Current retinal imaging methods primarily focus on optical imaging of anatomy. However, anatomic changes are the end result of complex upstream molecular changes. Ocular imaging is shifting from providing not only anatomical but also functional information on the eye, enabling diagnosis and detection of various diseases at an earlier stage. Current retinal imaging technologies include fluorescein angiography (FA), fundus photography, indocyanine green angiography (ICGA),6-8 scanning laser ophthalmoscopy (SLO), adaptive optics, fundus autofluorescence, fluorescence lifetime imaging ophthalmoscopy (FLIO), optical coherence tomography (OCT), and OCT angiography (OCTA).6,9 Each modality has advantages and disadvantages. Optical coherence tomography can provide noninvasive imaging of intraocular structures with high resolution.10 Fluorescein angiography is the gold standard for retinal vascular imaging to evaluate leakage and microaneurysms, but it involves an invasive injection of exogenous contrast agents.10 These imaging modalities depend on the detection of back-scattering light from the retinal tissues or exogenous contrast agents, which limits the penetration depth and visualization of the choroidal vasculature.
Recent advances in laser and optical imaging have enabled the development of photoacoustic microscopy (PAM) to visualize retinal and choroidal vasculature and tissues. Photoacoustic microscopy is a novel, noninvasive, nonionizing, hybrid, high-resolution imaging technology. It can offer anatomic, functional, and molecular retinal characterizations with excellent resolution and contrast, ultrahigh sensitivity, and a great depth of penetration to both the retina and choroid. It enables visualization of oxygenated and deoxygenated hemoglobin and melanin in retinal vessels, neovascularization, the choroidal layer, and the retinal pigment epithelium. Photoacoustic microscopy can be integrated in multimodal systems, including integration with OCT, fluorescence microscopy, or SLO. The combination of these modalities can provide several advantages and can be co-registered to one another to better evaluate disease. Photoacoustic microscopy has been demonstrated in preclinical human-relevant animal models.
Photoacoustic microscopy converts light into sound. In PAM, the targeted tissue is irradiated by a short pulsed laser (3-5 ns) to induce an acoustic wave that is detected by an ultrasound sensor. The major advantage of PAM imaging is that it can assess both structural and functional information of blood vessels without an exogenous contrast agent.11-15 These capabilities are significant and highly desirable for imaging the eyes.
In ophthalmology, PAM platforms have been studied since. 2010.11-13 Early systems were only described for small animals, for which the axial length of the eyeball is significantly smaller than that of humans. Due to the attenuation of the PA sound signal based on the distance from the sound source, the retina, this limited clinical application.
Tian et al introduced a multimodal PAM and OCT system that can image both retinal and choroidal vessels in rabbits with high resolution and high contrast.16 This system can achieve high lateral resolution of 4.1 µm for PAM and 3.8 µm for OCT, and axial resolution of 37.0 µm for PAM and 4.0 µm for OCT. With this high partial resolution, the system is suitable to detect single capillaries. In addition, the axial length of rabbit eyes is quite similar to that of humans, enabling possible translation of this system to clinical applications.17
PHOTOACOUSTIC MICROSCOPY OF THE EYE
Photoacoustic microscopy can be used to detect endogenous absorbers, such as hemoglobin and melanin.18-23 Photoacoustic microscopy uses a short-pulse laser light as an excitation source and an ultrasound transducer to acquire the photoacoustic (PA) signal in tissue. The laser light is absorbed by chromophores like melanin or hemoglobin and results in a rapid localized temperature increase and subsequent thermoelastic expansion. This expansion generates PA waves, which are detected by an ultrasound transducer in contact with the conjunctiva of the eye. The recorded PA signal is used to reconstruct 2-dimensional (2D) or 3-dimensional (3D) images. Two types of information about the sample can be obtained from the acoustic wave. A single laser pulse excitation at a fixed position creates a 1-dimensional depth-resolved PA image along the z axis, referred to as an A-line. By scanning along the x-axis of a sample, a 2D depth-sensitive PA image is acquired. The pulsed laser is then scanned along the x- and y-axes across the sample to obtain 3D volumetric PA images. The PA amplitude depends on the optical absorption of the chromophores, as shown in Figure 1A. In addition, spectroscopic PA imaging is performed to determine the concentration of chromophore or to quantify the suitable excitation wavelength for distinguishing between normal and abnormal tissues.19,24 Due to high optical absorption properties within the visible light, PA imaging has the potential to precisely measure the variations in retinal blood oxygen saturation as well as the concentration of blood and melanin.11,14,25 Figures 1B and 1C show the schematic and physical setup of multimodal PAM and OCT for label-free imaging of retinal vessels.26 This technique uses a 570-nm laser pulse as a light source to generate the PA waves. Laser-induced PA signals are detected by a custom-built unfocused needle ultrasonic transducer with a central frequency of 27 MHz, 16 MHz bandwidth, and field of view of 4x4 mm2. The lateral and axial resolution of the system were 37.0 µm and 4.1 µm, respectively.
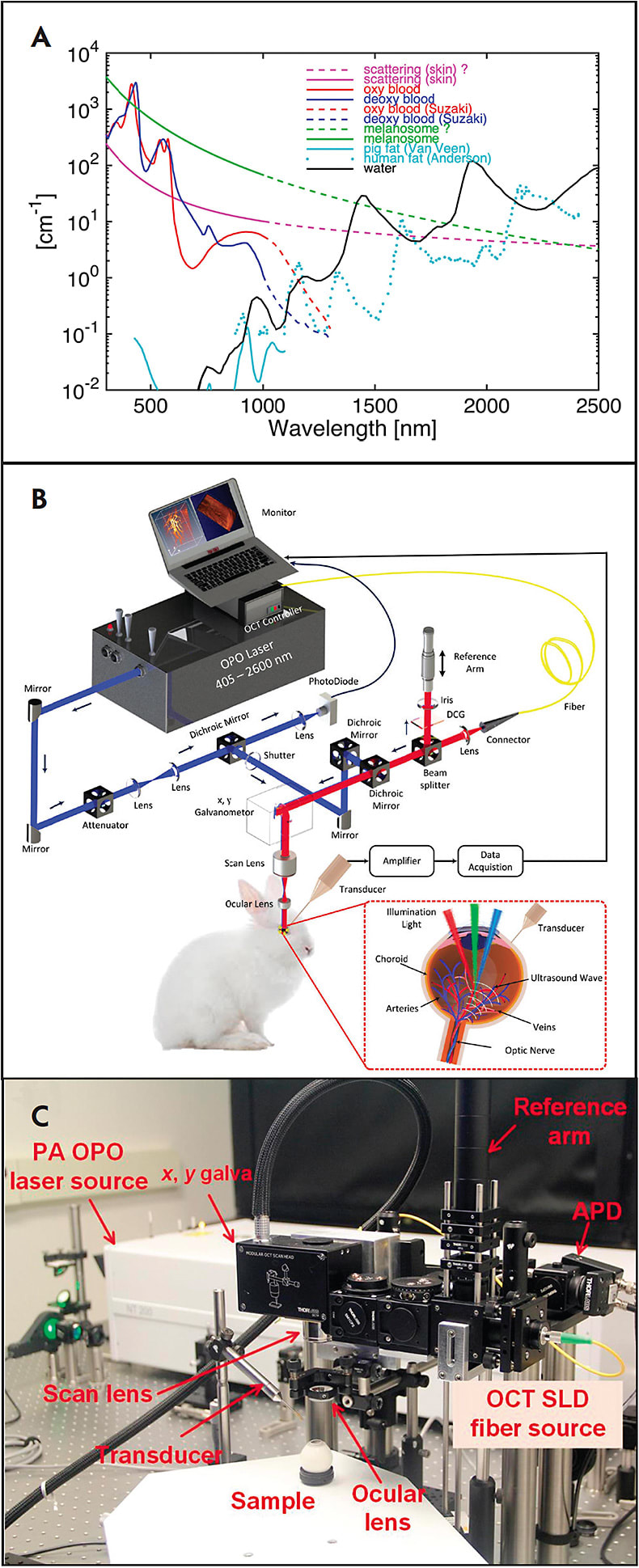
To acquire high-resolution PAM images without damaging sensitive neural tissue of the eye, the laser light energy used must be below the American National Standards Institute safety limit standard (ie, 160 nJ at 532-700 nm) 27-31 Rapid acquisition is also required to avoid motion artifacts, because the eye has a fixation time of approximately 500 ms.31
PHOTOACOUSTIC MICROSCOPY OF RODENT RETINA
Photoacoustic microscopy imaging has been used to image hemoglobin in the retinal and choroidal blood vessels as well as melanin in the RPE. Xie et al developed a PAM system to measure the optical absorption properties of retinal structures.26 The authors used a 533-nm laser pulse as a light source to generate PA waves. Lateral resolution was 20 µm and axial resolution was 23 µm, and en face images of individual capillaries were visualized in the albino mouse retina. Several groups have successfully applied PAM imaging platforms to evaluate ocular tissues, including retinal vasculature, choroidal vasculature, and RPE melanin.32-36 Song et al developed a multimodal ocular imaging system by combining PAM, OCT, fluorescence microscopy (FM), and adaptive-optics-based SLO.37 In their system, both PAM and SLO used the same laser source. Fluorescence microscopy uses an additional laser source at the wavelength of 488 nm. This approach provides strong optical absorption, optical backscattering, and fluorescence properties of the retina.
Unfortunately, these studies could only visualize normal vessels in the eye. Another study reported by Dai et al used integrated PAM and OCT to visualize laser-induced choroidal neovascularization (CNV) in mice (Figure. 2).2 In their experiments, the authors used laser light at 532 nm (pulse duration less than 2 ns and repetition rate over 10 kHz) to illuminate inside the eye. The laser light (~60 nJ) was delivered to a galvanometer using a single mode optical fiber before focus onto the retina. The acoustic signal was detected by a 30 MHz ultrasound transducer. This PAM system has a lateral resolution of 20 µm and axial resolution of 40 µm. The acquisition time is about 13 s to obtain a 3D volumetric PAM image. The CNV was induced by illumination of laser light at high power of 200 mW with exposure duration of 100 ms and laser beam size of 50 µm. The red dotted square box shows the position of developed CNV obtained by the PAM system.
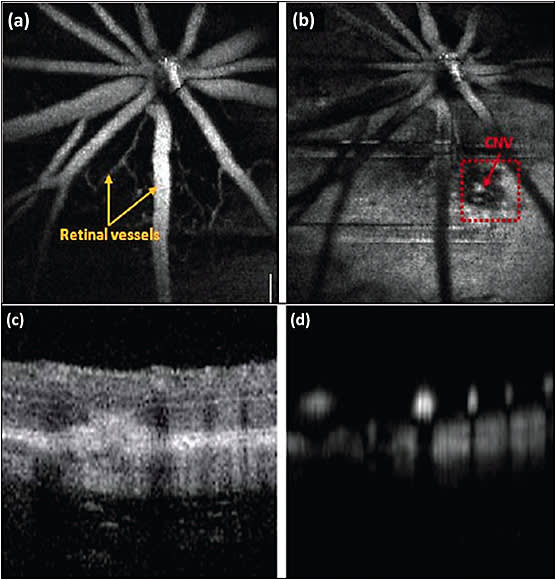
PHOTOACOUSTIC MICROSCOPY OF THE RETINA IN LARGE ANIMALS
To overcome the limitations of visualizing rodent eyes, Tian et al demonstrated a multimodal imaging platform that integrated PAM and spectral-domain OCT (SD-OCT) for label-free imaging of the retina in rabbits.28,29,38 This system used a single-element needle-shape ultrasound transducer in contact with conjunctiva to detect the laser-induced PA signals. This system was able to image retinal and choroidal vessels and capillaries in vivo in living rabbits with high temporal and spatial resolution.17,30,38 Figure 3 shows PAM and OCT obtained in vivo in rabbit retinal and choroidal vessels. To achieve the high contrast of blood vessels, the PA images were obtained at a peak absorption of hemoglobin at the wavelength of 570 nm.39
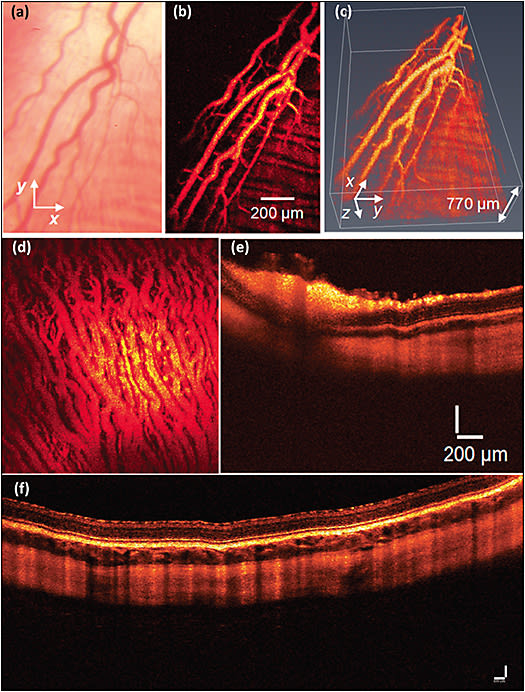
Nguyen et al employed multimodal PAM and OCT to detect both healthy retinal vessels and laser-induced retinal neovascularization in living rabbits.29 This study can detect single microvessels with a diameter of approximately 30 µm. In another study, Nguyen et al showed that integrated PAM and OCT was also adapted to image RNV in a rabbit model.28,31 Newly developed RNV was clearly visualized using multimodal PAM and OCT with high contrast and high resolution in both 2 dimensions and 3 dimensions. However, the limitation of these experiments is that it is hard to distinguish native retinal vessels from RNV. To classify between native and RNV vessels, the authors performed semisegmentation. To better visualize the RNV network, Tian et al built upon this work and added FM to the PAM system to develop a triple PAM, SD-OCT, and FM imaging platform.16
By acquiring a signal at different optical wavelengths, PAM has the potential to measure the concentration of melanin, blood flow, and oxygen saturation in vasculature. Hariri et al described a high-speed multimodal PA and ultrasound imaging to detect chorioretinal oxygen gradient in an in vivo model of hypoxia as well as an ischemia reperfusion model on live rabbits.40 In this study, PA imaging was integrated with high-frequency ultrasound with a tunable laser having pulse width of 6 ns and pulse repetition rate of 20 Hz with wavelengths of 750 and 850 nm to measure the retinal and choroidal oxygen saturation.39
CONTRAST AGENTS
Although PAM imaging systems can be used for imaging the retinal and choroidal vasculature, CNV, and RNV without using a contrast agent, it is hard to accurately distinguish new developed microvasculature from native vessels. Exogenous contrast agent has been investigated to boost the sensitivity and enhance PAM image contrast and to perform molecular imaging. Several exogenous contrast agents, including Cy7 fluorophore, colloidal gold nanoparticles, and gold nanorods, have been explored for improved blood vessel detection using PAM techniques.39-42
CLINICAL CHALLENGES AND FUTURE DEVELOPMENT
Photoacoustic microscopy imaging is a promising tool for anatomical, functional, and molecular imaging of retinal diseases. Various retinal pathologies can be detected using PAM system, with high resolution and deep tissue penetration. This could aid researchers and clinicians to better understand retinal disease. Photoacoustic microscopy is still not approved by the FDA for use in patients. Currently, the laser energy used for PAM ranges from 40 nJ to 80 nJ for retinal imaging, which is 50% to 75% lower than the American National Standards Institute safety limit. Thus, it can be regarded as safe. However, further safety evaluations should be conducted before applying this imaging technique on humans. Long-term safety studies of both structure and functional assessment of vision should be performed.
To bring PAM imaging to the clinic, there are several limitations to overcome. The first is the coupling between the ultrasound transducer and the eye. Currently, most PAM systems use a transducer in contact with the conjunctiva of the eye to maximize the detection of PA signal or directly coupled ultrasound transducer with the eyelid using ultrasonic gel. Unfortunately, these coupling methods are not ideal. It may cause patient discomfort and motion artifacts. Non-contact ultrasound detectors are needed to overcome these issues. Noncontact remote optical detection of the sound waves could be employed using optical interferometry to detect the PA signal.41-45
The second limitation is acquisition time and lateral and axial resolutions. An optical PAM system requires ultrahigh axial and lateral resolution to resolve retinal capillaries. Currently, the lateral resolution of PAM is about 20 µm to 37 µm,3,5,27,37,46 which is not enough to distinguish single capillaries with a diameter less than 10 µm. To improve lateral resolution, a synthetic-aperture technique should be investigated. Acquisition time may be improved by switching to a higher laser repetition rate.
The third limitation is that multimodal imaging acquires single images from a single imaging modality and then co-registers these images on the same imaging plane, requiring further post-processing. In addition, to measure and observe blood flow in the retinal vasculature, the PAM system must perform this rapidly. Therefore, PAM of the eye needs further investigation to achieve real-time imaging.
CONCLUSION
Photoacoustic signals are directly correlated with hemoglobin absorption, and spectroscopic PAM techniques make it possible to quantify functional information of the retina. Accurate quantification of hemoglobin concentrations is essential to measuring oxygen saturation. Photoacoustic microscopy contrast agents have potential application for improved visualization of the retinal and choroidal microvasculature, RNV, and CNV. Future developments and the applicability for clinical translation offered by PAM may lead to a new diagnostic technique for retinal diseases. RP
REFERENCES
- Taruttis A, Ntziachristos V. Advances in real-time multispectral optoacoustic imaging and its applications. Nat Photonics. 2015;9:219-227.
- Dai C, Li L, Liu W, Wang F, Zhou C. In vivo time-serial evaluation of laser-induced choroidal neovascularization in rats simultaneously using photoacoustic microscopy and optical coherence tomography. Proc SPIE Int Soc Opt Eng. 2018;10494:1049469.
- Nguyen VP, Li Y, Zhang W, Wang X, Paulus YM. Multi-wavelength, en-face photoacoustic microscopy and optical coherence tomography imaging for early and selective detection of laser induced retinal vein occlusion. Biomed Opt Expess. 2018;9(12):5915-5938.
- Nguyen VP, Li Y, Zhang W, Wang X, Paulus YM. High-resolution multimodal photoacoustic microscopy and optical coherence tomography image-guided laser induced branch retinal vein occlusion in living rabbits. Sci Rep. 2019;9(1):1-14.
- Nguyen V, Li Y, Aaberg M, Zhang W, Wang X, Paulus Y. In vivo 3D imaging of retinal neovascularization using multimodal photoacoustic microscopy and optical coherence tomography imaging. J Imaging. 2018;4(12):150.
- Coscas F, Glacet-Bernard A, Miere A, et al. Optical coherence tomography angiography in retinal vein occlusion: evaluation of superficial and deep capillary plexa. Am J Ophthalmol. 2016;161:160-171.
- Prasad PS, Oliver SC, Coffee RE, Hubschman JP, Schwartz SD. Ultra wide-field angiographic characteristics of branch retinal and hemicentral retinal vein occlusion. Ophthalmology. 2010;117(4):780-784.
- McAllister IL, Yu DY, Vijayasekaran S, Barry C, Constable I. Induced chorioretinal venous anastomosis in experimental retinal branch vein occlusion. Br J Ophthalmol. 1992;76(10):615-620.
- Soetikno BT, Shu X, Liu Q, et al. Optical coherence tomography angiography of retinal vascular occlusions produced by imaging-guided laser photocoagulation. Biomed Opt Expess. 2017;8(8):3571-3582.
- Li Y, XX, Paulus YM. Novel retinal imaging technologies. Int J Opthalmol Eye Sci. 2017;5(7):1-5.
- Song W, Wei Q, Liu W, et al. A combined method to quantify the retinal metabolic rate of oxygen using photoacoustic ophthalmoscopy and optical coherence tomography. Sci Rep. 2014;4:6525.
- Hennen SN, Xing W, Shui YB, et al. Photoacoustic tomography imaging and estimation of oxygen saturation of hemoglobin in ocular tissue of rabbits. Exp Eye Res. 2015;138:153-158.
- Ning B, Kennedy MJ, Dixon AJ, et al. Simultaneous photoacoustic microscopy of microvascular anatomy, oxygen saturation, and blood flow. Opt Lett. 2015;40(6):910-913.
- Yao J, Maslov KI, Zhang Y, Xia Y, Wang LV. Label-free oxygen-metabolic photoacoustic microscopy in vivo. J Biomed Opt. 2011;16(7):076003.
- Chen Z, Yang S, Xing D. In vivo detection of hemoglobin oxygen saturation and carboxyhemoglobin saturation with multiwavelength photoacoustic microscopy. Opt Lett. 2012;37(16):3414-3416.
- Tian C, Zhang W, Nguyen VP, Huang Z, Wang X, Paulus YM. Integrated photoacoustic microscopy, optical coherence tomography, and fluorescence microscopy for multimodal chorioretinal imaging. Proc SPIE Int Soc Opt Eng. 2018;104945U.
- Hughes A. A schematic eye for the rabbit. Vis Res. 1972;12(1):123-138.
- Nguyen VP, Park S, Oh J, Wook Kang H. Biocompatible astaxanthin as novel contrast agent for biomedical imaging. J Biophotonics. 2017;10(8):1053-1061.
- Nguyen VP, Oh J, Park S, Wook Kang H. Feasibility of photoacoustic evaluations on dual‐thermal treatment of ex vivo bladder tumors. J Biophotonics. 2017;10(4):577-588.
- Wang LV. Multiscale photoacoustic microscopy and computed tomography. Nat Photonics. 2009;3(9):503-509.
- Wang LV, Hu S. Photoacoustic tomography: in vivo imaging from organelles to organs. Science. 2012;335(6075):1458-1462.
- Luke GP, Yeager D, Emelianov SY. Biomedical applications of photoacoustic imaging with exogenous contrast agents. Ann Biomed Eng. 2012;40(2):422-437.
- Beard P. Biomedical photoacoustic imaging. Interface Focus. 2011;1(4)602-631.
- Sun Y, O’Neill B. Imaging high-intensity focused ultrasound-induced tissue denaturation by multispectral photoacoustic method: an ex vivo study. Appl Opt. 2013;52(8):1764-1770.
- Hu S, Rao B, Maslov K, Wang LV. Label-free photoacoustic ophthalmic angiography. Opt Lett. 2010;35(1):1-3.
- Xie D, Li Q, Gao Q, Song W, Zhang HF, Yuan X. In vivo blind‐deconvolution photoacoustic ophthalmoscopy with total variation regularization. J Biophotonics. 2018;11(9):e201700360.
- Nguyen VP, Li Y, Qian W, et al. Contrast agent enhanced multimodal photoacoustic microscopy and optical coherence tomography for imaging rabbit choroidal and retinal vessels in vivo. Sci Rep. 2019;9(1):5945.
- Nguyen VP, Li Y, Henry J, et al. Integrated photoacoustic microscopy and optical coherence tomography image-guided laser induced branch retinal vein occlusion in living rabbits. Paper presented at: Multimodal Biomedical Imaging XIV; 2019; San Francisco, CA.
- Nguyen VP, Li Y, Henry J, et al. Multi-wavelength photoacoustic microscopy for detection of retinal vein occlusion during laser photocoagulation in rabbits. Paper presented at: Label-free Biomedical Imaging and Sensing (LBIS); 2019; San Francisco, CA.
- Tian C, Zhang W, Nguyen VP, Wang X, Paulus YM. Novel photoacoustic microscopy and optical coherence tomography dual-modality chorioretinal imaging in living rabbit eyes. J Vis Exp. 2018;132:10.3791/57135.
- Zhang W, Li Y, Nguyen VP, et al. High-resolution, in vivo multimodal photoacoustic microscopy, optical coherence tomography, and fluorescence microscopy imaging of rabbit retinal neovascularization. Light Sci Appl. 2018;7:103.
- de La Zerda A, Paulus YM, Teed R, et al. Photoacoustic ocular imaging. Opt Lett. 2010;35(3):270-272.
- Hu Z WX, Liu Q, Paulus YM. Photoacoustic imaging in ophthalmology. Int J Opthalmol Eye Sci. 2015;8:126-132.
- Jiao S, Jiang M, Hu J, et al. Photoacoustic ophthalmoscopy for in vivo retinal imaging. Opt Express. 2010;18(4):3967-3972.
- Silverman RH, Kong F, Chen YC, et al. High-resolution photoacoustic imaging of ocular tissues. Ultrasound Med Biol. 2010;36(5):733-742.
- Kim H, Nguyen VP, Manivasagan P, et al. Doxorubicin-fucoidan-gold nanoparticles composite for dualchemo-photothermal treatment on eye tumors. Oncotarget. 2017;8(69):113719-113733.
- Song W, Wei Q, Liu T, et al. Integrating photoacoustic ophthalmoscopy with scanning laser ophthalmoscopy, optical coherence tomography, and fluorescein angiography for a multimodal retinal imaging platform. J Biomedical Opt. 2012;17(6):061206.
- Tian C, Zhang W, Mordovanakis A, Wang X, Paulus YM. Noninvasive chorioretinal imaging in living rabbits using integrated photoacoustic microscopy and optical coherence tomography. Opt Epxress. 2017;25:15947-15955.
- Prahl S. Optical absorption of hemoglobin. 2012. Available at: http://omlc.org/spectra/hemoglobin .
- Hariri A, Wang J, Kim Y, Jhunjhunwala A, Chao DL, Jokerst JV. In vivo photoacoustic imaging of chorioretinal oxygen gradients. J Biomed Opt. 2018;23(3):036005.
- Chen SL, Xie Z, Ling T, Guo LJ, Wei X, Wang X. Miniaturized all-optical photoacoustic microscopy based on microelectromechanical systems mirror scanning. Opt Lett. 2012;37(20):4263-4265.
- Xie Z, Chen SL, Ling T, Guo LJ, Carson PL, Wang X. Pure optical photoacoustic microscopy. Opt Express. 2011;19(10):9027-9034.
- George D, Lloyd H, Silverman RH, Chitnis PV. A frequency‐domain non‐contact photoacoustic microscope based on an adaptive interferometer. J Biophotonics. 2018;11(6):e201700278.
- Sampathkumar A, Chitnis PV, Silverman RH. All-optical photoacoustic microscopy (AOPAM) system for remote characterization of biological tissues. In Proceedings of Photons Plus Ultrasound: Imaging and Sensing. 2014; p. 89432Y.
- Xu G, Wang C, Feng T, Oliver DE, Wang X. Non-contact photoacoustic tomography with a laser Doppler vibrometer. In Proceedings of Photons Plus Ultrasound: Imaging and Sensing. 2014;894332.
- Liu W, Zhang HF. Photoacoustic imaging of the eye: a mini review. Photoacoustics. 2016;4(3):112-123.