Optical coherence tomography angiography (OCTA) is an evolving imaging modality that performs routine structural OCT imaging and also images retinal and choroidal blood flow without the use of exogenous dyes. Compared with fluorescein angiography (FA) and indocyanine green angiography (ICGA), OCTA is a noninvasive technique that is faster, easily repeatable, less expensive to perform, and safer, without the risks of nausea, vomiting, or serious anaphylactic complications.1,2 The modality uses different decorrelation algorithms to detect flow-related changes between repeated B-scans at the same position, and different OCTA instruments use different algorithms.3,4 Because OCT angiograms are obtained from repeated B-scans within a volumetric data set, the routine OCT structural images are co-registered with the vascular images.
There are currently 2 types of OCTA instruments: spectral-domain OCTA (SD-OCTA) and swept-source OCTA (SS-OCTA). Both instruments use Fourier domain detection techniques. Spectral-domain OCT instruments use a broadband near-infrared super luminescent diode as a light source with a center wavelength of approximately 840 nm and a spectrometer as the detector. Swept-source OCTA instruments (Zeiss Plex Elite 9000 and Topcon Triton) use a tunable swept-source laser with a center wavelength of approximately 1,060 nm and a single photodiode detector. Compared with SD-OCTA, the longer wavelength used by SS-OCTA is safer, which means a higher laser power can be used. This results in a better signal-to-noise ratio.5,6 Moreover, the longer wavelength undergoes less scattering by the retinal pigment epithelium (RPE) and there is less sensitivity roll-off of the signal as it penetrates into the choroid. The swept-source laser with its longer wavelength and the higher laser power results in a better signal from the sub-RPE layers, which translates into better detection of both structure and flow information from the vitreous through the choroid in a single volume scan. As a result, the technique known as enhanced-depth imaging, which was used for choroidal imaging with SD-OCT instruments, is no longer needed for SS-OCT imaging of the choroid. Thus, SS-OCTA is superior to SD-OCTA for imaging tissues beneath the RPE.7 When these benefits are combined with the faster scanning speed of at least 100,000 A-scans/second, with the next generation SS-OCT instruments now being deployed into the clinics routinely scanning at 200,000 A-scans per second, it is obvious that for a given acquisition time, SS-OCTA instruments provide faster, deeper, wider, and denser scans compared with conventional SD-OCTA instruments, which scan in the range of 80,000 A-scans per second.8
Swept-source OCTA is now being used as the main imaging modality for the diagnosis and follow-up of retina patients in our clinic. Fluorescein angiography and ICGA are being replaced by SS-OCTA for routine diagnosis and management of vitreoretinal, retinal vascular, chorioretinal, and choroidal vascular diseases. Most importantly, SS-OCTA provides early recognition of pathologic changes and quantitative assessment of these changes in eyes with age-related macular degeneration (AMD) and diabetic retinopathy (DR) in situations where routine FA and ICGA would not usually be performed.
In AMD, SS-OCTA is ideal for diagnosing macular neovascularization (MNV) even before exudation arises. Swept-source OCTA provides better visualization of the neovascular network, choriocapillaris, and choroid compared with FA and ICGA.9 Optical coherence tomography angiography is also able to confirm the absence of MNV when FA is inconclusive due to blockage from subretinal hemorrhage. Unlike dye-based techniques that lack depth resolution, SS-OCTA can localize the MNV by using different boundary specific segmentation strategies.10 While FA may show nonspecific diffuse staining patterns or blockage of hyperfluorescence, SS-OCTA can demonstrate layer specific flow and structural information. Swept-source OCTA is helpful for positively identifying a neovascular network and differentiating vascular from avascular components in macular diseases when other imaging studies may be inconclusive.7,9,11
Although SD-OCTA is able to identify MNV in neovascular AMD, there are limitations in its ability to image neovascular structures below the RPE such as type 1 MNV. A 2017 study using similar segmentation strategies showed that SS-OCTA was better than SD-OCTA in visualizing the full extent of MNV due to its advantage in imaging structures below the RPE.5,7,11 Thus, SS-OCTA provides better visualization of type 1 MNV, and better visualization results in the ability to measure the growth of MNV and its response to treatment once exudation arises. Whether this improved ability to follow the growth and response pattern of MNV after treatment improves retreatment strategies or outcomes remains to be determined.
In addition, SS-OCTA is a useful imaging tool for the diagnosis of patients with central serous retinopathy who are suspected of having MNV.12 Dye-based imaging modalities may be inconclusive in cases of chronic CSR with MNV, whereas studies have shown that SS-OCTA has higher rates of detecting MNV and was helpful in confirming the absence or presence of MNV.13
DETECTION OF SUBCLINICAL NONEXUDATIVE MNV
An important clinical application of SS-OCTA is the detection and monitoring of neovascularization in patients with AMD in the absence of exudation. These subclinical nonexudative lesions are equally prevalent in both intermediate dry AMD and late dry AMD with geographic atrophy (GA).14,15 In the 1990s, abnormal ICGA findings, such as focal hot spots or ICGA plaques, were previously detected in eyes with nonexudative AMD. While these eyes were found to have an increased risk of exudation,16 dye-based angiography could not be justified as a screening tool in these patients due to both costs and risks, especially because no successful treatment had been developed. Clearly the costs and risks significantly outweighed the potential benefit of early detection of MNV. Due to these limitations, angiographic studies on patients with nonexudative AMD were not routinely performed. With the availability of SS-OCTA, subclinical type 1 MNV corresponding to ICGA plaques can now be visualized noninvasively.
A recent study showed that 13% of patients with dry AMD harbor these subclinical lesions. By 12 months of follow-up, the risk of exudation was 15-fold higher in eyes with subclinical MNV compared with eyes without detectable MNV, and 21% of eyes with documented subclinical MNV developed exudation compared with 3.6% of eyes without detectable MNV.15 By 24 months of follow-up, the risk of exudation was 13.6 times greater in eyes with subclinical MNV compared with eyes without MNV and 34.5% of eyes with documented subclinical MNV developed exudation compared with 6.3% of eyes without detectable MNV.17 Some subclinical lesions were noted to grow during the follow-up period. However, growth of these lesions did not necessarily correlate with exudation. It has been postulated that the development of MNV under the RPE may have a protective effect against atrophy, and may provide support for the overlying photoreceptors and RPE.18 As a result, these patients should be followed closely and not treated with vascular endothelial growth factor (VEGF) inhibitors until symptomatic exudation develops. After all, if anti-VEGF therapy is started, when would it ever stop if exudation never develops and the type 1 MNV never regresses? Patients maintain excellent vision if treatment is initiated once symptomatic exudation develops. Moreover, if SS-OCTA can detect changes in the structure or flow characteristics of MNV that predict the development of exudation, then all AMD patients will need to be followed using SS-OCTA. Until these harbingers of exudation can be identified using SS-OCTA imaging, home monitoring and frequent clinic visits every 8 weeks are recommended to detect early signs of exudation. Thus, SS-OCTA allows early diagnosis and the ability to follow these subclinical lesions using both structural and flow OCT information to facilitate early detection and management of exudation as seen in Figure 1.
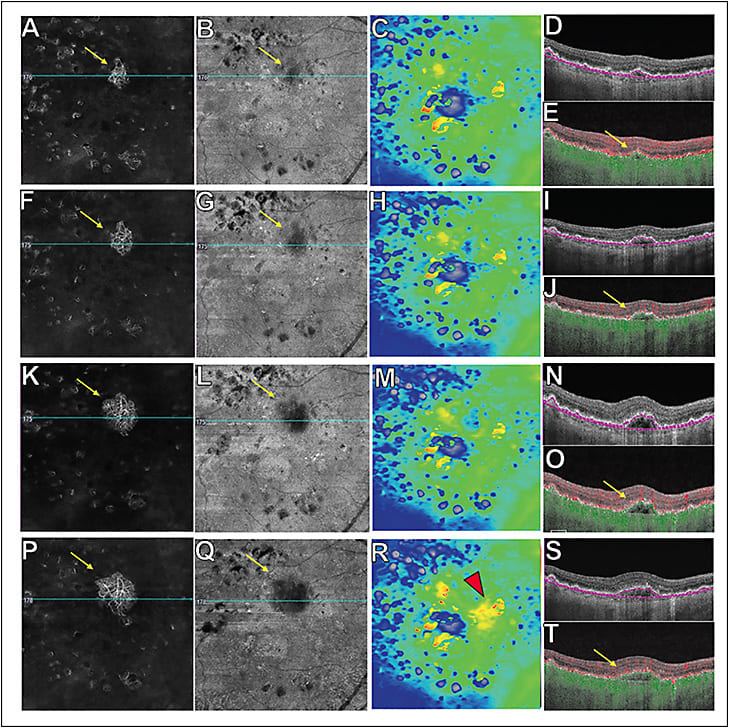
Detection of subclinical MNV is also important when recruiting nonexudative AMD patients into clinical trials so that these eyes at higher risk of exudation can be identified at baseline. This technology allows better understanding of the natural history of nonexudative AMD and the effects of future experimental drugs on those patients.
Standardized criteria need to be defined to help retinal physicians interpret SS-OCTA scans. In clinical practice, 6x6 mm and 12x12 mm scans are performed on all patients. The 12x12 mm scans are helpful in providing a wider field 40-degree view of the fundus at a lower scan density, and the 6x6 mm denser scans provide better image quality when focusing on particular pathologies. However, it is important for the clinician to understand that image interpretation may require hands-on interaction with the OCTA device. Choosing the appropriate segmentation strategy is key to obtaining the correct information from the OCTA image. An initial screening slab is recommended that extends from the outer retina (OR) to the choriocapillaris (CC) to detect any MNV. These segmentation boundaries are referred to as the ORCC slab.19 The next step is to select an appropriate segmentation slab depending on the location of the MNV to obtain the best visualization of the entire neovascular complex. Once the segmentation strategy is chosen, it is important to analyze both the flow and structural SS-OCTA B-scans and en face images. For optimal viewing of type 1 MNV and polypoidal choroidal vasculopathy (PCV), the RPE to RPE-fit (Bruch’s membrane) slab is recommended (Figure 1), and for type 2 and type 3 MNV, the outer plexiform layer (OPL) to RPE slab is recommended. For MNV with both type 1 and type 2 lesions, the ORCC slab is helpful. A boundary-specific segmentation strategy can be used for each lesion component that can be modified accordingly depending on its location for ideal visualization. When using the RPE to RPE-fit slab, the type 1 MNV will show a flow signal on the flow slab that usually appears dark on the corresponding structural slab (Figure 1). This finding is important because it implies that the flow image represents true flow rather than a projection artifact. A bright signal on both en face SS-OCTA flow and structural image may be an artifact and is known as pseudo-flow. This can be due to including too much of the RPE layer in the segmentation of the RPE, which results in the detection of projection artifacts from the retinal circulation. To exclude the possibility that projection artifacts are being confused with the flow from type 1 MNV, it is straightforward to look at the total retinal slab to eliminate flow signals that represent retinal vessels.14 Fortunately, many of the current instruments include projection-artifact removal algorithms.
SS-OCTA IN AMD WITHOUT MNV
With the ability of SS-OCTA to image reliably beneath the RPE, it now becomes possible to visualize the choriocapillaris and the choroid and to study the microvascular changes that occur in normal eyes and AMD eyes, which improves our understanding of disease pathogenesis and paves the way for further research.20 While SS-OCTA imaging of dry AMD without MNV does not impact the management of these patients, it does provide visualization of the choriocapillaris under drusen and an appreciation of the choroid and its thickness.21 A decrease in choriocapillaris density and focal areas of choriocapillaris flow impairment were seen in eyes with early dry AMD compared with age-matched controls.22 In patients with GA, choriocapillaris alteration and flow impairment were seen around regions of GA.23,24 More interestingly, a recent study by our group showed that the global choriocapillaris flow deficit in a 6x6 mm scan correlated better with the enlargement rate of GA compared with the region immediately adjacent to the GA.25 Thus, choriocapillaris flow impairment in GA provides vital information regarding progression of the disease, which may be helpful in managing patients’ expectations. Future studies are being done in the hopes of predicting the direction of atrophic growth, as well as areas where atrophy may develop. While SS-OCTA imaging may be a valuable tool in understanding the natural history of dry AMD, its main value is as a research tool to study the effects of novel therapies for nonexudative AMD.
SS-OCTA IN DIABETIC RETINOPATHY AND OTHER RETINAL VASCULAR DISEASES
Another important clinical use of SS-OCTA is in widefield imaging for the detection of peripheral microvascular changes in DR and other retinal vascular diseases. This is possible due to the development of widefield scans, such as the 12x12 mm and 15x9 mm scans, as well as automated algorithms that can montage these scans. With SS-OCTA, extended field-of-view images are obtained due to the faster scanning rate coupled with eye tracking algorithms that eliminate motion artifacts.26 Hence, SS-OCTA imaging is able to provide excellent widefield vascular detail, which allows for detection of retinal perfusion deficits and neovascularization as shown in Figure 2. Areas of decreased capillary perfusion can be monitored over time. Recent studies have shown that SS-OCTA imaging can detect microvascular anomalies and neovascularization with excellent reliability as long as the pathology is within the field-of-view.27 The vitreoretinal interface slab is able to precisely localize the proliferative changes in DR and monitor neovascular progression (Figure 2). Changes in the flow and structural characteristics of retinal neovascularization can be assessed and monitored serially, and this helps guide treatment strategies.28,29
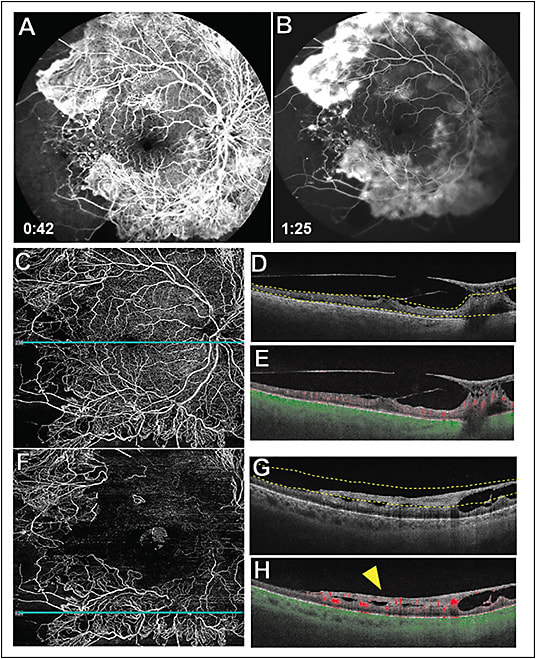
CONCLUSION
This is just the beginning for SS-OCTA imaging. As the technology advances and the instrument costs decrease, retinal practices will evolve to a technology that can reliably image both flow and structural information from the vitreous through the choroid. As the instruments get faster and scans get denser and wider, SS-OCTA will become the standard for imaging in retinal practices. Retina specialists can pinpoint anatomic localization of both flow and structural information by selecting different boundary-specific segmentation slabs using these widefield instruments. Swept-source OCTA represents multimodal imaging in a single imaging modality. RP
REFERENCES
- Kwiterovich KA, Maguire MG, Murphy RP, et al. Frequency of adverse systemic reactions after fluorescein angiography. Results of a prospective study. Ophthalmology. 1991;98(7):1139-1142.
- Yannuzzi LA, Rohrer KT, Tindel LJ, et al. Fluorescein angiography complication survey. Ophthalmology. 1986;93(5):611-617.
- Zhang A, Zhang Q, Chen CL, Wang RK. Methods and algorithms for optical coherence tomography-based angiography: a review and comparison. J Biomed Opt. 2015;20(10):100901.
- Chen CL, Wang RK. Optical coherence tomography based angiography [Invited]. Biomed Opt Express. 2017;8(2):1056-1082.
- Miller AR, Roisman L, Zhang Q, et al. Comparison between spectral-domain and swept-source optical coherence tomography angiographic imaging of choroidal neovascularization. Invest Ophthalmol Vis Sci. 2017;58(3):1499-1505.
- Potsaid B, Baumann B, Huang D, et al. Ultrahigh speed 1050nm swept source/Fourier domain OCT retinal and anterior segment imaging at 100,000 to 400,000 axial scans per second. Opt Express. 2010;18(19):20029-20048.
- Zhang Q, Chen CL, Chu Z, et al. Automated quantitation of choroidal neovascularization: a comparison study between spectral-domain and swept-source OCT angiograms. Invest Ophthalmol Vis Sci. 2017;58(3):1506-1513.
- Mohler KJ, Draxinger W, Klein T, et al. Combined 60 degrees wide-field choroidal thickness maps and high-definition en face vasculature visualization using swept-source megahertz OCT at 1050 nm. Invest Ophthalmol Vis Sci. 2015;56(11):6284-6293.
- Cicinelli MV, Cavalleri M, Consorte AC, et al. Swept-source and spectral domain optical coherence tomography angiography versus dye angiography in the measurement of type 1 neovascularization. Retina. 2019.
- Motulsky EH, Zheng F, Shi Y, Gregori G, Rosenfeld PJ. Anatomic localization of type 1 and type 2 macular neovascularization using swept-source OCT angiography. Ophthalmic Surg Lasers Imaging Retina. 2018;49(11):878-886.
- Novais EA, Adhi M, Moult EM, et al. Choroidal neovascularization analyzed on ultrahigh-speed swept-source optical coherence tomography angiography compared to spectral-domain optical coherence tomography angiography. Am J Ophthalmol. 2016;164:80-88.
- Sulzbacher F, Schutze C, Burgmuller M, Vecsei-Marlovits PV, Weingessel B. Clinical evaluation of neovascular and non-neovascular chronic central serous chorioretinopathy (CSC) diagnosed by swept source optical coherence tomography angiography (SS OCTA). Graefes Arch Clin Exp Ophthalmol. 2019;257(8):1581-1590.
- Wang F, Zhang Q, Deegan AJ, Chang J, Wang RK. Comparing imaging capabilities of spectral domain and swept source optical coherence tomography angiography in healthy subjects and central serous retinopathy. Eye Vis (Lond). 2018;5:19.
- Roisman L, Zhang Q, Wang RK, et al. Optical coherence tomography angiography of asymptomatic neovascularization in intermediate age-related macular degeneration. Ophthalmology. 2016;123(6):1309-1319.
- de Oliveira Dias JR, Zhang Q, Garcia JMB, et al. Natural history of subclinical neovascularization in nonexudative age-related macular degeneration using swept-source OCT angiography. Ophthalmology. 2018;125(2):255-266.
- Hanutsaha P, Guyer DR, Yannuzzi LA, et al. Indocyanine-green videoangiography of drusen as a possible predictive indicator of exudative maculopathy. Ophthalmology. 1998;105(9):1632-1636.
- Yang J, Zhang Q, Motulsky EH, et al. Two-year risk of exudation in eyes with non-exudative amd and subclinical neovascularization detected with swept source OCT angiography. Am J Ophthalmol. 2019.
- Capuano V, Miere A, Querques L, et al. Treatment-naive quiescent choroidal neovascularization in geographic atrophy secondary to nonexudative age-related macular degeneration. Am J Ophthalmol. 2017;182:45-55.
- Zhang Q, Wang RK, Chen CL, et al. Swept source optical coherence tomography angiography of neovascular macular telangiectasia type 2. Retina. 2015;35(11):2285-2299.
- Choi W, Mohler KJ, Potsaid B, et al. Choriocapillaris and choroidal microvasculature imaging with ultrahigh speed OCT angiography. PLoS One. 2013;8(12):e81499.
- Lane M, Moult EM, Novais EA, et al. Visualizing the choriocapillaris under drusen: comparing 1050-nm swept-source versus 840-nm spectral-domain optical coherence tomography angiography. Invest Ophthalmol Vis Sci. 2016;57(9):OCT585-590.
- Waheed NK, Moult EM, Fujimoto JG, Rosenfeld PJ. Optical coherence tomography angiography of dry age-related macular degeneration. Dev Ophthalmol. 2016;56:91-100.
- Choi W, Moult EM, Waheed NK, et al. Ultrahigh-speed, swept-source optical coherence tomography angiography in nonexudative age-related macular degeneration with geographic atrophy. Ophthalmology. 2015;122(12):2532-2544.
- Moult EM, Waheed NK, Novais EA, et al. Swept-source optical coherence tomography angiography reveals choriocapillaris alterations in eyes with nascent geographic atrophy and drusen-associated geographic atrophy. Retina. 2016;36 Suppl 1:S2-S11.
- Thulliez M. Correlations between choriocapillaris flow deficits around geographic atrophy and enlargement rates based on swept source OCT imaging. J Fr Ophtalmol. 2018;41(6):569-570.
- Zhang Q, Rezaei KA, Saraf SS, Chu Z, Wang F, Wang RK. Ultra-wide optical coherence tomography angiography in diabetic retinopathy. Quant Imaging Med Surg. 2018;8(8):743-753.
- Russell JF, Flynn HW, Jr., Sridhar J, et al. Distribution of diabetic neovascularization on ultra-widefield fluorescein angiography and on simulated widefield OCT angiography. Am J Ophthalmol. 2019. [Epub ahead of print]
- Russell JF, Shi Y, Hinkle JW, et al. Longitudinal wide-field swept-source OCT angiography of neovascularization in proliferative diabetic retinopathy after panretinal photocoagulation. Ophthalmol Retina. 2019;3(4):350-361.
- Elbendary AM, Abouelkheir HY. Bimodal imaging of proliferative diabetic retinopathy vascular features using swept source optical coherence tomography angiography. Int J Ophthalmol. 2018;11(9):1528-1533.