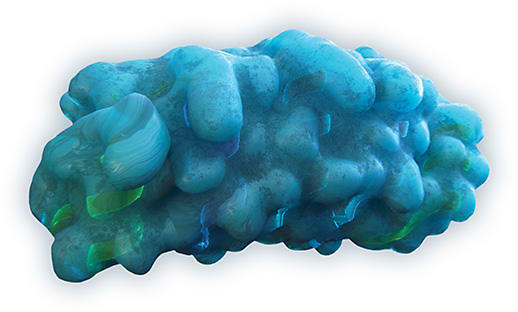
Baruch D. Kuppermann, MD, PhD: The science leading up to the development of therapeutics, such as monoclonal antibodies and DARPin® molecules, an investigational class of binding proteins, is fascinating.1 Around 1900, Paul Ehrlich described the “side chain theory,” which was actually quite prescient given the lack of biochemical information he had on antibodies.2 In the 1970s, Georges J.F. Köhler and César Milstein, building upon some techniques developed by Niels K. Jerne, created the monoclonal antibody technique by fusing antibody-producing B cells with myeloma cells.1 Later, the molecular biology era brought rapid advancements to the field. Moving from mouse to more human-like sequences, chimeric antibodies were developed, grafting mouse variable domains to human constant domains.1,3 Subsequently, humanized antibodies were developed, followed by fully human antibodies using phage display and transgenics.3
Keith Luhrs, PhD: Emerging more recently, DARPin® platform is being investigated for potential use in targeted therapeutics.4 DARPin® molecules are synthetic binding proteins based on naturally occurring ankyrin repeat protein domains (Figure 1).4
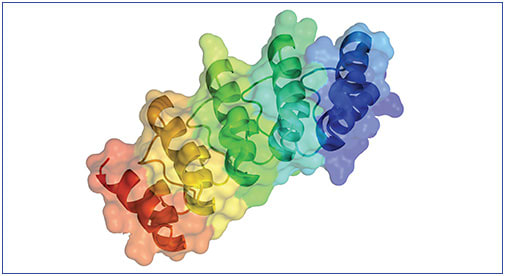
Dr. Kuppermann: DARPin® proteins, along with other types of molecules, are being studied as alternative scaffolds.5 Unlike monoclonal antibodies and antibody fragments, which have been used in several therapeutic areas for more than a decade, these other types of molecules, including DARPin® molecules, are binding proteins that are not derived from antibodies.4,6
Dr. Luhrs: Yes, alternative scaffolds, such as DARPin® molecules, provide a way to create drugs that have different properties from what are available with monoclonal antibodies and antibody fragments, adding to the drug development toolbox. DARPin® platform technology arose from the laboratory of Dr. Andreas Plückthun, one of the leading researchers in the alternative scaffold field, at the University of Zurich. In 2004, scientists who created the technology formed the biopharmaceutical company Molecular Partners to develop the technology for therapeutic use.
How DARPin® Molecules Are Created
Dr. Luhrs: DARPin® molecules are synthetic binding proteins based on naturally occurring ankyrin repeat protein domains.4 Many examples of ankyrin repeats are found in eukaryotic proteins.7 The repeated structures they contain mediate many examples of protein-protein binding interactions that occur in cells.8 These domains are self-compatible stacking units.8 Much of the sequence in these domains is highly similar, so that the backbone structure is maintained.8 However, some segments of each ankyrin repeat domain are different.8 When the repeat domains fold, these variant structures reside on one side of the domain, resulting in the unique binding surfaces that mediate the binding specificity (Figure 2).8
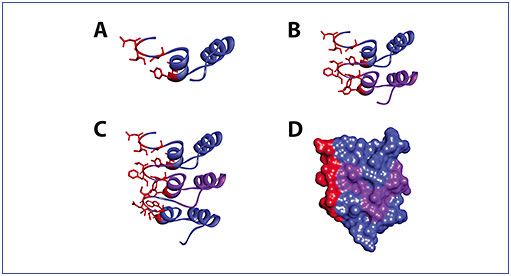
Dr. Kuppermann: How are the unique binding surfaces developed and what creates diversity in their binding potential?
Dr. Luhrs: To create this technology, researchers aligned the sequences of these repeat domains to reveal aminoacids, also known as residues, which are highly conserved among ankyrin repeats and which tend to vary. Thorough understanding of the structure of ankyrin repeat domains, the stacking properties of the domains, and the locations of residues that can be varied enabled the construction of libraries of DARPin® molecules with extremely high binding site diversity.8 Diversity comes from both the varied residues within each repeat as well as by stacking different numbers of different repeats. In addition, the stacking of these domains and the many interactions between the stacked units creates a strong, rigid backbone. So, we have this library of proteins with desirable biophysical properties, stability, and a very large binding site diversity.8
Dr. Kuppermann: What are key similarities and differences between DARPin® molecules and antibodies?
Dr. Luhrs: Naturally occurring binding proteins like antibodies have a structural component like antibodies and synthetic binding proteins. Antibodies, for example, have a structural component, or scaffold, as well as a binding site, which is highly variant and not encoded directly as such in the genome. Both of these features are important for function; the scaffold gives antibodies the physical properties and immune functions they require, while the binding site uniquely directs the molecule to its target.9 DARPin® molecules are similar in that they also have structural scaffold and binding site components.8 The differences arise from the specific properties of their respective scaffolds and the way that the binding sites are generated. And it was only possible to create alternative scaffold proteins, including DARPin® molecules, by understanding how nature had solved the very same challenges we face in making these binding proteins.
Dr. Kuppermann: It’s really quite interesting, and an impressive history from more than 100 years ago. So, how is a specific DARPin® therapeutic created?
Dr. Luhrs: Let’s begin with the end in mind. The ultimate goal of a DARPin® therapeutic discovery campaign is to create a molecule that 1) specifically binds a target, 2) produces a specific mechanism on that target, and 3) has properties, such as stability, solubility, and pharmacokinetics, that will be effective for the particular application. An initial stage in constructing a DARPin® therapeutic is to arrive at a viable set of building blocks, ie, individual DARPin® modules that bind a single target tightly.4 To achieve this, it’s necessary to sample a high diversity of binding sites.8 This is analogous to finding a key to a padlock in a random pile of keys. If the pile of keys is small, it is much less likely to include the key that opens the padlock. However, if the pile of keys is very, very large, it is much more likely to include a key that opens the padlock. Nature has solved this issue in antibodies by not fully assembling them in the inherited genome. Diversity, in this case, results from recombination and somatic hypermutation events during development and the immune response. This is one of the ways that evolution has figured out how to sample a large number of molecules to find tight binders.
In the DARPin® platform discovery process, a highly diverse library is created at the beginning by varying specific residues and mixing and matching different numbers and combinations of these self-compatible stacking units. In general, that high affinity binding site we want preexists in the DARPin® molecule library we screen. Like that key in our pile, we just need to find it.4,6 This differs from other technologies that may frequently require rounds of affinity maturation.
Because we have this huge diversity of potential binders, with perhaps trillions of molecules in the library, we have good chances for high affinity, but this also presents a problem. One-by-one screening of this many molecules would be impossible. Instead, we test in bulk, and we typically accomplish that with ribosome display technology, an in vitro method of selecting binding proteins.
Like other display technologies, this method physically links the nucleic acid encoding for the molecules with their binding activity. Thus, we can fish them out of the library based on binding activity, then recover the information to make them again and go through another cycle to further enrich the pool for binders.4
Dr. Kuppermann: I’m assuming that the process is repeated many times to ultimately select the molecule that binds to the target?
Dr. Luhrs: The process is iterative through a series of steps (Figure 3). From a pool of more than a trillion molecules, we initially narrow to perhaps hundreds to thousands of binders to our target. This is done in bulk and through multiple cycles of “panning” for binders to our target. This reduced number of molecules is now one that can be tested individually in the lab. At this point, we can go beyond binding and evaluate more complex biology, such as mechanism. We know this molecule binds the target, but does it do what we want it to do? Once the number of molecules is further reduced to tens, more time can be spent examining each in greater detail. The last step in the DARPin® platform discovery process is what we call “lead optimization.” Here, engineering can be applied to fine-tune the desired attributes of the molecule. We might add a polymer or other moiety to adjust the pharmacokinetics, or link DARPin® domains that bind different targets to make a multispecific drug. This ability to customize function and properties through assembling components is an important facet of the technology.4,5
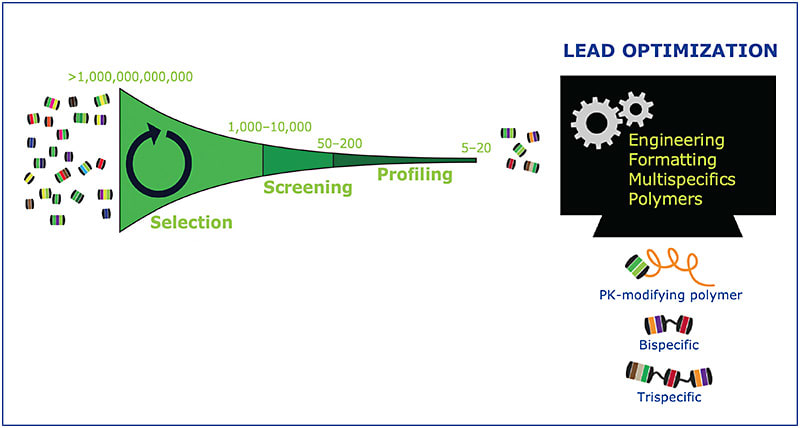
Justis P. Ehlers, MD: Why is the DARPin® platform discovery process attractive from a drug discovery standpoint?
Dr. Luhrs: There are several reasons. One is that the process creates molecules with high binding affinity, stability, and solubility, all of which are favorable properties for a drug. Because the initial outputs are of high quality, we don’t need to spend a lot of time engineering to fix flaws. In general, there are enough good hits that molecules with flaws can be abandoned early in favor of those better-suited to moving forward in the process.4,8
Beyond those advantages, the overall speed of the selection process is rather quick. High-affinity molecules can be identified swiftly. This allows us to rapidly test new ideas by making proof-of-concept molecules. In many cases, we can have molecules with high affinity available to test a particular therapeutic approach in model systems in just a few months.
Key Properties of DARPin® Molecules
Dr. Ehlers: As physicians, we’re familiar with antibody-based therapies and their role in the management of the disease states we treat. Now that we’re learning about DARPin® molecules, what are the key points that differentiate the two that we should keep in mind?
Dr. Luhrs: Each molecule class—antibodies, antibody fragments, and DARPin® molecules—has some inherent properties. These properties differ, which is why it’s helpful for drug developers to have multiple tools in the kit—to allow us to create the right drug for the right application. DARPin® molecules possess a range of valuable properties that we can exploit.
Three key properties of DARPin® molecules are that the molecules have high binding affinity, are engineered for flexibility in molar dose, and are customizable for a range of applications. DARPin® molecules can be customized for pharmacokinetics, multispecifics, and drug conjugates.4,8
High binding affinity
Dr. Luhrs: The biological function of naturally occurring ankyrin repeat domains, as well as DARPin® therapeutic molecules, is to bind to a target. The high target affinity comes from the structure of the molecule, which is very rigid and has a highly complementary binding surface (Figure 4), and the DARPin® platform discovery process that I described earlier. That process queries an immense number of highly diverse binding surfaces to enable finding very high affinity molecules for each target.4,5
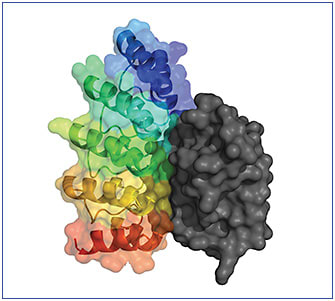
Dr. Ehlers: As we know, antibody-based therapies and DARPin® molecules are tailored to bind targets. Are there specific differences in how they bind?
Dr. Luhrs: In general, yes, so I’ll describe these as class properties. Antibodies have complementarity-determining regions (CDRs), which are more or less flexible loops. Most antibodies bind through an induced fit or conformational selection mechanism, meaning that the CDRs move a bit to fit the target.6
In contrast, DARPin® molecules generally have a rigid structure and bind by a mechanism described as rigid body binding. The binding surface of a DARPin® molecule must be highly complementary, and it typically does not undergo a conformational change in order to bind the target. This may convey somewhat of a entropic thermodynamic advantage. It takes time and energy to move or select for compatible conformations of a molecule; therefore, the lack of the need to undergo that type of change may theoretically contribute to the high affinity.6
Dr. Ehlers: Is the release of antibodies and DARPin® molecules from their targets different? Is it comparable, or do they stay bound the same way and for the same length of time?
Dr. Luhrs: It depends on the specific case, but DARPin® molecules do tend to bind to their targets very quickly. They have a very fast “on-rate.” Because they can have very high affinity, DARPin® molecules also have a slow “off-rate.” It is this binding quickly and releasing slowly that leads to high affinity.6
Engineered for molar dose
Dr. Luhrs: Another key property of DARPin® molecules is they have been engineered for dose flexibility.4 When developing a drug, in some cases, you want a high dose; in others, you want a low dose.
Having a range of choices is the most favorable scenario. When you have high binding affinity, you build the potential dose lower. In effect, you have a high potency molecule, which means you don’t necessarily need a whole lot of the drug to achieve the desired result. In circumstances in which a high dose is desired, several features of DARPin® molecules allow flexibility in this direction.4 One feature is the small size, which allows for a high molar dose with a low mass dose (Figure 5).4
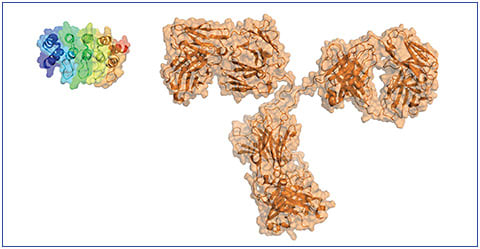
Generally, it is the molar dose we are interested in, ie, how many molecules we are putting in. If you put in a milligram of something large, it is going to be fewer moles of drug, fewer molecules, than if you put in a milligram of something small. Other DARPin® platform features that enable high dosing are solubility and stability.8 Solubility is important because high dosing requires a highly concentrated drug product. Stability is especially important at high concentrations, as well as when the drug is in the patient, both of which can be challenging environments for protein therapeutics.
Highly customizable
Dr. Luhrs: DARPin® molecules are customizable for a range of potential applications (Figure 6).4 Antibodies evolved to fulfill a specific function in biology. Sometimes this aligns well with what we want a drug to do; sometimes it doesn’t. Certainly, there are engineering options to adapt antibodies to different applications.10
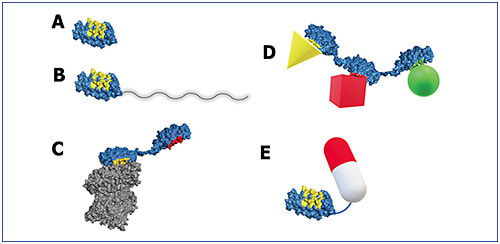
Alternatively, with the DARPin® platform, we begin with a small, stable monomer unit and are able to add different functionalities.4 The technology is very tractable in this respect; the attachment points are well established, so you can assemble more complex structures from these monomer building blocks, with a high confidence of maintaining the desirable qualities of the original. Importantly, for some applications, DARPin® molecules do not have any of the immune effector functions that antibodies have.4
One of the ways we can customize DARPin® therapeutics by assembling these components is to combine multiple DARPin® modules that bind different targets into a single multispecific molecule.4 This gives us the opportunity to approach complex disease biology with a single drug.
Pharmacokinetics are, of course, a very important aspect of drug design. The pharmacokinetics of DARPin® molecules can also be optimized for different applications. For some purposes, a molecule that clears out of the system quickly would be ideal. This might be the case for an imaging agent, or a drug where you want to limit systemic exposure. An unmodified DARPin® molecule normally clears relatively quickly from tissues as well as systemically.11 For other applications, the goal may be to slow diffusion of a therapeutic to extend its half-life within a local tissue. In that case, a polymer can be added to a DARPin® molecule.4
Dr. Ehlers: What are some examples of polymers that can be attached to a DARPin® molecule?
Dr. Luhrs: There are many examples of polymers that can be attached, but the general effect would be similar. The polymer makes the DARPin® molecule larger so that it diffuses more slowly and is therefore eliminated from the tissue more slowly.
In yet another example, if the goal is to create a DARPin® therapeutic that lasts for a long time in systemic circulation, it can be linked with a specific pharmacokinetic-modifying DARPin® molecule, such as one that binds to serum albumin.12 Serum albumin is a large protein that is maintained in the blood. The DARPin® drug in this example would, in effect, be “hitching a ride” on serum albumin and, therefore, persist for a long time in the systemic circulation.12
In addition to the customizations we have already mentioned, we can also envision making drugs where the DARPin® molecules would be linked to different payloads.4 Payloads could include drug conjugates, radionuclides, immunotoxin conjugates, or even imaging agents.4
Dr. Ehlers: What about manufacturing? Is there a difference between manufacturing DARPin® drugs and manufacturing antibody-based drugs?
Dr. Luhrs: In general, the manufacturing processes for DARPin® molecules are similar to other protein-based biologics. The first stage of manufacturing is to have cells express the protein, a process called fermentation. While full-length antibodies are most commonly expressed using a mammalian cell line, DARPin® therapeutics, like many antibody fragments, are typically produced in recombinant E coli. Regardless of the expression system used, in the next stage of manufacturing, the expressed protein must be separated from the host cells and other products using a variety of biochemical separation techniques. While the processes are largely similar, the properties of DARPin® proteins are different from those of antibodies, so the specific conditions will differ.4,13
Dr. Ehlers: Obviously, having a customizable option for the pharmacokinetics of a drug is attractive. Regarding modifications, can you tell us more about the customization manufacturing process?
Dr. Luhrs: Typically, the DARPin® molecules are initially discovered as monomer binding units, as we previously discussed. Then, for example, if we want to add a polymer to change the pharmacokinetics, we can use a chemical reaction to add the modification to a specific site on the protein during the manufacturing process. In other cases, such as creating a bispecific, we can take two independent DARPin® modules and link them as a genetically encoded fusion so that the end result is a single protein comprising two DARPin® modules. So, we first create the building blocks, then assemble the components to get the desired functionality for a particular application.
Dr. Ehlers: The customization possibilities we are discussing, such as making a drug long-acting vs. short-acting and adding polymers, are they separate from the binding characteristics we discussed originally?
Dr. Luhrs: Yes, that has been our experience. I mentioned before that the DARPin® molecules have internal repeats and capping domains on either side. The presumed binding surface is created by the internal repeats, while the end caps can include sites where other components can be attached without interfering with the binding. Therefore, we tend not to see any loss of affinity when assembling the DARPin® modules into more complex structures with multispecificity or addition of these chemical entities.4,12
The Vision for the DARPin® Platform: Expanding the Therapeutic Toolbox
The modular assembly and formatting available with the DARPin® platform can be leveraged to create novel tools to fight disease. The molecule generation engine produces monomer building blocks that tend to maintain their properties all the way through the engineering and optimization.12
Dr. Ehlers: I assume as you are doing this, the complexity increases in terms of testing for other effects and the potential number of targets that may be involved. Have you seen, or has anyone in the field seen, a maximum level of customization? Or is the sky the limit?
Dr. Luhrs: Certainly the more you do, the greater the complexity. We’ve have had very good success putting together molecules with three and four binding domains, as well as adding other customizations, so this is well within reason and still relatively easy to work with.
DARPin® molecules are an investigational class of therapeutics that may be potential alternatives to other types of molecules, such as antibodies and antibody fragments. For example, like an antibody or antibody fragment, a single DARPin® binding module could block a single pathway. However, the DARPin® molecule can have different properties for potentially different applications. In that respect, a DARPin® therapeutic could be an alternative to a conventional therapy to achieve, for example, different exposure or duration.
Disease biology can be complex and we may want to target multiple pathways by blocking multiple targets in parallel with a single molecule. The approach would be to independently make a DARPin® molecule to target A and another DARPin® molecule to target B, then link them through a genetic fusion. The fusion is accomplished with a flexible peptide linker and the result is a two-domain DARPin® molecule in a single polypeptide.4 It is easier to develop a single molecule that attacks two targets than it is to create a mixture of two drugs. There are advantages to making multispecific molecules, which is likely why this has been a very active area of research for alternative scaffold proteins as well as antibody engineering.
Another potential way to use a multispecific DARPin® molecule would be to attack a single target at multiple binding sites, which opens up some new potential mechanisms. This may include novel mechanisms on cell surface targets. Perhaps a receptor binds more than one ligand, or has multiple binding sites. Either scenario can be difficult to target with a single-specificity molecule; however, these situations can be addressed with a multispecific drug. It also may be possible to use a multispecific DARPin® protein to link multiple cellular receptors together.4 In biology, it is common for receptors to be activated by dimerization or higher-order clustering. You can imagine that you could exploit that using the DARPin® platform to create molecules that could act as agonists rather than antagonists.4
In addition, there is a lot of biology that occurs at the interface between two cells. One could envision using the DARPin® platform to direct one cell to attack or signal to another.
There are many possibilities. We’re only at the beginning with this investigational class of biologics. I am excited to see how scientists will apply the DARPin® platform to creating new therapies to benefit patients. ■
References:
- Alkan. Nat Rev Immunol. 2004.
- Bosch and Rosich. Pharmacology. 2008.
- Nelson. MAbs. 2010.
- Stumpp et al. Drug Discov Today. 2008.
- Plückthun. In: Little, ed. Recombinant Antibodies for Immunotherapy; 2009.
- Binz et al. Nat Biotechnol. 2004.
- Al-Khodor et al. Trends Microbiol. 2010.
- Binz et al. J Mol Biol. 2003.
- Schroeder and Cavacini. J Allergy Clin Immunol. 2010.
- Chiu and Gilliland. Curr Opin Struct Biol. 2016.
- Tamaskovic et al. Methods Enzymol. 2012.
- Binz et al. MAbs. 2017.
- Shukla and Thömmes. Trends Biotechnol. 2010.
DARPin® molecules are investigational.
Their efficacy and safety have not been established.
SPONSORED BY Allergan™