Fundus Autofluorescence in Retinal Disease: A Review and Perspectives
The second of a two-part series
MADELINE YUNG, MD • DAVID SARRAF, MD
In the first part of this series, which appeared in the last issue of Retinal Physician, we provided an overview of fundus autofluorescence (FAF) imaging technologies (Table), and we reviewed the factors that produce and can affect fluorescence. In this second part, we apply these concepts to specific retinal conditions.
IMAGING MODALITY | FUNDUS AUTOFLUORESCENCE IMAGING SYSTEMS | EXCITATION WAVELENGTH | BARRIER FILTER | FIELD OF VIEW | ADVANTAGES | DISADVANTAGES |
---|---|---|---|---|---|---|
Fundus camera | Better for visualizing exudative retinal disease, red-shifted wavelengths decrease absorption by macular pigments, can be used with FA, color imaging, decreased motion artifact, more comfortable for patient | No real-time averaging, poor contrast, capture more reflected and scattered light, prone to pseudoautofluorescence | ||||
Topcon TRC-50DX | 535-585 nm | 615-715 nm | 20, 35, 50 | Nonmydriatic, also offers FA, ICG | ||
Zeiss Visucam 224/524 | 510-580 nm | 650-735 nm | 30, 45 | Nonmydriatic. Visucam 524 with FA and optional ICG | ||
Canon CR-2 plus AF (nonmydriatic) | 530-580 | 640 nm | 35, 45 | Nonmydriatic, also offers cobalt setting | ||
Confocal scanning laser ophthalmoscope (cSLO) | Confocal optics reduces interference from the lens, real-time averaging, high contrast, high resolution, decreased scattered light | Excitation beam is absorbed by macular pigments, cannot be preceded by FA, fixation loss, monochromatic, patient discomfort | ||||
Heidelberg Retinal Angiograph (HRA 2) | 488 nm | 500 nm | 20, 30, 55 | No longer commercially available | ||
Heidelberg Spectralis | 488 nm | 500 nm | 20, 30, 55 | Also offers red-free, FA, ICG, simultaneous FA/ICG, infrared reflectance, multicolor imaging; dual wavelength technology can calculate macular pigment density, spectral-domain OCT | ||
Zeiss prototype SM 30 4024 (ZcSLO) | 488 nm | 521 nm | 20, 40 | No longer commercially available | ||
Rodenstock (RcSLO) | 488 nm | 515 nm | 20, 40 | No longer commercially available | ||
Nidek F-10 | 490 nm | 510 nm | 40, 60 | Also offers multicolor imaging, retro-mode, FA, ICG | ||
Widefield cSLOs | Detects peripheral findings, nonmydriatic, brief image acquisition time, can be used with FA | Disadvantages vary by system and lens | ||||
Optos Ultra-Widefield | 532 nm, 633 nm | 540 nm | 200 | Decreased absorption by macular pigments, also offers color fundus, red-free, FA, ICG | No real-time averaging, poor contrast, distortion of peripheral retina, view limited in superior and inferior quadrants, lid/lash artifact | |
Staurenghi lens | N/A | N/A | 150 | Lens attaches to cSLO | Requires placement of contact lens | |
Heidelberg Ultra-widefield lens | N/A | N/A | 105 | Lens attaches to HRA or Spectralis. High contrast, non-distorted images, no lid/lash artifact, can be used with FA | Smaller field of view, view limited in nasal and temporal quadrants | |
ALTHOUGH SOME SYSTEMS USE MULTIPLE WAVELENGTHS, ONLY THE FAF EXCITATION WAVELENGTH IS PROVIDED. CSLO: CONFOCAL SCANNING LASER OPHTHALMOSCOPE. ICG: INDOCYANINE GREEN ANGIOGRAPHY. FA: FLUORESCEIN ANGIOGRAPHY. OCT: OPTICAL COHERENCE TOMOGRAPHY. COURTESY OF BIOMED CENTRAL. |
RETINAL DISEASES WITH CHARACTERISTIC FAF FINDINGS
Age-related Macular Degeneration
Age-related macular degeneration has been characterized by FAF, and unique FAF findings not otherwise seen with funduscopy or other imaging systems are valuable in determining the diagnosis, management, and prognosis of the disease.
In early, non-neovascular AMD, FAF can show both hyper- and hypoautofluorescent foci, often revealing more widespread disease than identified with clinical examination. In 2005, an international FAF classification group designated eight FAF patterns seen in early AMD: normal, minimal change, focal increase, lace-like, speckled, patchy, linear, and reticular. The patchy phenotype, as well as linear and reticular patterns, is associated with increased risk for the development of choroidal neovascularization.1
Madeline Yung, MD, is an intern at Olive View-UCLA Medical Center in Los Angeles, CA. David Sarraf, MD, is on the faculty of the Stein Eye Institute at UCLA. Dr. Sarraf has received research grants from Allergan, Genentech, Optovue, and Regeneron, and he is a consultant to Genentech and Optovue. Dr. Yung reports no financial interests. Dr. Sarraf can be reached via e-mail at dsarraf@g.ucla.edu.
The FAF appearance of drusen varies substantially depending on drusen size and type, as well as the health of the overlying retinal pigment epithelium. Small drusen show minimal changes.2 Intermediate drusen (65-125 nm) show central hypoautofluorescence from RPE atrophy and a hyperautofluorescent border representing abnormal RPE cells.3 Large drusen and drusenoid pigment epithelial detachments (PEDs) are hyperautofluorescent.2
Cuticular and crystalline drusen are hypoautofluorescent.4,5 Reticular pseudodrusen, referring to deposits above the RPE, present as small, round, hypoautofluorescent foci with interspersed reticular hyperautofluorescence.6 Of these entities, drusenoid pigment epithelial detachments and reticular pseudodrusen confer the greatest risk for disease progression.7,8
On FAF, geographic atrophy manifests as sharply demarcated areas of hypoautofluorescence due to RPE atrophy. Holz et al identified five different patterns of perilesional hyperautofluorescence that can surround areas of geographic atrophy — none, focal, banded, patchy, and diffuse — with the diffuse pattern further subdivided into reticular, branching, trickling, fine granular, and fine granular with peripheral punctate spots.9 The banded and diffuse trickling patterns have been associated with high risk for disease progression.
Early CNV can cause minimal FAF changes due to intact RPE and photoreceptor layers10 or hypoautofluorescence due to atrophy of the overlying RPE or a blocking effect.11 Retinal hemorrhage or exudates initially absorb light, resulting in decreased autofluorescence, but they become hyperautofluorescent after degradation.12,13 Patients may also have a hyperautofluorescent ring of RPE especially encircling type 2 neovascularization and in association with subretinal fluid and photoreceptor degeneration.14
As a well-known complication of AMD, RPE tears present as a wedge- or crescent-shaped area of remarkable hypoautofluorescence with sharp, irregular borders representing the zone of denuded RPE, and adjacent hyperautofluorescence at the site of rolled retracted RPE (Figure 1). Over time, RPE tears undergo resurfacing, with recovery of autofluorescence and associated visual function occurring centripetally toward the center of the lesion (Figure 2).15-18
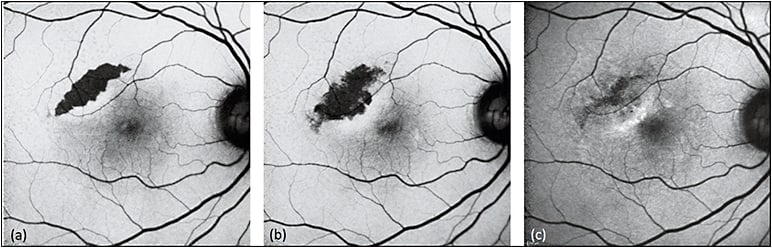
Figure 1. Sequential FAF of RPE tear. RPE tears (a) appear as well-demarcated central hypoautofluorescence due to absent RPE with adjacent irregular hyperautofluorescence corresponding to the retracted edges of RPE. Serial images obtained three weeks (b) and one year (c) later show resurfacing and remodeling of the lesion, with centripetal recovery of autofluorescence extending from the borders.
COURTESY OF BIOMED CENTRAL.
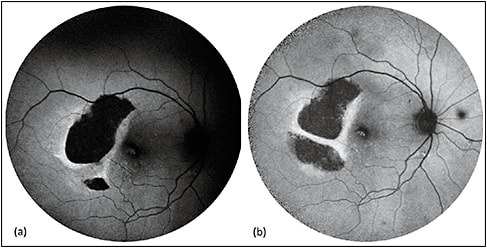
Figure 2. Sequential FAF of RPE tear treated with aflibercept. RPE tears (a) appear as a well-demarcated central hypoautofluorescence due to absent RPE with adjacent irregular hyperautofluorescence corresponding to the retracted edges of RPE. The patient received anti-VEGF therapy with half-dose aflibercept. Over time (b), the lesion shows evidence of remodeling and resurfacing, with centripetal recovery of autofluorescence.
COURTESY OF WOLTERS KLUWER
Central Serous Chorioretinopathy
In central serous chorioretinopathy, RPE and choroidal dysfunction results in accumulation of subretinal fluid and serous retinal detachment. Near-infrared (NI)-FAF has superior sensitivity over short-wavelength (SW)-FAF in detecting abnormalities,19 and ultrawidefield FAF is an essential component of imaging evaluation because 57% of CSC cases involve the peripheral retina.20,21
The initial formation of serous retinal detachment causes hypoautofluorescence due to blockage by subretinal fluid.22-24 As photoreceptor debris and macrophages accumulate in the form of subretinal precipitates, the serous detachment develops a granular or stippled hyperautofluorescence, most notably at the margins (Figure 3). With time, there is gravitation of the fluid and the autofluorescent findings inferiorly.24,25 Chronic cases lasting more than six months are associated with hypoautofluorescent, atrophic gravitational tracts.23,25
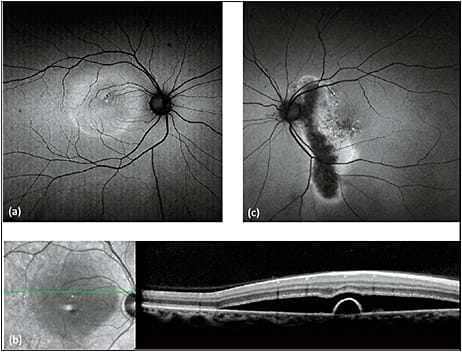
Figure 3. FAF in central serous chorioretinopathy. FAF (a) of a patient with acute exacerbation of CSC shows macular detachment, with hyperautofluorescent material at the margin and inferior region of the detachment. SD-OCT (b) of the lesion shows a serous retinal detachment associated with a small pigment epithelial detachment. FAF of the patient’s asymptomatic father (c) incidentally revealed an atrophic hypoautofluorescent gravitational tract from chronic inactive CSC with hyperautofluorescent margins.
COURTESY OF BIOMED CENTRAL.
Stargardt Macular Dystrophy
Stargardt disease is a hereditary juvenile macular dystrophy resulting from autosomal recessive mutations in the ABCA4 gene and, in rare cases, autosomal dominant mutations in the ELOVL4 gene. The salient retinal findings include central macular atrophy (hypoautofluorescent on FAF) in a bull’s eye or geographic pattern and surrounding yellow flecks that exhibit peripapillary sparing.26,27 Active flecks are hyperautofluorescent, while resorbed flecks are hypoautofluorescent (Figure 4).
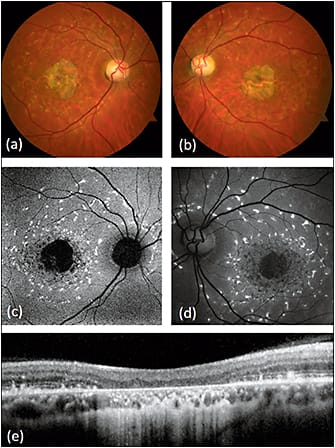
Figure 4. Fundus autofluorescence of Stargardt disease. While fundus photography (a,b) and clinical examination may have nonspecific findings, fundus autofluorescence (c,d) shows hyperautofluorescent flecks with peripapillary sparing characteristic of the disease. The flecks surround a hypoautofluorescent central macula corresponding to significant chorioretinal atrophy on SD-OCT (e).
COURTESY OF BIOMED CENTRAL.
FAF is sensitive for abnormalities early in the disease course and can be used in the diagnostic evaluation for Stargardt disease.28
Cideciyan et al proposed a six-stage disease model that correlates well with FAF findings, in which early stages involve a general increase in lipofuscin and hyperautofluorescence, intermediate stages involve broad variation in the mean intensity and texture of autofluorescence, and late stages involve hypoautofluorescent chorioretinal atrophy in the macula with hyperautofluorescent flecks in the midperiphery.27-30
Best Macular Dystrophy
Autosomal dominant mutations in the BEST1 gene cause early-onset, bilateral vitelliform lesions in the macula with associated vision loss.31,32 FAF findings have been defined for the five clinical stages of disease progression.33 Previtelliform lesions have zero to minimally increased autofluorescence, vitelliform lesions are hyperautofluorescent, the pseudohypopyon stage shows a hyperautofluorescent layer gravitating inferiorly, the vitelliruptive lesion is hypoautofluorescent and bordered by hyperautofluorescent condensations, and the atrophic stage appears as diffuse hypoautofluorescence.33
Pattern Dystrophies
Pattern dystrophies encompass a collection of late-onset macular dystrophies with a stable, benign course, primarily resulting from autosomal dominant mutations in the PRPH2 gene (formerly the RDS gene).34 These dystrophies display a wide range of phenotypic variability, and FAF can be useful in disease diagnosis.35
Adult onset vitelliform dystrophy (AOVD) manifests as small, bilateral, hyperautofluorescent vitelliform lesions that are usually subfoveal but can be multifocal.36 As the disease progresses, RPE atrophy causes regions of hypoautofluorescence.37
Parodi et al found that AOVD progressed through normal, then focal, and finally patchy SW-FAF patterns, which correlate with deteriorating visual function.38 NI-FAF is more sensitive than SW-FAF for the detection of abnormalities.
As suggested by its name, multifocal pattern dystrophy simulating fundus flavimaculatus presents as a flecked retina syndrome, with hyperautofluorescent flecks at the posterior pole and vascular arcades. However, unlike Stargardt, this disease has an autosomal dominant inheritance, late onset, normal appearance of the choroid, and absence of hypoautofluorescent atrophy of the central macula.39
Other pattern dystrophies, including butterfly pigment dystrophy, fundus pulverulentus, and reticular dystrophy, are not yet well characterized by FAF. However, FAF can be used to identify more sensitively the remarkable hyperautofluorescent butterfly or reticular patterns characteristic of these diseases.
Retinitis Pigmentosa
Retinitis pigmentosa (RP) refers to a genetically heterogeneous spectrum of diseases that result in rod photoreceptor degeneration. Common autosomal dominant mutations include the RHO gene, autosomal recessive mutations include the USH2A gene, and X-linked mutations include the RPGR and RP2 genes. Although electroretinography remains the gold standard for the diagnosis and monitoring of RP, it becomes less reliable in advanced stages of disease.40 FAF can be used in lieu of electroretinography to monitor disease progression.41
On FAF, a large subset of patients with RP demonstrates a hyperautofluorescent Robson-Holder ring, which represents the border of active outer segment dysgenesis and abnormal lipofuscin production (Figure 5).42-45
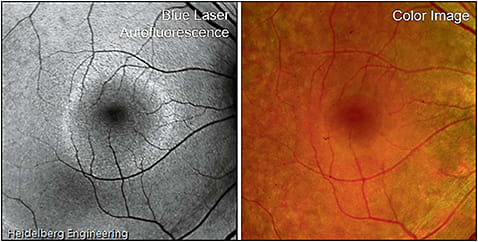
Figure 5. Robson-Holder ring in retinitis pigmentosa. FAF (left) of retinitis pigmentosa shows an area of normal preserved retina at the posterior fundus bordered by a hyperautofluorescent Robson-Holder ring, a characteristic finding visible on FAF but not on fundus photography (right).
COURTESY OF HEIDELBERG ENGINEERING
Optical coherence tomography studies have shown photoreceptor loss outside the ring and normal retina within. In addition, the diameter of the ring corresponds with retinal sensitivity and visual field constriction — the more the ring encroaches centrally, the more constricted the visual field is.46
Serial FAF imaging of the Robson-Holder ring provides an accurate measurement of disease progression.43 Similar rings are also seen in other retinal dystrophies, including Leber congenital amaurosis, Best macular dystrophy, and cone dystrophy.47
Choroideremia
Arising from an X-linked mutation in the CHM gene, choroideremia is associated with choroidal, RPE, and photoreceptor atrophy with macular sparing, leading to night blindness and visual field constriction.48,49 FAF shows bilateral midperipheral zones of hypoautofluorescence with scalloped edges bordering a central stellate area of preserved autofluorescence (Figure 6).50
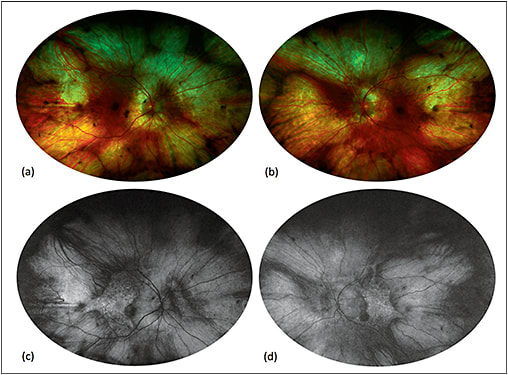
Figure 6. Fundus autofluorescence of choroideremia. Fundus photography (a, b) shows a pale fundus with zones of choroidal atrophy in the midperiphery, revealing the sclera underneath. Fundus autofluorescence (c, d) demonstrates corresponding zones of hyperautofluorescence given the autofluorescent properties of the sclera. Note the central stellate island of preserved choriocapillaris and retinal pigment epithelium in each eye, which is very characteristic of choroideremia.
COURTESY OF SRINIVAS SADDA, MD
Interestingly, female carriers of the CHM mutation are asymptomatic but may demonstrate a peripheral speckled pattern of hyper- and hypoautofluorescence, underscoring the value of FAF in evaluating the female relatives of affected patients.49,51
Fundus Albipunctatus
Due to an autosomal recessive mutation in the RDH5 gene causing defective rhodopsin recycling and delayed regeneration of visual pigments, fundus albipunctatus is associated with delayed dark adaptation and stationary night blindness.52,53 Decreased lipofuscin production results in severely attenuated background autofluorescence and grainy FAF images (Figure 7).54,55
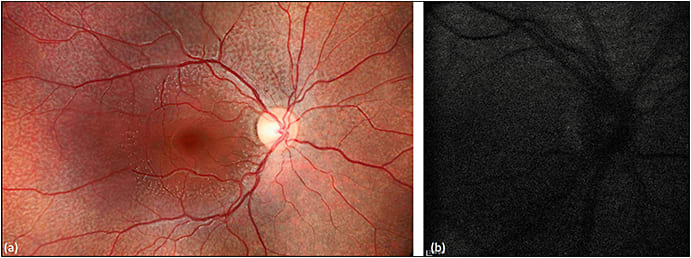
Figure 7. Fundus autofluorescence of fundus albipunctatus. Fundus photography (a) demonstrates multifocal white flecks in the midperiphery with macular sparing. FAF (b) shows profoundly decreased background autofluorescence and a grainy resolution.
COURTESY OF BIOMED CENTRAL.
The white spots seen on funduscopy correlate with the foci of relatively enhanced autofluorescent signals, although these foci are often not visible on FAF given the overall decreased background autofluorescence.56 Other flecked retina syndromes not associated with an RDH5 mutation produce hyperautofluorescent dots with FAF. Hyperautofluorescent crescents and concentric parafoveal rings have also been reported with fundus albipunctatus.
Other retinal diseases involving mutations in the same visual cycle pathway include retinitis punctata albescens and RPE65 mutations. Retinitis punctata albescens arises from an autosomal recessive mutation in the RLBP1 gene, causing white, hyperautofluorescent, punctate retinal lesions and severe progressive night blindness.57 RPE65 mutations also decrease background autofluorescence, but they perpetrate more severe disease than fundus albipunctatus.58,59
White Dot Syndromes
White dot syndromes include a diverse collection of inflammatory chorioretinopathies with the common phenotype of multifocal white or yellow spots in the posterior pole and peripheral fundus. White dot syndromes vary significantly in pathogenesis, treatment, and prognosis.60 FAF and widefield FAF are sensitive tools for detecting lesions and differentiating diseases.61,62
Multiple evanescent white dot syndrome (MEWDS) is a self-limited, unilateral disorder that affects young, healthy women. Funduscopy shows multifocal 100- to 200-µm white spots in the paramacular and midperipheral fundus, which are hyperautofluorescent due to a window defect from photoreceptor loss (Figure 8).63 FAF can show hypoautofluorescence at the fovea, and it is more sensitive than clinical exam or fluorescein angiography for detecting lesions.64
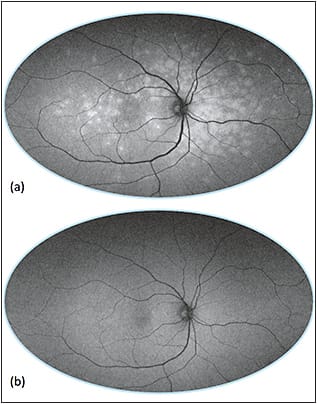
Figure 8. Ultra-widefield imaging of multiple evanescent white dot syndrome (MEWDS). Ultra-widefield FAF of active disease (a) with multifocal hyperautofluorescence and resolution (b) six weeks later.
COURTESY OF BIOMED CENTRAL.
Punctate inner choroidopathy occurs in young, myopic female subjects and is a diagnosis of exclusion, occurring in the absence of uveitis or presumed ocular histoplasmosis. The white dots represent sub-RPE nodules that can have minimal hyperautofluorescence.65
Widefield FAF has been used to detect clinically occult hyperautofluorescent lesions indicative of active or recurrent disease, and it can be used to guide management.66 As the nodules break through the RPE, the lesions may become hypoautofluorescent with a hyperautofluorescent margin.67
Punctate inner choroidopathy may be associated with CNV, which has similar FAF findings to the CNV in AMD.65 Ninety percent of patients with diffuse hyperautofluorescent patches were found to have concurrent MEWDS on multimodal imaging.67
Hydroxychloroquine Toxicity
Hydroxychloroquine (Plaquenil, Sanofi, Bridgewater, NJ) is an inexpensive, well-tolerated agent to treat systemic rheumatological diseases, but its risk for ocular toxicity rises sharply with cumulative doses greater than 1,000 g.
In Caucasians, toxicity manifests as parafoveal photoreceptor loss with foveal sparing, resulting in a hyperautofluorescent parafoveal ring due to the window defect, with decreasing autofluorescence over time as the RPE progressively atrophies, and in advanced cases, progression to a characteristic bull’s eye maculopathy.68
Recent studies have found that Asians tend to have a more eccentric pericentral pattern of toxicity (a pericentral hyperautofluorescent ring is identified with FAF), with a hazard ratio of 27.1, which is more difficult to recognize on standard screening.69
The 2016 guidelines from the American Academy of Ophthalmology recommend a baseline examination and then annual screening with spectral-domain optical coherence tomography and perimetry starting after five years of standard use.70 FAF can be used as a supplemental imaging modality to detect early photoreceptor degeneration, to provide a topographic map of retinal toxicity, and to show extramacular patterns in Asian eyes.70
CONCLUSION
FAF is a noninvasive retinal imaging modality with rapid acquisition that detects the natural autofluorescence of ocular fluorophores. SW-FAF reflects the topography of lipofuscin and can be visualized using several imaging systems, including fundus cameras, confocal scanning laser ophthalmoscopes, and ultrawidefield systems.
FAF can detect abnormalities not otherwise identified with clinical examination, color fundus photography, FA, spectral-domain optical coherence tomography, and other techniques, and it can identify signature phenotypes facilitating the diagnosis of a wide variety of retinal disorders. In short, FAF has become a rapidly evolving and valuable tool in the evaluation and management of retinal disease. RP
REFERENCES
1. Batioglu F, Demirel S, Ozmert E, Oguz YG, Ozyol P. Autofluorescence patterns as a predictive factor for neovascularization. Optom Vis Sci. 2014;91:950-955.
2. Lois N, Owens SL, Coco R, Hopkins J, Fitzke FW, Bird AC. Fundus autofluorescence in patients with age-related macular degeneration and high risk of visual loss. Am J Ophthalmol. 2002;133:341-349.
3. Delori FC, Fleckner MR, Goger DG, Weiter JJ, Dorey CK. Autofluorescence distribution associated with drusen in age-related macular degeneration. Invest Ophthalmol Vis Sci. 2000;41:496-504.
4. Spaide RF, Curcio CA. Drusen characterization with multimodal imaging. Retina. 2010;30:1441-1454.
5. Holz FG, Steinberg JS, Gobel A, Fleckenstein M, Schmitz-Valckenberg S. Fundus autofluorescence imaging in dry AMD: 2014 Jules Gonin lecture of the Retina Research Foundation. Graefes Arch Clin Exp Ophthalmol. 2015;253:7-16.
6. Schmitz-Valckenberg S, Steinberg JS, Fleckenstein M, Visvalingam S, Brinkmann CK, Holz FG. Combined confocal scanning laser ophthalmoscopy and spectral-domain optical coherence tomography imaging of reticular drusen associated with age-related macular degeneration. Ophthalmology. 2010;117:1169-1176.
7. Mrejen S, Sarraf D, Mukkamala SK, Freund KB. Multimodal imaging of pigment epithelial detachment: a guide to evaluation. Retina. 2013;33:1735-1762.
8. Pumariega NM, Smith RT, Sohrab MA, Letien V, Souied EH. A prospective study of reticular macular disease. Ophthalmology. 2011;118:1619-1625.
9. Holz FG, Bindewald-Wittich A, Fleckenstein M, Dreyhaupt J, Scholl HP, Schmitz-Valckenberg S. Progression of geographic atrophy and impact of fundus autofluorescence patterns in age-related macular degeneration. Am J Ophthalmol. 2007;143:463-472.
10. Vaclavik V, Vujosevic S, Dandekar SS, Bunce C, Peto T, Bird AC. Autofluorescence imaging in age-related macular degeneration complicated by choroidal neovascularization: a prospective study. Ophthalmology. 2008;115:342-346.
11. McBain VA, Townend J, Lois N. Fundus autofluorescence in exudative age-related macular degeneration. Br J Ophthalmol. 2007;91:491-496.
12. Guerra RL, Silva IS, Marback EF, Maia Ode O Jr, Marback RL. Fundus autofluorescence in blunt ocular trauma. Arq Bras Oftalmol. 2014;77:139-142.
13. Sawa M, Ober MD, Spaide RF. Autofluorescence and retinal pigment epithelial atrophy after subretinal hemorrhage. Retina. 2006;26:119-120.
14. Camacho N, Barteselli G, Nezgoda JT, et al. Significance of the hyperautofluorescent ring associated with choroidal neovascularisation in eyes undergoing anti-VEGF therapy for wet age-related macular degeneration. Br J Ophthalmol. 2015;99:1277-1283.
15. Mendis R, Lois N. Fundus autofluorescence in patients with retinal pigment epithelial (RPE) tears: an in-vivo evaluation of RPE resurfacing. Graefes Arch Clin Exp Ophthalmol. 2014;252:1059-1063.
16. Sarraf D, Reddy S, Chiang A, Yu F, Jain A. A new grading system for retinal pigment epithelial tears. Retina. 2010;30:1039-1045.
17. Sarraf D, Joseph A, Rahimy E. Retinal pigment epithelial tears in the era of intravitreal pharmacotherapy: risk factors, pathogenesis, prognosis and treatment (an American Ophthalmological Society thesis). Trans Am Ophthalmol Soc. 2014;112:142-159.
18. Nagiel A, Sadda SR, Schwartz SD, Sarraf D. Resolution of a giant pigment epithelial detachment with half-dose aflibercept. Retin Cases Brief Rep. 2015;9:269-272.
19. Zhang P, Wang H-Y, Zhang Z-F, et al. Fundus autofluorescence in central serous chorioretinopathy: association with spectral-domain optical coherence tomography and fluorescein angiography. Int J Ophthalmol. 2015;8:1003-1007.
20. Pang CE, Shah VP, Sarraf D, Freund KB. Ultra-widefield imaging with autofluorescence and indocyanine green angiography in central serous chorioretinopathy. Am J Ophthalmol. 2014;158:362-371.
21. Shin JY, Choi HJ, Lee J, Choi M, Chung B, Byeon SH. Fundus autofluorescence findings in central serous chorioretinopathy using two different confocal scanning laser ophthalmoscopes: correlation with functional and structural status. Graefes Arch Clin Exp Ophthalmol. 2016;254:1537-1544.
22. Dinc UA, Tatlipinar S, Yenerel M, Gorgun E, Ciftci F. Fundus autofluorescence in acute and chronic central serous chorioretinopathy. Clin Exp Optom. 2011;94:452-457.
23. Teke MY, Elgin U, Nalcacioglu-Yuksekkaya P, Sen E, Ozdal P, Ozturk F. Comparison of autofluorescence and optical coherence tomography findings in acute and chronic central serous chorioretinopathy. Int J Ophthalmol. 2014;7:350-354.
24. Iacono P, Battaglia PM, Papayannis A, La Spina C, Varano M, Bandello F. Acute central serous chorioretinopathy: a correlation study between fundus autofluorescence and spectral-domain OCT. Graefes Arch Clin Exp Ophthalmol. 2015;253:1889-1897.
25. Spaide RF, Klancnik JM Jr. Fundus autofluorescence and central serous chorioretinopathy. Ophthalmology. 2005;112:825-833.
26. Zhang K, Kniazeva M, Han M, et al. A 5-bp deletion in ELOVL4 is associated with two related forms of autosomal dominant macular dystrophy. Nat Gen. 2001;27:89-93.
27. Lois N, Halfyard AS, Bird AC, Holder GE, Fitzke FW. Fundus autofluorescence in Stargardt macular dystrophy-fundus flavimaculatus. Am J Ophthalmol. 2004;138:55-63.
28. Boon CJ, Jeroen Klevering B, Keunen JE, Hoyng CB, Theelen T. Fundus autofluorescence imaging of retinal dystrophies. Vision Res. 2008;48:2569-2577.
29. Cideciyan AV, Aleman TS, Swider M, et al. Mutations in ABCA4 result in accumulation of lipofuscin before slowing of the retinoid cycle: a reappraisal of the human disease sequence. Hum Mol Genet. 2004;13:525-534.
30. Burke TR, Duncker T, Woods RL, et al. Quantitative fundus autofluorescence in recessive Stargardt disease. Invest Ophthalmol Vis Sci. 2014;55:2841-2852.
31. Parodi MB, Iacono P, Campa C, Del Turco C, Bandello F. Fundus autofluorescence patterns in Best vitelliform macular dystrophy. Am J Ophthalmol. 2014;158:1086-1092.
32. Bakall B, Radu RA, Stanton JB, et al. Enhanced accumulation of A2E in individuals homozygous or heterozygous for mutations in BEST1 (VMD2). Exp Eye Res. 2007;85:34-43.
33. Querques G, Zerbib J, Georges A, et al. Multimodal analysis of the progression of Best vitelliform macular dystrophy. Mol Vis. 2014;20:575-592.
34. Molday RS, Hicks D, Molday L. Peripherin. A rim-specific membrane protein of rod outer segment discs. Invest Ophthalmol Vis Sci. 1987;28:50-61.
35. Renner AB, Fiebig BS, Weber BHF, et al. Phenotypic variability and long-term follow-up of patients with known and novel PRPH2/RDS gene mutations. Am J Ophthalmol. 2009;147:518-530.e511.
36. Renner AB, Tillack H, Kraus H, et al. Morphology and functional characteristics in adult vitelliform macular dystrophy. Retina. 2004;24:929-939.
37. Chowers I, Tiosano L, Audo I, Grunin M, Boon CJ. Adult-onset foveomacular vitelliform dystrophy: A fresh perspective. Prog Retin Eye Res. 2015;47:64-85.
38. Parodi MB, Iacono P, Pedio M, et al. Autofluorescence in adult-onset foveomacular vitelliform dystrophy. Retina. 2008;28:801-807.
39. Boon CJ, Klevering BJ, den Hollander AI, et al. Clinical and genetic heterogeneity in multifocal vitelliform dystrophy. Arch Ophthalmol. 2007;125:1100-1106.
40. Mitamura Y, Mitamura-Aizawa S, Nagasawa T, Katome T, Eguchi H, Naito T. Diagnostic imaging in patients with retinitis pigmentosa. J Med Invest. 2012;59:1-11.
41. Ogura S, Yasukawa T, Kato A, et al. Wide-field fundus autofluorescence imaging to evaluate retinal function in patients with retinitis pigmentosa. Am J Ophthalmol. 2014;158:1093-1098.
42. Murakami T, Akimoto M, Ooto S, et al. Association between abnormal autofluorescence and photoreceptor disorganization in retinitis pigmentosa. Am J Ophthalmol. 2008;145:687-694.
43. Robson AG, Tufail A, Fitzke F, et al. Serial imaging and structure-function correlates of high-density rings of fundus autofluorescence in retinitis pigmentosa. Retina. 2011;31:1670-1679.
44. Fleckenstein M, Charbel Issa P, Helb HM, Schmitz-Valckenberg S, Scholl HP, Holz FG. Correlation of lines of increased autofluorescence in macular dystrophy and pigmented paravenous retinochoroidal atrophy by optical coherence tomography. Arch Ophthalmol. 2008;126:1461-1463.
45. Greenstein VC, Duncker T, Holopigian K, et al. Structural and functional changes associated with normal and abnormal fundus autofluorescence in patients with retinitis pigmentosa. Retina. 2012;32:349-357.
46. Popovic P, Jarc-Vidmar M, Hawlina M. Abnormal fundus autofluorescence in relation to retinal function in patients with retinitis pigmentosa. Graefes Arch Clin Exp Ophthalmol. 2005;243:1018-1027.
47. Fleckenstein M, Charbel Issa P, Fuchs HA, et al. Discrete arcs of increased fundus autofluorescence in retinal dystrophies and functional correlate on microperimetry. Eye (Lond). 2009;23:567-575.
48. Seitz IP, Zhour A, Kohl S, et al. Multimodal assessment of choroideremia patients defines pre-treatment characteristics. Graefes Arch Clin Exp Ophthalmol. 2015;253:2143-2150.
49. Pichi F, Morara M, Veronese C, Nucci P, Ciardella AP. Multimodal imaging in hereditary retinal diseases. J Ophthalmol. 2013;2013:634351.
50. Morgan JI, Han G, Klinman E, et al. High-resolution adaptive optics retinal imaging of cellular structure in choroideremia. Invest Ophthalmol Vis Sci. 2014;55:6381-6397.
51. Preising MN, Wegscheider E, Friedburg C, Poloschek CM, Wabbels BK, Lorenz B. Fundus autofluorescence in carriers of choroideremia and correlation with electrophysiologic and psychophysical data. Ophthalmology. 2009;116:1201-1209.
52. Zeitz C, Robson AG, Audo I. Congenital stationary night blindness: an analysis and update of genotype-phenotype correlations and pathogenic mechanisms. Prog Retin Eye Res. 2015;45:58-110.
53. Yamamoto H, Simon A, Eriksson U, Harris E, Berson EL, Dryja TP. Mutations in the gene encoding 11-cis retinol dehydrogenase cause delayed dark adaptation and fundus albipunctatus. Nat Gen. 1999;22:188-191.
54. Schatz P, Preising M, Lorenz B, et al. Lack of autofluorescence in fundus albipunctatus associated with mutations in RDH5. Retina. 2010;30:1704-1713.
55. Wang NK, Chuang LH, Lai CC, et al. Multimodal fundus imaging in fundus albipunctatus with RDH5 mutation: a newly identified compound heterozygous mutation and review of the literature. Doc Ophthalmol. 2012;125:51-62.
56. Sergouniotis PI, Sohn EH, Li Z, et al. Phenotypic variability in RDH5 retinopathy (fundus albipunctatus). Ophthalmology. 2011;118:1661-1670.
57. Genead MA, Fishman GA, Lindeman M. Spectral-domain optical coherence tomography and fundus autofluorescence characteristics in patients with fundus albipunctatus and retinitis punctata albescens. Ophthalmic Genet. 2010;31:66-72.
58. Moiseyev G, Chen Y, Takahashi Y, Wu BX, Ma JX. RPE65 is the isomerohydrolase in the retinoid visual cycle. Proc Natl Acad Sci U S A. 2005;102:12413-12418.
59. Lorenz B, Wabbels B, Wegscheider E, Hamel CP, Drexler W, Preising MN. Lack of fundus autofluorescence to 488 nanometers from childhood on in patients with early-onset severe retinal dystrophy associated with mutations in RPE65. Ophthalmology. 2004;111:1585-1594.
60. Crawford CM, Igboeli O. A review of the inflammatory chorioretinopathies: the white dot syndromes. ISRN Inflamm. 2013;2013:783190.
61. Furino C, Boscia F, Cardascia N, Alessio G, Sborgia C. Fundus autofluorescence and multiple evanescent white dot syndrome. Retina. 2009;29:60-63.
62. Yenerel NM, Kucumen B, Gorgun E, Dinc UA. Atypical presentation of multiple evanescent white dot syndrome (MEWDS). Ocul Immunol Inflamm. 2008;16:113-115.
63. Joseph A, Rahimy E, Freund KB, Sorenson JA, Sarraf D. Fundus autofluorescence and photoreceptor bleaching in multiple evanescent white dot syndrome. Ophthalmic Surg Lasers Imaging Retina. 2013;44:588-592.
64. Marsiglia M, Gallego-Pinazo R, Cunha de Souza E, et al. Expanded clinical spectrum of multiple evancescent white dot syndrome with multimodal imaging. Retina. 2016;36:64-71.
65. Spaide RF, Goldberg N, Freund KB. Redefining multifocal choroiditis and panuveitis and punctate inner choroidopathy through multimodal imaging. Retina. 2013;33:1315-1324.
66. Klufas MA, O’Hearn T, Sarraf D. Optical coherence tomography angiography and widefield fundus autofluorescence in punctate inner choroidopathy. Retin Cases Brief Rep. 2015;9:323-326.
67. Li M, Zhang X, Wen F. The fundus autofluorescence spectrum of punctate inner choroidopathy. J Ophthalmol. 2015;2015:202097.
68. Kellner U, Renner AB, Tillack H. Fundus autofluorescence and mfERG for early detection of retinal alterations in patients using chloroquine/hydroxychloroquine. Invest Ophthalmol Vis Sci. 2006;47:3531-3538.
69. Melles RB, Marmor MF. Pericentral retinopathy and racial differences in hydroxychloroquine toxicity. Ophthalmology. 2015;122:110-116.
70. Marmor MF, Kellner U, Lai TY, Melles RB, Mieler WF; American Academy of Ophthalmology. Recommendations on screening for chloroquine and hydroxychloroquine retinopathy (2016 revision). Ophthalmology. 2016;123:1386-1394.