Diabetic retinopathy (DR) is the most common cause of blindness among working-age adults in developed countries.1 Traditionally described retinal vascular changes with this disease process include microaneurysms, intraretinal microvascular abnormalities (IRMAs), capillary nonperfusion, and diabetic macular edema (DME).2 In proliferative DR (PDR), neovascularization can lead to vitreous hemorrhage, tractional retinal detachment, and severe vision loss.2 Color fundus photographs have been the gold standard for assessing DR severity, with the use of the Early Treatment Diabetic Retinopathy Study (ETDRS) grading system.3,4 Fluorescein angiography (FA) has been an important imaging technique for diagnosis and monitoring of DR since the 1960s.
In recent years, optical coherence tomography angiography (OCTA) has become increasingly used to evaluate DR. Optical coherence tomography angiography is relatively fast, noninvasive, safe, and easily repeatable; it can use en-face angiograms of specific anatomic layers while providing cross-sectional B-scans that are useful for detecting DME and other signs of DR.5-9 It can be used to demonstrate enlargement of the foveal avascular zone, capillary perfusion abnormalities and ischemia, choriocapillaris flow impairment, and preretinal neovascularization (Figure 1).10-14 While there has been no standardized classification system for DR based on OCTA findings, the use of this technology has allowed for description of new entities associated with diabetic retinopathy.
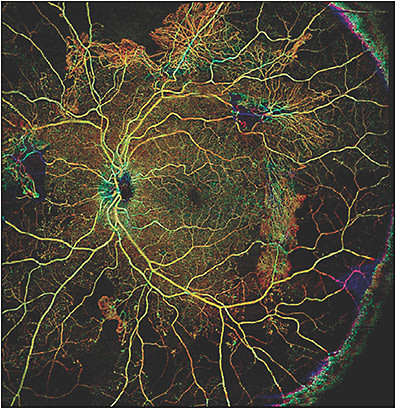
In comparison to spectral-domain OCTA (SD-OCTA), swept-source OCTA (SS-OCTA) has been increasingly used and has numerous advantages (Table 1). One of the limitations of OCTA is the size of the scanning area, which is of concern because vascular disease in DR extends beyond the posterior pole. The use of widefield SS-OCTA imaging (12 mm x 12 mm and 15 mm x 9 mm with montage) can localize neovascularization in a similar fashion to that of ultrawidefield FA.15 The ability to capture neovascularization with a single 12 mm x 12 mm SS-OCTA scan or by using the wider 15 mm x 9 mm montage view emphasizes the advantage it has over SD-OCTA, which has a smaller field of view and requires targeted scans of areas with neovascularization.12
Spectral-Domain OCTA | Swept-Source OCTA |
---|---|
Light source: broadband near-infrared superluminescent diode laser (wavelength: 840 nm) | Light source: tunable swept laser (wavelength: 1060 nm) |
Slower scanning speed | Faster scanning speed, allowing for denser scan patterns and larger scan areas |
Less detail through the retinal pigment epithelium | Better light penetration through the retinal pigment epithelium (advantage in use for imaging choroidal neovascularization) |
* Adapted from Miller et al, Comparison between spectral-domain and swept-source optical coherence tomography angiographic imaging of choroidal neovascularization. Invest Ophthalmol Vis Sci. 2017;58(3):1499-1505. OCTA, optical coherence tomography angiography |
Swept-source OCTA has the ability to clearly demonstrate neovascularization that can only be seen in part on fundus exam. It has been reported that SS-OCTA detected 50% of intraretinal microvascular abnormalities that were missed on color fundus photographs.16 For PDR, SS-OCTA has the ability to identify subtle neovascularization that may not be seen on clinical exam (Figure 2). Futhermore, SS-OCTA is able to detect choroidal neovascularization secondary to retinal laser that cannot be readily identified on clinical exam (Figure 3). Swept-source OCTA has significant advantages in the detection of choroidal neovascular membranes in various other retinal diseases.
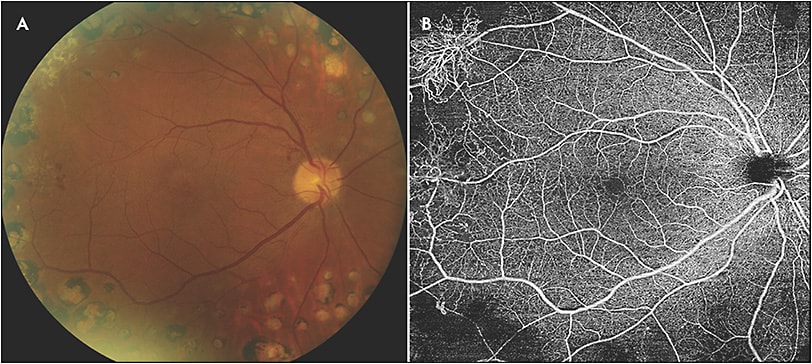
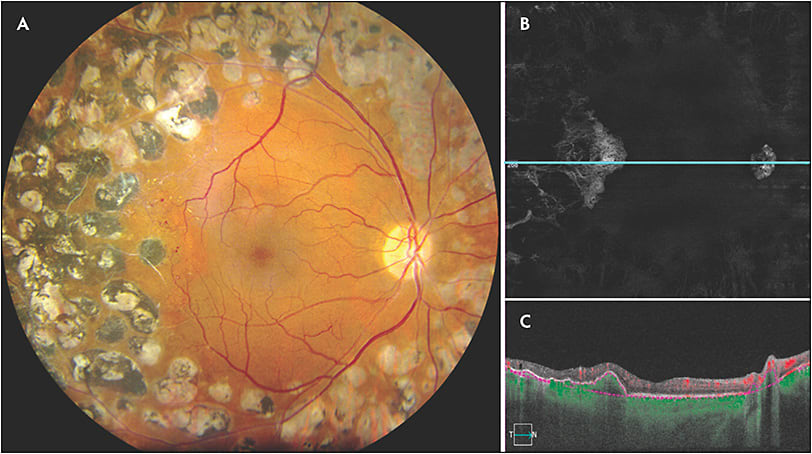
Although SS-OCTA can be used to confirm the neovascularization seen on ultrawidefield FA, SS-OCTA also has the advantage of providing en-face segmentation of different layers, because vascular changes in DR may not be confined to the superficial vascular network alone.17-19 Parafoveal capillary nonperfusion seems to occur initially at the level of the deep capillary plexus, and microaneurysms can be seen in all 3 capillary plexuses.18,19 Neovascularization as seen on FA can be correlated to similar findings on SS-OCTA, with the advantage of the latter being noninvasive (Figure 4). Swept-source OCTA can identify subtle alterations in flow within diabetic lesions, in addition to being able to identify microvascular changes unable to be detected by FA.15 Another advantage of SS-OCTA over FA is that it can be used to monitor eyes for progression or regression of neovascularization in response to treatment, because fluorescein leakage may obscure detailed visualization of neovascularization (Figure 5).15
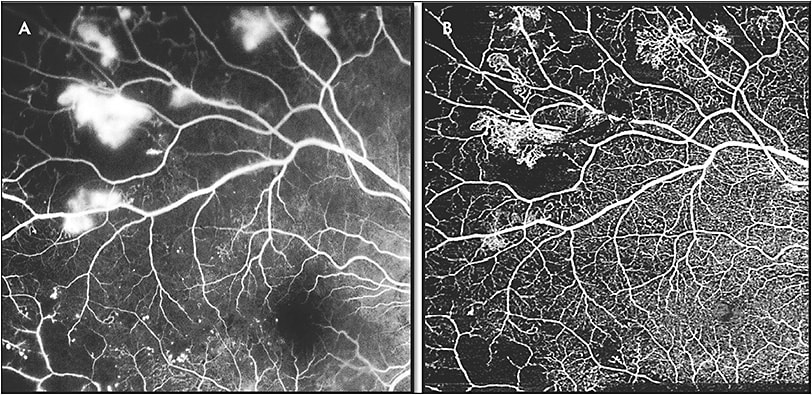
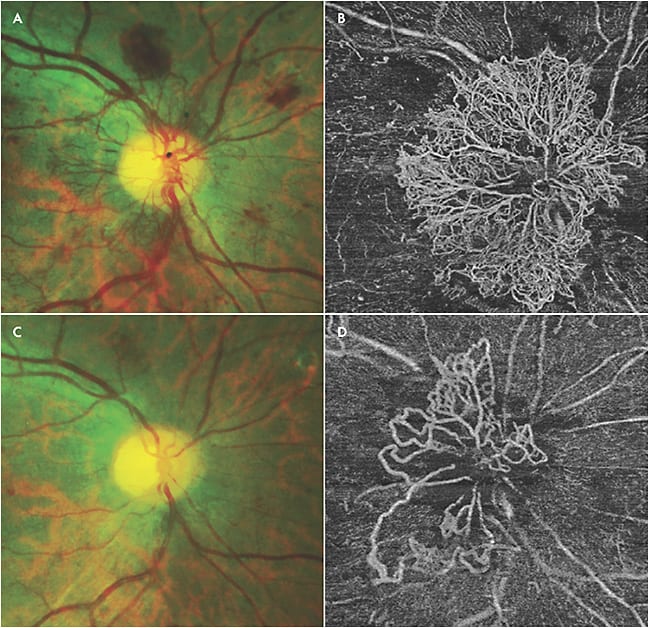
Regarding new terminology, there are at least 3 new OCTA descriptions of retinal vascular changes that can be identified in DR (Table 2). Disorganization of retinal inner layers (DRIL) occurs in eyes with ischemic changes in diabetic retinopathy and is characterized by loss of boundaries between the ganglion cell, inner plexiform, and outer plexiform layers.20 It is associated with morphologic changes in the inner retina, corresponding to more severe DR and poorer visual acuity.21 While exclusively an OCT diagnosis, DRIL can be better identified on SS-OCTA because of the advantage of segmenting different layers (Figure 6). In particular, areas of ischemia on OCTA scans correspond to areas of DRIL as seen on OCT B scans.22 Diabetic macular edema/DRIL can be viewed via B scans, allowing for one imaging modality to evaluate diabetic retinopathy.15
Disorganization of Inner Retinal Layers | This optical coherence tomography (OCT)/OCT angiography (OCTA) feature identifies areas in which the boundaries between ganglion cells, inner plexiform layer, inner nuclear layer, and outer plexiform layer cannot be distinguished on the B scan. These areas are associated with capillary flow deficit or capillary nonperfusion.20 |
Suspended Scattering Particles in Motion | These regions show extravascular motion on OCTA corresponding to intraretinal accumulations of fluid and increased OCT signal density associated with hyper-reflective material found bordering the fluid.23 |
Capillary Perfusion Density | OCTA can be used to create color-coded perfusion maps and capillary perfusion density values in diabetic retinopathy. This mapping may be useful in defining early stages of diabetic retinopathy.10 |
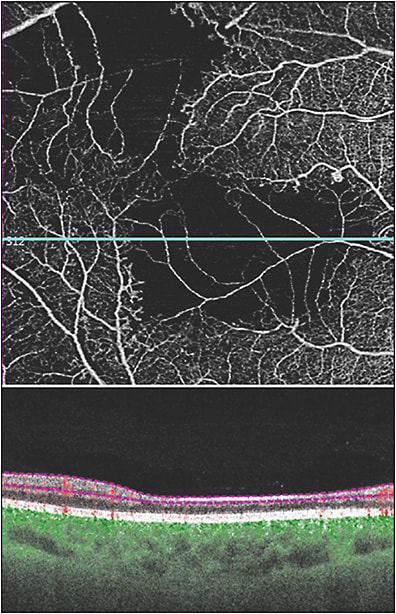
Another finding specific to that of OCTA is suspended scattering particles in motion, which has been characterized by an extravascular OCTA signal corresponding to hyper-reflective fluid in exudative maculopathies, including diabetic retinopathy (Figure 7).23 Suspended scattering particles in motion have an anatomic localization near vascular-avascular junctions and are most often identified in Henle fiber layer. It is largely associated with hyper-reflective material as seen on OCT, which likely represents hard exudates or subclinical extravasation of lipoproteins. The signal itself is probably associated with lipid aggregates suspended in motion as a result of breakdown of the blood–retinal barrier. While its clinical utility is still under investigation, ultimately its resolution can be associated with visualization of hard exudates within the neurosensory retina.
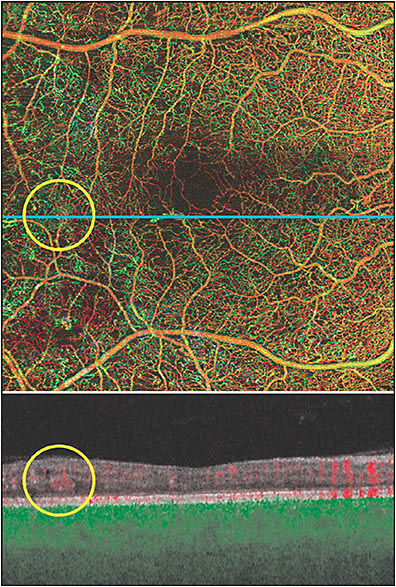
In diabetic patients without clinically apparent retinopathy, OCTA can be used to detect abnormalities in and around the foveal avascular zone, showing subtle abnormalities in capillary perfusion density compared to nondiabetic eyes.13 When comparing OCT metrics in diabetic patients without DR, perifoveal capillary loss in the superficial capillary plexus and inner retinal layer thickness can be identified.24 Measurement of capillary perfusion density on OCTA has been found to be lower in eyes with moderate or severe nonproliferative DR or PDR compared to eyes with no or mild nonproliferative DR.25 Capillary perfusion density (CPD) maps employ color-coded topographic maps with quantitative data to objectively grade and linearly assess the microvascular status of diabetic disease in the 3 layers from the en face OCTA (superficial retinal capillaries, deep retinal capillaries, and choriocapillaris). CPD values have been found to be significantly lower in those with early changes of diabetic retinopathy compared to age-matched controls.10 In nonproliferative DR and PDR, OCTA enables assessment and quantification of perfusion, as well as the ability to identify in which layer the nonperfusion is located within the retinal and choroidal vasculature (Figure 8). However, measurements of vessel density as well as perfusion density are not standardized across different OCTA platforms.26
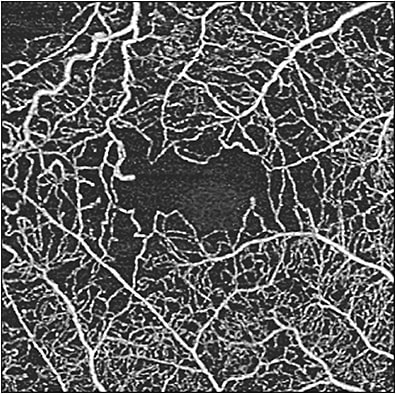
The use of OCTA, particularly SS-OCTA, has widespread implications in evaluating diabetic retinopathy. Imaging research suggests that widefield SS-OCTA may be the only imaging modality needed for diagnosis and longitudinal management of PDR.27 While the clinical significance of some of the new findings using this technology are yet to be determined, its ability to further characterize specific diseases processes in diabetic retinopathy may allow earlier detection, better prognostication, and improved treatment outcomes. RP
REFERENCES
- Zhang X, Saaddine JB, Chou CF, et al. Prevalence of diabetic retinopathy in the United States, 2005-2008. JAMA. 2010;304(6):649-656.
- Schachat AP, Wilkinson CP, Hinton DR, et al, eds. Ryan’s Retina. 6th ed. Edinburgh: Elsevier; 2017.
- Early Treatment Diabetic Retinopathy Study Research Group. Grading diabetic retinopathy from stereoscopic color fundus photographs--an extension of the modified Airlie House classification. ETDRS report number 10. Ophthalmology. 1991;98(5 Suppl):786-806.
- Diabetic retinopathy study. Report Number 6. Design, methods, and baseline results. Report Number 7. A modification of the Airlie House classification of diabetic retinopathy. Prepared by the Diabetic Retinopathy. Invest Ophthalmol Vis Sci. 1981;21(1 Pt 2):1-226.
- Choi W, Mohler KJ, Potsaid B, et al. Choriocapillaris and choroidal microvasculature imaging with ultrahigh speed OCT angiography. PLoS One. 2013;8(12):e81499.
- Huang Y, Zhang Q, Thorell MR, et al. Swept-source OCT angiography of the retinal vasculature using intensity differentiation-based optical microangiography algorithms. Ophthalmic Surg Lasers Imaging Retina. 2014;45(5):382-389.
- Schaal KB, Legarreta AD, Gregori G, et al. Widefield en face optical coherence tomography imaging of subretinal drusenoid deposits. Ophthalmic Surg Lasers Imaging Retina. 2015;46(5):550-559.
- Sun JK, Lin MM, Lammer J, et al. Disorganization of the retinal inner layers as a predictor of visual acuity in eyes with center-involved diabetic macular edema. JAMA Ophthalmol. 2014;132(11):1309-1316.
- Wang JC, Lains I, Providencia J, et al. Diabetic choroidopathy: choroidal vascular density and volume in diabetic retinopathy with swept-source optical coherence tomography. Am J Ophthalmol. 2017;184:75-83.
- Agemy SA, Scripsema NK, Shah CM, et al. Retinal vascular perfusion density mapping using optical coherence tomography angiography in normals and diabetic retinopathy patients. Retina. 2015;35(11):2353-2363.
- Couturier A, Mane V, Bonnin S, et al. Capillary plexus anomalies in diabetic retinopathy on optical coherence tomography angiography. Retina. 2015;35(11):2384-2391.
- de Carlo TE, Bonini Filho MA, Baumal CR, et al. Evaluation of preretinal neovascularization in proliferative diabetic retinopathy using optical coherence tomography angiography. Ophthalmic Surg Lasers Imaging Retina. 2016;47(2):115-119.
- de Carlo TE, Chin AT, Bonini Filho MA, et al. Detection of microvascular changes in eyes of patients with diabetes but not clinical diabetic retinopathy using optical coherence tomography angiography. Retina. 2015;35(11):2364-2370.
- Takase N, Nozaki M, Kato A, Ozeki H, Yoshida M, Ogura Y. Enlargement of foveal avascular zone in diabetic eyes evaluated by en face optical coherence tomography angiography. Retina. 2015;35(11):2377-2383.
- Russell JF, Shi Y, Hinkle JW, et al. Longitudinal wide-field swept-source oct angiography of neovascularization in proliferative diabetic retinopathy after panretinal photocoagulation. Ophthalmol Retina. 2019;3(4):350-361.
- Schaal KB, Munk MR, Wyssmueller I, Berger LE, Zinkernagel MS, Wolf S. Vascular abnormalities in diabetic retinopathy assessed with swept-source optical coherence tomography angiography widefield imaging. Retina. 2019;39(1):79-87.
- Zahid S, Dolz-Marco R, Freund KB, et al. Fractal dimensional analysis of optical coherence tomography angiography in eyes with diabetic retinopathy. Invest Ophthalmol Vis Sci. 2016;57(11):4940-4947.
- Simonett JM, Scarinci F, Picconi F, et al. Early microvascular retinal changes in optical coherence tomography angiography in patients with type 1 diabetes mellitus. Acta Ophthalmol. 2017;95(8):e751-e755.
- Park JJ, Soetikno BT, Fawzi AA. Characterization of the middle capillary plexus using optical coherence tomography angiography in healthy and diabetic eyes. Retina. 2016;36(11):2039-2050.
- Sun JK, Radwan SH, Soliman AZ, et al. Neural retinal disorganization as a robust marker of visual acuity in current and resolved diabetic macular edema. Diabetes. 2015;64(7):2560-2570.
- Das R, Spence G, Hogg RE, Stevenson M, Chakravarthy U. Disorganization of inner retina and outer retinal morphology in diabetic macular edema. JAMA Ophthalmol. 2018;136(2):202-208.
- Moein HR, Novais EA, Rebhun CB, et al. Optical coherence tomography angiography to detect macular capillary ischemia in patients with inner retinal changes after resolved diabetic macular edema. Retina. 2018;38(12):2277-2284.
- Kashani AH, Green KM, Kwon J, et al. Suspended scattering particles in motion: a novel feature of oct angiography in exudative maculopathies. Ophthalmol Retina. 2018;2(7):694-702.
- Vujosevic S, Muraca A, Alkabes M, et al. Early microvascular and neural changes in patients with type 1 and type 2 diabetes mellitus without clinical signs of diabetic retinopathy. Retina. 2019;39(3):435-445.
- Lin AD, Lee AY, Zhang Q, et al. Association between OCT-based microangiography perfusion indices and diabetic retinopathy severity. Br J Ophthalmol. 2017;101(7):960-964.
- Munk MR, Giannakaki-Zimmermann H, Berger L, et al. OCT-angiography: a qualitative and quantitative comparison of 4 OCT-A devices. PLoS One. 2017;12(5):e0177059.
- Russell JF, Flynn HW, Jr., Sridhar J, et al. Distribution of diabetic neovascularization on ultra-widefield fluorescein angiography and on simulated widefield OCT angiography. Am J Ophthalmol. 2019. [Epub ahead of print]