitelliform, or second stage, there is homogeneously increased AF. The pseudohypopyon stage demonstrates increased AF in the inferior portion of the lesion. In the scrambled-egg stage, the lesion’s border typically has increased AF, while the center may have decreased AF (Figure 7), and in the atrophic stage, AF signal is significantly decreased.38
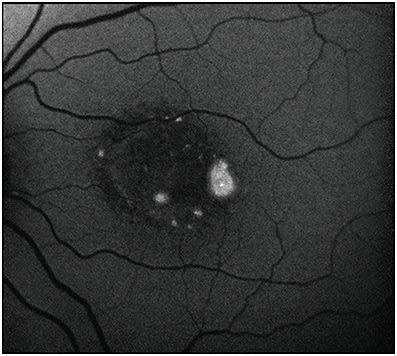
CENTRAL SEROUS CHORIORETINOPATHY
Central serous chorioretinopathy (CSCR) is a condition characterized by RPE and serous retinal detachment, often in the posterior pole. The pathophysiology is unclear, but hyperpermeability of the choroid is thought to lead to leakage of fluid through the RPE and into the subretinal space, and the strongest known associated risk factor is exogenous glucocorticoid.39,40 On FAF, areas of serous retinal detachment demonstrate increased AF, particularly at the borders of the detachment. In acute CSCR, small punctate areas of increased AF correspond to punctate subretinal precipitates seen on funduscopic examination. Diffuse RPE and outer retinal atrophy, which can be seen in chronic CSCR, appears as decreased AF.41,42 In some cases, gravitational tracking, or “guttering,” is seen in areas of atrophy secondary to chronic subretinal fluid (Figure 8).43
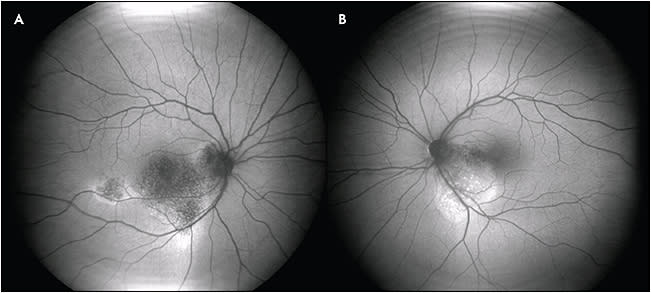
RECENT DEVELOPMENTS IN FAF IMAGING
In recent years, procedures for quantitative FAF (qFAF) have been developed using cSLO. Measurements of FAF intensity and spatial information are used. This provides the advantages of discerning truly abnormal signal intensity from normal age-related increases in AF, directly comparing FAF imaging of an eye with subsequent images over time, and allowing for interpatient comparisons of AF.7,44 With a quantitative approach, differences in lesion size, distribution, and patterns will be able to be objectively analyzed.
Initial research utilizing qFAF has been seen in conditions such as AMD, Stargardt disease, Best disease, retinitis pigmentosa, acute zonal occult outer retinopathy, and pseudoxanthoma elasticum, as well as in basic and translational animal studies.7
LIMITATIONS OF FAF
The limitations of fundus autofluorescence may relate to image quality and acquisition. As discussed, autofluorescence artifacts from the ocular media reduce the signal-to-noise ratio. The phenomenon of photoreceptor bleaching, due to rhodopsin absorption and photoisomerization, limits the ability of examiners to obtain reliable FAF and fluorescein angiography in the same sitting, because recently excited photoreceptors demonstrate relatively increased AF.45,46 RP
REFERENCES
- Delori FC, Dorey CK, Staurenghi G, Arend O, Goger DG, Weiter JJ. In vivo fluorescence of the ocular fundus exhibits retinal pigment epithelium lipofuscin characteristics. Invest Ophthalmol Vis Sci. 1995;36(3):718-729.
- Tsang SH, Sharma T. Fundus autofluorescence. Adv Exp Med Biol. 2018;1085:15-16. doi: 10.1007/978-3-319-95046-4_4
- Boulton M, McKechnie NM, Breda J, Bayly M, Marshall J. The formation of autofluorescent granules in cultured human RPE. Invest Ophthalmol Vis Sci. 1989;30(1):82-89.
- Wassell J, Ellis S, Burke J, Boulton M. Fluorescence properties of autofluorescent granules generated by cultured human RPE cells. Invest Ophthalmol Vis Sci. 1998 Jul;39(8):1487-1492.
- Calvo-Maroto AM, Esteve-Taboada JJ, Domínguez-Vicent A, Pérez-Cambrodí RJ, Cerviño A. Confocal scanning laser ophthalmoscopy versus modified conventional fundus camera for fundus autofluorescence. Expert Rev Med Devices. 2016;13(10):965-978. doi:10.1080/17434440.2016.1236678.
- Spaide RF. Fundus autofluorescence and age-related macular degeneration. Ophthalmology. 2003;110(2):392-399. doi:10.1016/S0161-6420(02)01756-6
- Sparrow JR, Duncker T, Schuerch K, Paavo M, de Carvalho JRL Jr. Lessons learned from quantitative fundus autofluorescence. Prog Retin Eye Res. 2020;74:100774. doi:10.1016/j.preteyeres.2019.100774
- Schmitz-Valckenberg S, Fleckenstein M, Göbel AP, et al. Evaluation of autofluorescence imaging with the scanning laser ophthalmoscope and the fundus camera in age-related geographic atrophy. Am J Ophthalmol. 2008;146(2):183-192. doi:10.1016/j.ajo.2008.04.006
- Choudhry N, Giani A, Miller JW. Fundus autofluorescence in geographic atrophy: a review. Semin Ophthalmol. 2010;25(5-6):206-213. doi:10.3109/08820538.2010.518121
- Geleris J, Sun Y, Platt J, et al. Observational study of hydroxychloroquine in hospitalized patients with covid-19. N Engl J Med. 2020;382(25):2411-2418. doi:10.1056/NEJMoa2012410
- Boulware DR, Pullen MF, Bangdiwala AS, et al. A randomized trial of hydroxychloroquine as postexposure prophylaxis for covid-19. N Engl J Med. 2020;383(6):517-525. doi: 10.1056/NEJMoa2016638
- Marmor MF, Kellner U, Lai TY, Melles RB, Mieler WF; American Academy of Ophthalmology. Recommendations on screening for chloroquine and hydroxychloroquine retinopathy (2016 revision). Ophthalmology. 2016;123(6):1386-1394. doi:10.1016/j.ophtha.2016.01.058
- Pearce WA, Chen R, Jain N. Pigmentary maculopathy associated with chronic exposure to pentosan polysulfate sodium. Ophthalmology. 2018;125(11):1793-1802. doi:10.1016/j.ophtha.2018.04.026
- Hanif AM, Shah R, Yan J, et al. Strength of association between pentosan polysulfate and a novel maculopathy. Ophthalmology. 2019;126(10):1464-1466. doi:10.1016/j.ophtha.2019.04.024
- Huckfeldt RM, Vavvas DG. Progressive maculopathy after discontinuation of pentosan polysulfate sodium. Ophthalmic Surg Lasers Imaging Retina. 2019;50(10):656-659. doi:10.3928/23258160-20191009-10
- Hanif AM, Armenti ST, Taylor SC, et al. Phenotypic spectrum of pentosan polysulfate sodium-associated maculopathy: a multicenter study. JAMA Ophthalmol. 2019;137(11):1275-1282. doi:10.1001/jamaophthalmol.2019.3392
- Hadad A, Helmy O, Leeman S, Schaal S. A Novel multimethod image analysis to quantify pentosan polysulfate sodium retinal toxicity. Ophthalmology. 2020;127(3):429-431. doi:10.1016/j.ophtha.2019.10.013
- Nickel JC, Herschorn S, Whitmore KE, et al. Pentosan polysulfate sodium for treatment of interstitial cystitis/bladder pain syndrome: insights from a randomized, double-blind, placebo controlled study. J Urol. 2015;193(3):857-862. doi:10.1016/j.juro.2014.09.036
- Banda HK, Shah GK, Blinder KJ. Applications of fundus autofluorescence and widefield angiography in clinical practice. Can J Ophthalmol. 2019;54(1):11-19. doi:10.1016/j.jcjo.2018.10.003
- Bird AC, Bressler NM, Bressler SB, et al. An international classification and grading system for age-related maculopathy and age-related macular degeneration. The International ARM Epidemiological Study Group. Surv Ophthalmol. 1995;39(5):367-74. doi:10.1016/s0039-6257(05)80092-x
- Schatz H, McDonald HR. Atrophic macular degeneration. Rate of spread of geographic atrophy and visual loss. Ophthalmology. 1989;96(10):1541-1551. doi:10.1016/s0161-6420(89)32694-7
- Sunness JS. The natural history of geographic atrophy, the advanced atrophic form of age-related macular degeneration. Mol Vis. 1999;5:25.
- Maguire P, Vine AK. Geographic atrophy of the retinal pigment epithelium. Am J Ophthalmol. 1986;102(5):621-625. doi:10.1016/0002-9394(86)90535-0
- Sunness JS, Margalit E, Srikumaran D, et al. The long-term natural history of geographic atrophy from age-related macular degeneration: enlargement of atrophy and implications for interventional clinical trials. Ophthalmology. 2007;114(2):271-277. doi:10.1016/j.ophtha.2006.09.016
- Holz FG, Bindewald-Wittich A, Fleckenstein M, et al; FAM-Study Group. Progression of geographic atrophy and impact of fundus autofluorescence patterns in age-related macular degeneration. Am J Ophthalmol. 2007;143(3):463-472. doi:10.1016/j.ajo.2006.11.041
- Lois N, Owens SL, Coco R, Hopkins J, Fitzke FW, Bird AC. Fundus autofluorescence in patients with age-related macular degeneration and high risk of visual loss. Am J Ophthalmol. 2002;133(3):341-349. doi:10.1016/s0002-9394(01)01404-0
- Gass JDM. Stereoscopic Atlas of Macular Diseases: Diagnosis & Treatment. Mosby; 1997.
- Ogura S, Yasukawa T, Kato A, et al. Wide-field fundus autofluorescence imaging to evaluate retinal function in patients with retinitis pigmentosa. Am J Ophthalmol. 2014;158(5):1093-1098. doi:10.1016/j.ajo.2014.07.021
- Mitamura Y, Mitamura-Aizawa S, Nagasawa T, Katome T, Eguchi H, Naito T. Diagnostic imaging in patients with retinitis pigmentosa. J Med Invest. 2012;59(1-2):1-11. doi:10.2152/jmi.59.1
- Wang NK, Chou CL, Lima LH, et al. Fundus autofluorescence in cone dystrophy. Doc Ophthalmol. 2009;119(2):141-144. doi:10.1007/s10633-009-9172-y
- Oishi A, Miyata M, Numa S, Otsuka Y, Oishi M, Tsujikawa A. Wide-field fundus autofluorescence imaging in patients with hereditary retinal degeneration: a literature review. Int J Retina Vitreous. 2019;5(Suppl 1):23. doi:10.1186/s40942-019-0173-z
- Oishi M, Oishi A, Ogino K, et al. Wide-field fundus autofluorescence abnormalities and visual function in patients with cone and cone-rod dystrophies. Invest Ophthalmol Vis Sci. 2014;55(6):3572-3577. doi:10.1167/iovs.14-13912
- Cukras CA, Wong WT, Caruso R, Cunningham D, Zein W, Sieving PA. Centrifugal expansion of fundus autofluorescence patterns in Stargardt disease over time. Arch Ophthalmol. 2012;130(2):171-179. doi:10.1001/archophthalmol.2011.332
- Georgiou M, Kane T, Tanna P, et al. Prospective cohort study of childhood-onset Stargardt disease: fundus autofluorescence imaging, progression, comparison with adult-onset disease, and disease symmetry. Am J Ophthalmol. 2020;211:159-175. doi:10.1016/j.ajo.2019.11.008
- Strauss RW, Kong X, Ho A, et al; ProgStar Study Group. Progression of Stargardt disease as determined by fundus autofluorescence over a 12-month period: ProgStar report no. 11. JAMA Ophthalmol. 2019;137(10):1134-1145. doi:10.1001/jamaophthalmol.2019.2885
- Querques G, Zerbib J, Georges A, et al. Multimodal analysis of the progression of Best vitelliform macular dystrophy. Mol Vis. 2014;20:575-592.
- Parodi MB, Iacono P, Campa C, Del Turco C, Bandello F. Fundus autofluorescence patterns in Best vitelliform macular dystrophy. Am J Ophthalmol. 2014;158(5):1086-1092. doi:10.1016/j.ajo.2014.07.026
- Jarc-Vidmar M, Kraut A, Hawlina M. Fundus autofluorescence imaging in Best’s vitelliform dystrophy. Klin Monbl Augenheilkd. 2003;220(12):861-867. doi:10.1055/s-2003-812555
- Bouzas EA, Karadimas P, Pournaras CJ. Central serous chorioretinopathy and glucocorticoids. Surv Ophthalmol. 2002;47(5):431-448. doi:10.1016/s0039-6257(02)00338-7
- Meyerle CB, Spaide RF. Central serous chorioretinopathy. In: Albert DM, Jakobiec FA, Miller JW, Azar DT, Blodi BA eds. Albert & Jakobiec’s Principles & Practices of Ophthalmology. Saunders; 2008:1871-1880.
- Matsumoto H, Kishi S, Sato T, Mukai R. Fundus autofluorescence of elongated photoreceptor outer segments in central serous chorioretinopathy. Am J Ophthalmol. 2011;151(4):617-623.e1. doi:10.1016/j.ajo.2010.09.031
- Nicholson B, Noble J, Forooghian F, Meyerle C. Central serous chorioretinopathy: update on pathophysiology and treatment. Surv Ophthalmol. 2013 Mar-Apr;58(2):103-126. doi:10.1016/j.survophthal.2012.07.004
- Teke MY, Elgin U, Nalcacioglu-Yuksekkaya P, Sen E, Ozdal P, Ozturk F. Comparison of autofluorescence and optical coherence tomography findings in acute and chronic central serous chorioretinopathy. Int J Ophthalmol. 2014;7(2):350-354. doi:10.3980/j.issn.2222-3959.2014.02.29
- Kleefeldt N, Bermond K, Tarau IS, et al. Quantitative fundus autofluorescence: advanced analysis tools. Transl Vis Sci Technol. 2020;9(8):2. doi:10.1167/tvst.9.8.2
- Prieto PM, McLellan JS, Burns SA. Investigating the light absorption in a single pass through the photoreceptor layer by means of the lipofuscin fluorescence. Vision Res. 2005;45(15):1957-1965. doi:10.1016/j.visres.2005.01.023
- Yung M, Klufas MA, Sarraf D. Clinical applications of fundus autofluorescence in retinal disease. Int J Retina Vitreous. 2016;2:12. doi:10.1186/s40942-016-0035-x