The restoration of sight to the blind has long been considered miraculous — literally. Restoring sight to the blind was cited in the sanctification of saints throughout history. Although ophthalmologists in the United States have become somewhat inured to the nearly miraculous vision restoration provided by cataract surgery and corneal transplantation, the idea that blindness from retinal degenerative disease may be reversible still seems the stuff of science fiction. But research in the past decade has brought this possibility to the brink of the clinic.
Two of the leading causes of blindness — age-related macular degeneration (AMD) and retinitis pigmentosa (RP) — both arise secondary to death of the photoreceptors. The remainder of the retina, however, remains largely intact. The bipolar cells and retinal ganglion cells of the retina still form functional connections. As the mapping of retinal ganglion cells to the visual world remains “hard wired,” if light sensitivity could be conferred upon the ganglion cells, it should be possible to restore something like vision to the blind individual. This is the principle used by optoelectronic implants, such as the Argus II (Second Sight). This 60-electrode epiretinal device, which was approved by the FDA in 2013, functioned by transducing images from an attached camera to the electrodes. Patients receiving the implant noted visual phosphenes that permitted discrimination of certain images.1 Utility was limited by the low resolution of the device, and implantation was technically challenging. The company has since prioritized a cortical stimulation approach to vision restoration.
While other, higher resolution devices, such as subretinal photovoltaic implants,2 offer the potential for higher resolution restoration, these devices will be limited at present to a relatively small visual field that is determined by the physical size of the device. Nonetheless, these devices have established that stimulation of retinal ganglion cells in patients with outer retinal degeneration can restore some degree of form vision.
Two other approaches — opsin gene therapy and small-molecule photoswitches — also represent potential means for conferring light sensitivity on ganglion cells, but they offer the possibility of much higher resolution while obviating the need for device development and placement. Both techniques rely on converting the ganglion cells or upstream cells of the retina into functional photoreceptors. In the case of gene therapy, this is done via transgenic expression of photoreceptors in the ganglion cells using a viral vector, while photopharmacology technology employs small-molecule “photoswitch” molecules that act as molecular light switches to turn cell firing on and off.
VISION RESTORATION THROUGH OPSIN GENE THERAPY
The origins of the gene therapy approach date back to the discovery of channel opsins. The opsin molecules that underlie vision in humans have ancient ancestral roots. Although human cone opsin and rhodopsin molecules are seven-transmembrane G-protein coupled receptors, the earliest opsins did not require a separate G-protein for signaling, but were directly coupled to ion channels. These opsins are known as channelopsins, and they may be linked to ion channels gating cations (positive charge) or anions (negative charge). Modern-day single-cell organisms still use these proteins to drive activities like light-dependent movement. Two proteins widely used for this are the cation channel channelopsin3 (typically derived from the algae Chlamydomonas) and anion channel halorhodopsin (derived from the archaean bacteria Natronomonas). The genes encoding these proteins have been cloned, and when expressed in mammalian neurons, confer light sensitivity upon the cells expressing them. This technology has been widely used in experimental biology, where this technique goes by the term optogenetics. These genes can also be cloned into viral vectors such as adeno-associated virus (AAV). Pan et al first showed that such viruses could be used to infect the retina of mice with outer retinal degeneration; following transduction, the animals regained pupillary light responses and behavioral responses to light.4 Busskamp et al showed that halorhodopsins could be virally introduced into degenerating cone pedicles to simulate the hyperpolarization that occurs naturally with light in intact cones; again, light responses could be restored in animals with hereditary retinal degeneration.5 Early generation channel-opsins suffered from low-light sensitivity but more recent versions are markedly improved and show a broader range of spectral sensitivity.6 This technology was commercialized by the company RetroSense, which packaged channelrhodopsin-2 in an AAV vector, and launched a phase 1/2 clinical trial for use in retinitis pigmentosa in 2016 (NCT02556736). The company was acquired by Allergan in 2016; to date, no preliminary data from the study have been released.
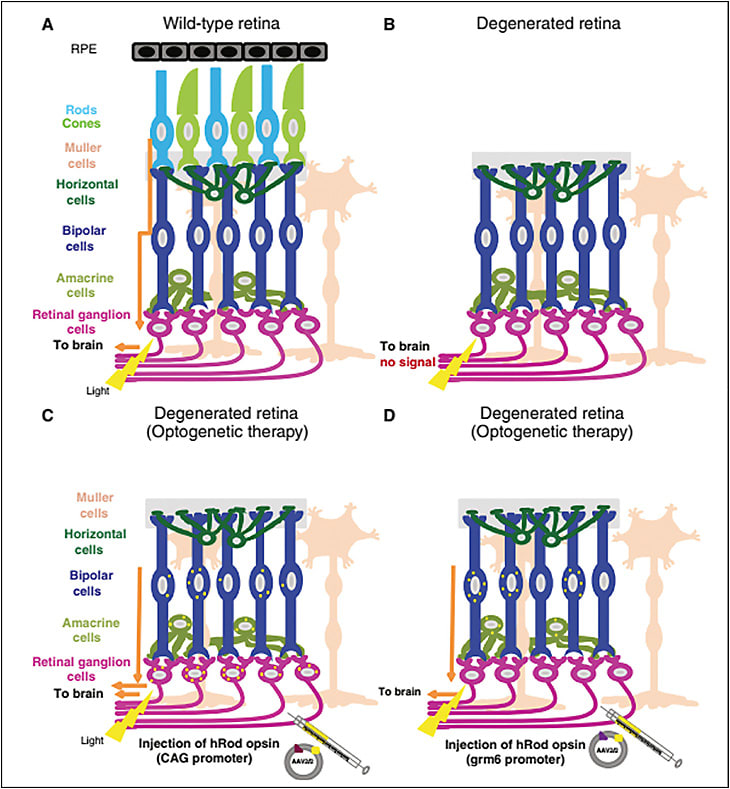
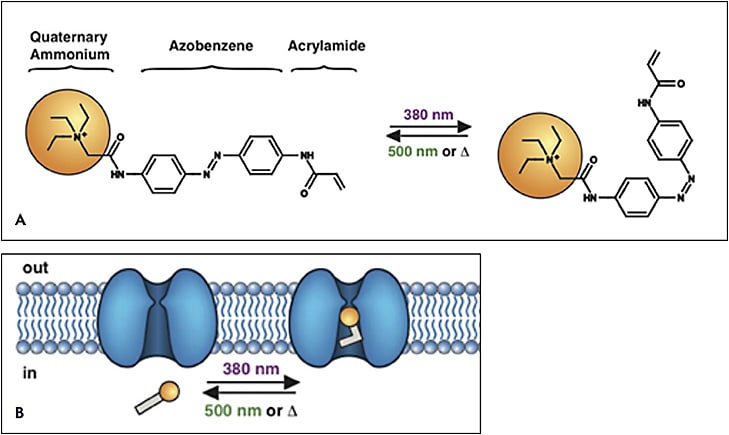
Although the channelopsin approach is very promising, it has 2 significant shortcomings. First, because each opsin molecule controls only its own, single ion channel, there is limited signal amplification. Thus, even current-generation molecules are relatively insensitive and likely will not restore scotopic or mesopic level vision. Second, these proteins are derived from prokaryotes or lower eukaryotes; the possibility of an immune response to the virally expressed, foreign genes is a real concern. To circumvent these problems, in 2015, 2 groups — the laboratories of John G. Flannery, PhD, at the University of California Berkeley7 and Robert Lucas, PhD, at the University of Manchester8 — packaged mammalian rhodopsin into AAV virus and infected the inner retinas of animals with hereditary outer retinal degeneration. Both groups reported identical (and remarkable) results — the viruses restored light sensitivity and visual behaviors to mice with outer retinal degeneration. Many vision scientists would have predicted these experiments would not work, because rhodopsin couples preferentially with the G-protein transducin, which is not expressed at appreciable levels outside the photoreceptors. However, opsins are promiscuous proteins, and it appears the ectopically expressed rhodopsin was able to couple with another G-protein expressed in the inner retina. More recently, the laboratories of Dr. Flannery and Ehud Isacoff, PhD, at Berkeley have expressed mid-wavelength cone in ganglion cells of blind mice and achieved even more impressive vision restoration, with improved kinetics and high sensitivity.9 Along with progress in “evolving” AAV vectors for cell-type specificity,10 this approach shows high promise for permitting intravitreal, single-treatment gene therapy for reversal of blindness. This technology has been licensed by Vedere pharmaceuticals, which is in planning stages for a phase 1/2 clinical trial using this technology.
VISION RESTORATION THROUGH SMALL-MOLECULE PHOTOSWITCHES
Small molecule photoswitches offer an alternative method for vision restoration.11,12 Azobenzene is a light-isomerizable chemical moiety (originally synthesized as a food dye). When covalently coupled to other molecules, it creates a light-dependent conformation change that can change the chemical activity of the molecule. Azobenzenes have been used to confer light-dependence on a wide variety of molecules. In 2005, the laboratories of Richard Kramer, PhD, at Berkeley and Dirk Trauner, PhD, now at New York University began to develop photoswitch agents for vision restoration using modified voltage-gated potassium-channel blockers.13 Closure of voltage-gated potassium channels leads to depolarization of neurons, causing them to fire. Thus, these agents confer light-dependent firing directly on the ganglion cells. The original molecule of this series, called AAQ (for acrylamide-azobenzene-quarternary ammonium) was shown to confer light-dependent firing on ganglion cells in retinal explants, and conferred pupillary light responses on blind mice.14 However, this compound required ultraviolet light for activation, and it required a long-wavelength light to be deactivated. Second-generation compounds, such as DENAQ, were synthesized, which could be activated by visible light and inactivated spontaneously in the dark;15 BENAQ, the successor to DENAQ, was longer lasting (up to 2 weeks following intravitreal delivery).16 A third-generation compound, DAD, remarkably photoswitches in bipolar cells, preserving some retinal signal processing.17 Photoswitches of all generations have been shown to restore light responses on multielectrode array recording of the retinas. Light-dependent behavioral responses have been documented in blind animals treated with first- and second-generation photoswitches.
These compounds have several unexpected but useful properties. First, they appear not to photoswitch nondegenerated retina. It is thought that degeneration upregulates certain channels on the cell surface of downstream cells from the degenerated photoreceptors, which allow these compounds to enter cells. Thus, these agents may have utility in earlier stages of degeneration by selectively photoactivating areas of the retina that are undergoing degeneration.15 Second, degenerated retinas produce 5-Hz and 10-Hz “hum” signals in retinal ganglion cell firing.18 This firing activity can reduce the signal-to-noise ratio, and it has been shown to decrease the effectiveness of inner retinal gene therapy.19 Remarkably, several of the photoswitch compounds are able to suppress this rhythmic firing activity, thus offering the possibility they may provide improved sensitivity.20
Because these compounds vary in solubility and potential for staining tissue, challenges remain in formulating them for optimal ocular use. Also, full toxicology data have not been published yet for these compounds. Nonetheless, this technology has been licensed by Photoswitch Biosciences, which is performing studies to advance these techniques.
WHICH TREATMENT FOR BLINDNESS?
Although both opsin gene therapeutic and small-molecule photoswitch approaches may achieve the same goal — conferring light sensitivity upon inner retinal cells following blindness from photoreceptor loss — they have different potential strengths and weaknesses. The gene therapeutic approach would have advantages of a single treatment leading to permanent restoration of vision. Toxicity is unlikely when using human opsin genes. However, the duration of treatment effect for viral gene therapy is not well established. Further, treatment with AAV likely does not offer an “upgrade” path. Treatment would also likely be limited to end-stage disease because the treatment will co-opt remaining functional vision. In contrast, the small-molecule photoswitch approach will likely require frequent repeated injections or a novel sustained-release delivery mechanism. As with any small-molecule therapy, questions of toxicity will need to be resolved. However, the user will be able to upgrade to new compounds as they become available.
It is important to note that the vision restored by these means will not be native, much the way hearing restored by cochlear implant is not identical to predeafness hearing. First, the visual code of the retina is co-opted in both techniques. For example, “off” pathways in the retina that normally respond to a light-dark transition are converted to “on” signals in both approaches. Second, both approaches are monochromatic; it is unclear what sort of color sensation the superposition of a single spectral sensitivity on a trichromatic retinal ganglion cell population will produce. Third, the mapping of retinal ganglion cells to the physical world in humans is not coincident with photoreceptor mapping. For instance, in the fovea, the lack of ganglion cells in the center of the normal visual field will create a central scotoma with the center of the natural visual field displaced to a ring of 1-2 degrees. However, it is most likely that restored vision, while non-native, will provide sufficient information for regaining many activities of daily living.
CONCLUSION
Opsin gene therapy and small-molecule photoswitch pharmacology allow for conferral of photosensitivity to retinal ganglion cells and have the potential to restore vision in blindness caused by outer retinal degeneration. Both approaches have relative strengths and weaknesses, and while neither will restore native vision, both have the potential to restore useful acuity to blind patients following an in-office procedure. Barring unforeseen issues with toxicity, both approaches should be entering the clinic in the coming decade. RP
REFERENCES
- da Cruz L, Coley BF, Dorn J, Merlini F, et al. The Argus II epiretinal prosthesis system allows letter and word reading and long-term function in patients with profound vision loss. Br J Ophthalmol. 2013;97(5):632-636.
- Goetz G, Smith R, Lei X, et al. Contrast sensitivity with a subretinal prosthesis and implications for efficient delivery of visual information. Invest Ophthalmol Vis Sci. 2015;56(12):7186-7194.
- Rappleye M, Berndt A. Structural basis for ion selectivity and engineering in channelrhodopsins. Curr Opin Struct Biol. 2019;57:176-184.
- Bi A, Cui J, Ma YP, et al. Ectopic expression of a microbial-type rhodopsin restores visual responses in mice with photoreceptor degeneration. Neuron. 2006;50(1):23-33.
- Busskamp V, Duebel J, Balya D, et al. Genetic reactivation of cone photoreceptors restores visual responses in retinitis pigmentosa. Science. 2010;329(5990):413-417.
- Ganjawala TH, Lu Q, Fenner MD, Abrams GW, Pan ZH. Improved CoChR variants restore visual acuity and contrast sensitivity in a mouse model of blindness under ambient light conditions. Mol Ther. 2019;27(6):1195-1205.
- Gaub BM, Berry MH, Holt AE, Isacoff EY, Flannery JG. Optogenetic vision restoration using rhodopsin for enhanced sensitivity. Mol Ther. 2015;23(10):1562-1571.
- Cehajic-Kapetanovic J, Eleftheriou C, Allen AE, et al. Restoration of vision with ectopic expression of human rod opsin. Curr Biol. 2015;25(16):2111-2122.
- Berry MH, Holt A, Salari A, et al. Restoration of high-sensitivity and adapting vision with a cone opsin. Nat Commun. 2019;10(1):1221.
- Dalkara D, Byrne LC, Klimczak RR, et al. In vivo-directed evolution of a new adeno-associated virus for therapeutic outer retinal gene delivery from the vitreous. Sci Transl Med. 2013;5(189):189ra76.
- Tochitsky I, Helft Z, Meseguer V, et al. How azobenzene photoswitches restore visual responses to the blind retina. Neuron. 2016;92(1):100-113.
- Van Gelder RN. Photochemical approaches to vision restoration. Vision Res. 2015;111(Pt B):134-141.
- Kramer RH, Chambers JJ, Trauner D. Photochemical tools for remote control of ion channels in excitable cells. Nat Chem Biol. 2005;1(7):360-365.
- Polosukhina A, Litt J, Tochitsky I, et al. Photochemical restoration of visual responses in blind mice. Neuron. 2012;75(2):271-282.
- Tochitsky I, Polosukhina A, Degtyar VE, et al. Restoring visual function to blind mice with a photoswitch that exploits electrophysiological remodeling of retinal ganglion cells. Neuron. 2014;81(4):800-813.
- Tochitsky I, Trautman J, Gallerani N, Malis JG, Kramer RH. Restoring visual function to the blind retina with a potent, safe and long-lasting photoswitch. Sci Rep. 2017;7:45487.
- Laprell L, Tochitsky I, Kaur K, et al. Photopharmacological control of bipolar cells restores visual function in blind mice. J Clin Invest. 2017;127(7):2598-2611.
- Borowska J, Trenholm S, Awatramani GB. An intrinsic neural oscillator in the degenerating mouse retina. J Neurosci. 2011;31(13):5000-5012.
- Barrett JM, Degenaar P, Sernagor E. Blockade of pathological retinal ganglion cell hyperactivity improves optogenetically evoked light responses in rd1 mice. Front Cell Neurosci. 2015;9:330.
- Hull K, Benster T, Manookin MB, Trauner D, Van Gelder RN, Laprell L. Photopharmacologic vision restoration reduces pathological rhythmic field potentials in blind mouse retina. Sci Rep. 2019;9(1):13561.
- Van Gelder RN, Kaur K. Vision science: can rhodopsin cure blindness? Curr Biol. 2015;25(16):R713-R715.
- Mourot A, Tochitsky I, Kramer RH. Light at the end of the channel: optical manipulation of intrinsic neuronal excitability with chemical photoswitches. Front Mol Neurosci. 2013;6:5.