Fifty years ago, the operating microscope was introduced into the ophthalmic surgical suite and became the standard for intraoperative surgical viewing. Current analog microscopes have excellent optics, lighting, and depth of field that permit vitreoretinal surgeons to repair an extensive array of surgical pathologies. However, there are limitations to our current analog optical visualization that include reliance on microscope oculars, excess light exposure for the patient, and the relative inability to add heads-up information to the surgeon’s view.
Over the past 5 to 10 years, digital technology has evolved to permit high-resolution intraoperative surgical visualization with short-image latency. This has resulted in quality commensurate with current analog microscopes and provides distinct advantages over our current standard of care. This article compares current analog operative microscopy with digital 3D-HD machine vision for primary viewing for vitreoretinal surgery and discusses developments on the horizon that promise to significantly enhance the digital surgical viewing experience.
Alan J. Franklin, MD, PhD, is from Diagnostic and Medical Clinic in Mobile, AL. Ramesh Sarangapani, PhD, and Lu Yin, PhD, are with Alcon Laboratories in Fort Worth, TX. Burton Tripathi, PhD, is from TrueVisionSystems, Inc. in Santa Barbara, CA. Christopher Riemann, MD, is from the Cincinnati Eye Institute and University of Cincinnati in Cincinnati, OH. Dr. Riemann reports consultancy to Haag-Streit USA, Haag-Streit AG, Haag-Streit Surgical, TrueVision, and Alcon. Dr. Tripathi reports patents with TrueVision Systems, Inc. The remaining authors report no related disclosures. The authors wish to acknowledge the assistance of Mark Maire and Joshua Anderson in the review of this manuscript, and Christopher Atkins for surgical assistance. Dr. Franklin can be reached at alfranklin84@gmail.com. Dr. Riemann can be reached at criemann@cincinnatieye.com.
IMAGE QUALITY AND STEREOACUITY
Critical determinants of digital image quality include resolution, camera dynamic range, and frame rate. A latency of less than 100 ms is necessary for the video feed to be useful for heads-up surgical viewing. Advances in digital technology — chipsets and firmware for image capture, processing, and display of real-time high-quality digital video — occurred approximately 5 to 7 years ago and have made heads-up surgical visualization a reality. Stereoacuity is directly related to the stereoscopic depth resolution at the scope object plane, which is the finest depth (axial resolution) that a physician can resolve from the patient eye. Depth resolution is also directly related to the magnification of the scope.
For example, 480i video (our parents’ TV) reproduces approximately 20/200 equivalent visual acuity when displayed with viewing geometry analogous to the surgical microscope. However, 1,080-pixel high-definition digital video yields an effective pixilar density comparable to 20/40 visual acuity. 4K monitors can display the full-resolution 1,080-pixel left and right images simultaneously and even better with the NGENUITY 4K UHD monitor. This is a key point. High-grade stereopsis is predicated on excellent high-grade visual acuity in both eyes. Vision of 20/200 (our parents’ 480i TV) yields stereoacuity of 800 seconds of arc — one circle on the Randot stereoacuity test. This is minimal gross stereopsis, which is incompatible with primary surgical viewing. Increasing the magnification on the microscope slightly and digital imaging with 1,080-pixel resolution produces image quality at least equivalent to 20/20 visual acuity. With higher magnification, stereopsis is further enhanced with digital 3D-HD 4K. This yields stereoacuity of 40 seconds of arc or 9 out of 9 circles on the Randot acuity test. So, digital outperforms analog in terms of stereoacuity.
Dynamic range represents the range of light levels that can be detected and is typically measured by f-stops, exposure values, decibels, or zones. Typical analog microscope systems coupled with human vision have a 10 to 14 f-stop dynamic range, whereas current digital technology is capable of displaying 8 to 11 f-stops of dynamic range. High-dynamic-range imaging algorithms have further increased the effective dynamic range so that digital dynamic range exceeds what our eyes see when looking through the oculars of the microscope.
Latency is defined as the delay from the occurrence of an event to when the event is visualized on a display device. With analog microscopy, the “latency” of the system is defined as zero. The event appears in the oculars at the same time as it occurs (the speed of light results in a negligible delay of about 2 to 3 nanoseconds). The NGENUITY Digitally Assisted Vitreoretinal Surgery System (Alcon Laboratories) has a latency in the 80 ms range. However, visual evoked potential data and reaction time experiments suggest that the inherent internal delay of the human visual system and afferent upper extremity motor neuron complex — or “perceptual latency” from seeing to reacting to a visual stimulus — is in the 200 to 350 ms range.1,2 Therefore, to calculate total latency, the digital latency of NGENUITY is added to the pre-existing and much greater “perceptual latency of human neurophysiology.” The “total perceptual latency” of “heads-up” digitally assisted viewing systems (80 + 200 = 280 ms) is therefore only mildly greater than the “perceptual latency” of analog viewing through the microscope (200 ms). Vitreoretinal surgery is a slow-moving surgery, and this increased delay is not clinically relevant.
Other important imaging parameters include contrast, color saturation, and tone reproduction or luminance. Contrast is relatively static in most analog surgical microscopes, although some modifications of light source, such as using either xenon or mercury vapor bulbs, can change and improve contrast for some surgical pathologies. However, both contrast and color saturation can be enhanced intraoperatively on the imaging side of things with digital visualization in real time. Currently available technology includes a digital redshift setting that is beneficial in patients with opacification from vitreous hemorrhage (Figure 1), and it enhances visualization of preretinal membranes in proliferative diabetic and vitreoretinopathy cases. We believe that real-time digital image processing also will support more refinements to enhance visualization of the intraocular anatomy.
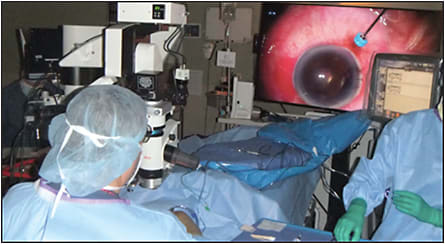
Tone reproduction (or luminance) is excellent with NGENUITY, which permits much lower lighting levels to be used from the light source. Typically, 20% to 30% of the light source intensity is used compared with analog viewing through the microscope. For us, light pipe intensity is reduced from 35% to the 5% to 20% range when operating with the Alcon Constellation and from 100% to 30% to 50% when using the Dutch Ophthalmic EVA machine. Moreover, reduced light exposure may be important in decreasing phototoxicity during more complicated cases, resident and fellow training, or macular peeling.
Another benefit of the high light sensitivity of the NGENUITY system is a gain in depth of field by using a smaller camera aperture while keeping the eye illumination at the same (or reduced) levels. Depth of field is the axial range in the patient eye where structure appears to be in focus for the physician (ie, in good sharpness). Depth of field is theoretically inversely related to the diameter of the camera aperture. For example, the NGENUITY system operating with the camera aperture reduced to half provides a depth of field slightly exceeding that of analog scope for a physician having 2 D of accommodation power. Physicians can gain additional depth of field by changing eye accommodation when using an analog scope but not with the NGENUITY system. The depth of field with NGENUITY exceeds that of the standard operating analog microscope by twofold to threefold if the NGENUITY system camera aperture is reduced to 30% (Table 1).3,4 This difference is even greater for surgeons without a high degree of eye accommodation.
COMPARISON CONDITION | SCOPE | NGENUITY | CONDITIONS/COMMENTS | ||
---|---|---|---|---|---|
Condition | |||||
Fraction of opening of the camera aperture (r) | N/A | 30% | 50% | In reference to camera aperture diameter | |
Viewing distance to NGENUITY display (lviewing) | N/A | 1.5 m | Recommended setting | ||
Total magnification (βtotal) | 10 | Magnification of the patient eye for the physician to see | |||
Comparison | |||||
Depth of field (ΔZ dof, mm) | Term 1 | 0.14 | 2.5 | 0.9 | Smaller camera aperture opening means smaller NAscope |
Term 2 | 0.75 | 3.2 | 1.9 | ||
Term 3 | 1.2 | N/A | |||
Total | 2.1 | 5.7 | 2.8 |
The theoretical calculation shown in Table 1 was based on the Zeiss OPMI LUMERA 700, with the objective lens having focal length of 200 mm and 10× oculars but without a retina viewing system, and the NGENUITY system 1.0.
The depth of field for viewing through oculars of the scope/NGENUITY is calculated as the summation of 3 terms in the following equation (equation 6.13 in Kaschke et al4):
Here λ is the wavelength of the light (550 nm); NAscope is the numerical aperture of the scope, which decreases for a smaller camera aperture; βtotal is the total magnification of Zeiss scope/NGENUITY system; lnv is the near viewing distance of 250 mm; and Paccom is the accommodation power of the physician’s eye (2 D).
Depth of field was estimated for the situation of viewing the anterior part of the patient’s eye without a retina viewing system. The estimates of depth of field for viewing the posterior part of patient eye will change with the retina viewing system in the light path. However, the qualitative comparison between scope and NGENUITY system shown in Table 1 remains valid.
ERGONOMICS
Surgeon ergonomics are often overlooked to the great detriment of ophthalmologists and their patients. Between 50% and 80% of ophthalmologists develop significant cervical-spine pathology during their career, and the proportion is likely worse for vitreoretinal surgeons. With analog visualization, our posture with the microscope is typically far from ideal (Figure 2A). While we can all do a better job of being cognizant of and maintaining better ergonomics during microscope-based surgery, a high risk of cervical-spine pathology remains. Heads-up surgery affords us a chance to completely change our ergonomics by eliminating the microscope oculars. Back and cervical-spine position becomes physiologic, and the surgeon can enjoy lumbar support throughout surgery (Figure 2B). Careful setup of the heads-up visualization system permits excellent monitor visualization without head tilting or turning.5-8
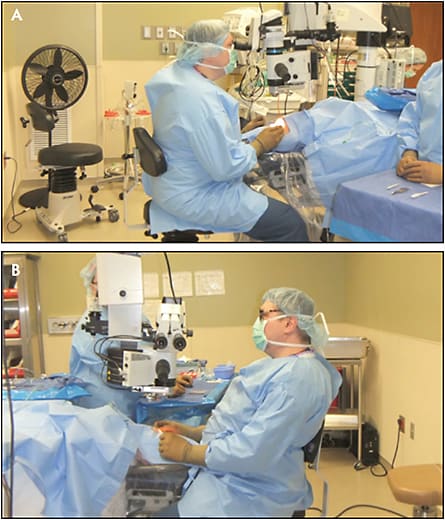
OBSERVATION AND TEACHING
With analog microscopes, the only observers with a high-grade stereo view of the surgical field are the operating surgeon and — sometimes — a single observer at a stereo side scope. Any remaining observers view a 2D video feed that does not render the fine nuances of the detailed microsurgical maneuvers and dissections being performed. Digital viewing affords the entire surgical team and observers the ability to view the exact same 3D high-definition view of the surgical field as the operating surgeon both in the OR suite and in remote locations so long as enough bandwidth is available. This is unprecedented. Large numbers of trainees can have a substantive observational experience far better than looking at a 2D monitor.5-8
Digital observation allows the attending surgeon to see the exact same high-definition 3D view as the operating trainee. This can be useful because optical paths from the eye to the side scope are different from those from the eye to the main scope and do not always show the same view, especially when operating at the edge of the surgical field. Surgical viewing with digitally assisted heads-up viewing is accomplished without the awkward positioning at the side scope — ergonomics at the side scope are often far worse than at the main oculars — and without the challenges of significant disparity in accommodation between the trainee and attending surgeon, which are eliminated by the 3D monitor (Figure 3). Because the attending surgeon sees exactly what the trainee sees and does, trainees can perform a greater proportion of the surgical case, and switching operating surgeons is seamless, faster, and efficient without the need to adjust microscope ocular pupillary distance or surgeon accommodation settings. Moreover, the trainee is observed from a distance measured in meters as opposed to centimeters, which increases the trainee’s perception of autonomy and reduces trainee anxiety. Reduced attending anxiety from better surgical viewing and a faster surgical switch and less trainee anxiety means trainees function better and get to do more — a recipe for surgical success.5-8
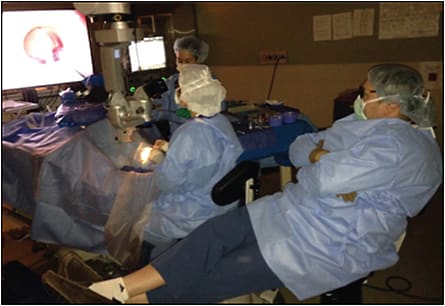
A SURGICAL INFORMATION-HANDLING “COCKPIT”
Image overlay potential is quite limited with analog or microscope surgical visualization, whereas digital technology can perform iris and retinal vascular surgical registry. From an anterior segment perspective, digital overlay technology greatly streamlines the intraoperative workflow necessary for operative astigmatism management. Limbal relaxing incisions and toric intraocular lens implants are precisely placed with refractive results within 0.5 D of the intended target in more than 90% of eyes without awkward and inefficient marking with an ink marking pen.
Translating this functionality to vitreoretinal surgery holds a great deal of promise. Preoperative widefield fluorescein angiography and optical coherence tomography can now be viewed on the same screen as the live surgical field. Soon, we will have these images registered and overlaid on the live surgical field. Vascular registry may permit automated intraoperative pattern laser targeted to ischemic retina in the future. In addition, we will be able to “see” areas of vitreoretinal adhesion and nonperfusion live on our surgical fields, and this information will guide our surgical dissections. Imagine being able to “see” the adhesion pegs of complex diabetic tractional retinal detachments.
The heads-up platform also permits display of the vitrectomy or phaco machine to “overlay” dashboard settings on the live surgical field. This allows the surgeon simultaneous surgical field and machine information, such as true aspiration, intraocular pressure, cut rate, laser power, etc. in real-time on the heads-up NGENUITY display.
The ability to seamlessly display information as either as an overlay or as auxiliary video on a single heads-up monitor offers a large advantage compared with current analog technology. As we gain access to more information and diagnostic aides that can be generated in real-time to assist the vitreoretinal surgeon, such as next-generation endoscopy and intraoperative OCT, the advantage gap between analog and digital technology will significantly widen toward the palette of heads-up digital display. Digitally assisted surgical viewing will allow for functionality as an information management system — a “surgical cockpit.”9
DIGITAL IMAGE ENHANCEMENT AND FUTURE TECHNOLOGIES
The ability to visualize vitreous for removal and to see through vitreous opacities are critical issues that influence the success and efficiency of retinal surgery. Digital viewing can enhance vitreous visualization to assist in more complete and efficient gel removal in individuals with relatively lucent vitreous. Redshift viewing to improve visualization through vitreous hemorrhage permits better, safer, and faster vitreous removal as the underlying retinal anatomy is better visualized through the blood. Membrane delamination is both safer and more efficient since redshift visualization enhances the view for tissue manipulation and does not require complete hemostasis throughout the process of membrane removal (Figure 4).
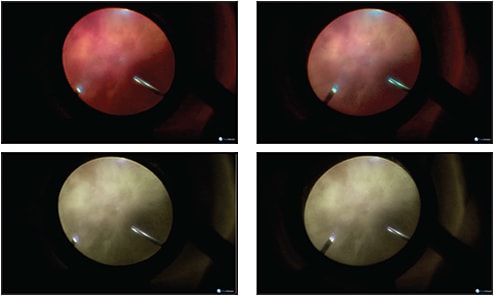
Digital surgical viewing is also amenable to image processing such as edge enhancement, color space transformation, and adjustment of brightness and contrast. These image enhancements are rendered in real time to the surgeon without a significant increase in latency. Algorithms are being explored to improve visualization of inner retinal structures and preretinal tissue — a “virtual dye” view to reduce or eliminate the need for potentially toxic dyes currently in use.
Intraoperative OCT is currently available from 3 manufacturers and can be displayed in very low resolution on an image injection system coupled to analog surgical microscope oculars or in high resolution on a separate digital screen that is a face turn away from the oculars. The low-resolution image is overlaid approximately 10° to 15° off the center of the field, which can interfere with visualization during complex membrane delamination during proliferative diabetic and vitreoretinopathy cases. As intraoperative OCT is further developed, intraoperative OCT images will be overlaid and registered to the live surgical field. Digital viewing should prove quite useful to display output from a surgical endoscope as an auxiliary video input also.
Multispectral retinal imaging utilized with fundus photography has shown some promising information in terms of improved visualization of the retinal vasculature, detection of oxygenation, and the ability to selectively visualize superficial and deep layers in the retina and choroid, so this technology may provide more useful information intraoperatively as well. Further refinements of digital enhancement algorithms will continue to provide more precise and clear retinal images during surgery. Vascular registry will permit real-time pattern laser intraoperatively, which will allow us to deliver endolaser more precisely and efficiently.
Other developing technologies that may be congruent with digital heads-up surgical viewing include digital instrument tip tracking to avoid inadvertent touch downs or lens touch, multifocal electroretinography, and ultrasound biomicroscopy (Table 2). We will be able to see and integrate more of the information we need, when we need it, and will be able to see it overlaid onto our live surgical field.9
Office diagnostic testing data that can be imported into heads-up multidisplay technology | Current and developing intraoperative visualization technologies that can be displayed as either multidisplay or overlay “surgical cockpit” technology |
---|---|
Office testing | Intraoperative visualization |
Intravenous fluorescein angiography | Instrument tip tracking |
Redshift | Safe zone |
Autofluorescence | Inadvertent touch down |
Infrared | Inadvertent lens touch |
OCT | Real-time surgical capture |
Microperimetry | Intraoperative OCT |
Multifocal electroretinography | Microscope integrated |
Ultrasound biomicroscopy | Intraocular B-scan |
B-scan ultrasound | Multispectral imaging |
High-resolution MRI with eye coil | Multifocal electroretinography |
CONCLUSIONS
Digital viewing for vitreoretinal surgery was first performed 9 years ago. This very young technology has already evolved to provide real-time surgical visualization that is at least equivalent to and often superior to the gold-standard surgical analog microscope. Digitally assisted vitreoretinal viewing technology currently offers distinct advantages for surgeon ergonomics, surgical teaching, light exposure and phototoxicity, and digital algorithms to suppress vitreous hemorrhage and enhance fibrotic and fibrovascular proliferation visualization. It also offers the ability to handle and efficiently display multiple preoperatively and intraoperatively obtained images and video streams. Future overlay technologies will permit facile simultaneous viewing of machine settings, registered preoperative and intraoperative OCT, endoscopy, and a whole array of future technologies. We stand at the precipice of a digital revolution for vitreoretinal surgical visualization. RP
REFERENCES
- Yuhas D. Speedy science: how fast can you react? Scientific American. Available at: http://www.scientificamerican.com/article/bring-science-home-reaction-time/ . Accessed April 4, 2017.
- Ng A, Lepinski J, Wigdor D, Sanders S, Dietz P. Designing for low-latency direct-touch input. Proceedings of the 25th annual ACM symposium on user interface software and technology. Cambridge, MA, October 7-10, 2012:453-464.
- James J. Relation of objective and eyepiece. In: Light Microscopic Techniques in Biology and Medicine. The Hague, the Netherlands: M. Nijhoff Medical Division; 1976:75-91.
- Kaschke M, Donnerhacke K, Rill M. Optical visualization, imaging, and structural analysis. In: Optical Devices in Ophthalmology and Optometry Technology: Design Principles and Clinical Applications. Weinheim, Germany: Wiley-VCH; 2014:147-276.
- Ophthalmology/image-guided surgery: ‘heads-up’ 3D-enabled retinal surgery broadcast live. BioOptics World. May 2014. Available at: http://www.bioopticsworld.com/articles/print/volume-7/issue-3/departments/news-notes/ophthalmology-image-guided-surgery-heads-up-3d-enabled-retinal-surgery-broadcast-live.html . Accessed April 4, 2017.
- Blum K. 3D in the OR: a better view from many angles. Ophthalmol Manag. 2013;17(9):53-57.
- Weinstock R, Donnenfeld E. 3D visualization in ophthalmology. Cataract Refractive Surg Today. 2008;May:62-63,65. Available at http://crstoday.com/articles/2008-may/crst0508_16-php/ . Accessed April 4, 2017.
- Dubay L. 3D-enabled Leica Microsystems microscope performs ophthalmic surgery. BioOptics World. April 2014. Available at: http://www.bioopticsworld.com/articles/2014/04/3d-enabled-leica-microsystems-microscope-performs-ophthalmic-surgery.html . Accessed April 4, 2017.
- Eckardt C, Paulo EB. Heads-up surgery for vitreoretinal procedures: an experimental and clinical study. Retina. 2015, July 21. [Epub ahead of print]