PEER REVIEWED
Emerging Treatments for Inherited Retinal Degenerations
Targeted therapy goes viral and beyond.
CRISTY A. KU, MD, PhD • RACHEL C. PATEL, BA • PAUL YANG, MD, PhD
Inherited retinal degenerations (IRD) are rare genetic disorders of the retina and/or choroid with a broad spectrum of symptoms, inheritance patterns, ages of onset, rates of progression, and phenotypic characteristics.
For example, even the most common IRD, retinitis pigmentosa (RP), with a worldwide prevalence of 1:4,000,1 is associated with all inheritance patterns, an age of presentation that spans from the first year to the sixth decade of life, and variable severity of progression that can lead to legal blindness over the course of years to many decades.
The combination of relatively low prevalence, high variability of presentation, and necessity of specialized visual diagnostic equipment makes the diagnosis of IRD inherently challenging. However, now more than ever, accurate diagnosis of IRD can have a tremendous impact on the way patients and families are counseled, not only for family planning and diagnosis of affected relatives, but also with regard to the options for participation in the multitude of emerging clinical trials for the treatment of IRD. As such, the scope of this article will not be a comprehensive review, but rather will be limited to the discussion of IRD for which there are currently active treatment trials in the United States.
At tertiary referral centers for IRD, widefield multimodal imaging, spectral domain optical coherence tomography, 90° full-field perimetry, and electroretinography (ERG) are used to complement the ocular exam, history, and pedigree, all of which guide the ordering and interpretation of confirmatory genetic testing and segregation analysis.
Although there are currently more than 250 genes known to be associated with IRD, the list is far from complete. Thus, the genetic testing detection rate has been estimated to be ~50% to 60%,2-4 underscoring the value of clinical suspicion and need for high pretest probability.
Cristy A. Ku, MD, PhD, is a postdoctoral fellow at Casey Eye Institute of the Oregon Health & Science University (OHSU) in Portland. Paul Yang, MD, PhD, serves on the faculty of Casey Eye Institute of OHSU. Rachel C. Patel, BA, at the University of Massachusetts Medical School in Worcester. None of the authors reports any financial interests in products mentioned in this article. Dr. Yang can be reached via e-mail at yangp@ohsu.edu.
While the precision, speed, and cost of genetic sequencing have advanced greatly in past years with the advent of next-generation sequencing and high-throughput strategies, a firm clinical diagnosis is still crucial to the interpretation of the not uncommon scenario of an inconclusive result, due to variations of unknown significance in the gene(s) in question.
That said, there must be a high degree of confidence in both the clinical diagnosis and confirmatory genetic test results for a patient to be eligible for most targeted therapies under clinical trials.
TREATMENT OF IRD
Although there is still no proven cure, rapidly emerging developments in the clinical management and therapy for IRD provide hope for the potential of vision preservation and even functional restoration for these devastating diseases.
The therapeutic options can be broadly categorized into three strategies: (1) slowing retinal degeneration via neuroprotection; (2) stopping disease progression by treating the genetic mutation; and (3) reviving visual function by the replacement of lost tissue or the substitution of photoreceptor function.
Most of the original clinical treatment studies in IRD have investigated the effects of oral nutritional supplementation in large heterogeneous groups of patients with RP. Novel approaches to therapy in IRD have begun targeting biochemical pathways specific to the genetic mutation and disease pathophysiology. In particular, gene and cell-based therapies have received much attention because these two platforms for delivering therapy are relevant across all three treatment strategies (Figure).
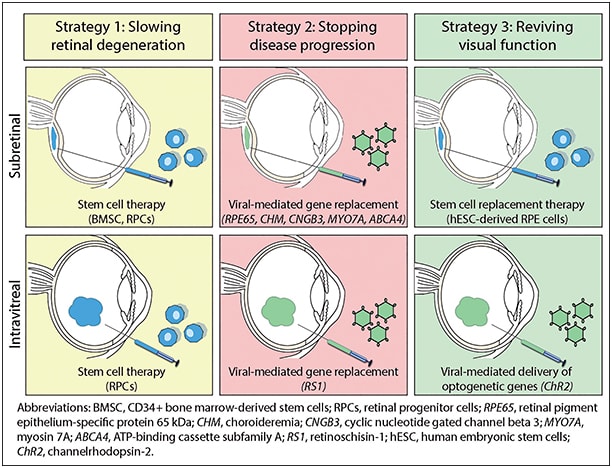
Figure. Gene and cell-based therapies currently in clinical trials in the United States.
The eye is particularly suitable for the delivery of gene and stem cell therapy because the ocular globe is anatomically distinct, readily accessible, and possesses some degree of immune privilege. The emergence of these targeted therapies offers hope for patients with IRD but also underscores the need for accurate diagnosis and genetic confirmation testing.
STRATEGY #1: SLOWING PROGRESSION OF RETINAL DEGENERATION
Slowing disease progression via retinal neuroprotection is mainly based on oral medications and nutritional supplements that are thought to improve the retinoid visual cycle, act as antioxidants, and/or mediate cell death. Cell-based approaches to neuroprotection are being developed to deliver neurotrophic factors that may also have antioxidant properties and promote photoreceptor survival.
Retinoids play a central role in the visual cycle and are obtained from dietary vitamin A as retinol and retinyl esters in meat and dairy or carotenoids in fruits and vegetables.5 The initiation of phototransduction involves the activation of the essential vitamin A-derived chromophore, 11-cis retinal, which must then be regenerated via the retinoid cycle, a series of enzymatic reactions within the photoreceptor outer segments (OS) and retinal pigment epithelium (RPE). Thus, a deficiency of 11-cis retinal due to a lack of dietary vitamin A or genetic mutation affecting the retinoid cycle can cause visual dysfunction and retinal degeneration.5
Most notably, mutations in RPE65- or LRAT- cause deficiencies in 11-cis retinal and are associated with Leber congenital amaurosis (LCA), the most severe early-infantile form of RP. A stable synthetic form of 11-cis-retinal called QLT091001, a 9-cis-retinal analog, has been shown in phase 1b clinical trials to improve visual acuity and visual fields in patients with RPE65- or LRAT-associated LCA.6 The benefits of repeated oral administration of QLT091001 are currently being examined (NCT01521793).
Moreover, genetic mutations in the retinoid cycle can also cause a buildup of retinoid metabolites that leads to cellular damage through the generation of reactive oxygen species and toxic metabolites.
In particular, mutations in the ABCA4 gene associated with Stargardt dystrophy7 and RP8,9 lead to an accumulation of all-trans-retinal and subsequent irreversible formation of the toxic retinoid dimer, N-retinyl-N-retinylidene ethanolamine (A2E), in OS disc membranes.10 Thus, while a proper supply of retinoid precursors is essential for maintaining the visual cycle, an abnormal accumulation of retinoid metabolites can be cytotoxic.
Consequently, caution must be exercised with the prescription of vitamin A in patients with RP, especially those who have not yet been genotyped. High-dose vitamin A palmitate supplementation has been shown to slow the decline of cone photoreceptor function on ERG, but had no significant effect on visual field or visual acuity in patients with RP.11
As such, the practice remains controversial due to opposing opinions12 and the risk of serious side effects (Table 1). In addition, it is universally accepted that high-dose vitamin A is contraindicated in ABCA4-associated Stargardt dystrophy and RP, where accumulation of toxic A2E can be exacerbated.
NEUROPROTECTION | ||||
---|---|---|---|---|
Nutritional supplement | Adult dosage | Indicated IRD | Contraindicated IRD. Systemic precautions. | Dietary sources |
Lutein | 12 mg qd | RP17 | None | Dark leafy greens (spinach, kale, Swiss chard, collards), kelp, zucchini, broccoli, corn |
DHA | 400 mg qd | XLRP18,19, STGD60 | None | Dark fishes (mackerel, tuna, sardines, salmon), halibut, cod, shrimp, scallops |
Vitamin A palmitate* | 15,000 IU qd | RP11 | ABCA4-STGD; cautioned in pregnancy, osteoporosis, hepatic dysfunction | N/A |
*Controversial, please refer to text for discussion | ||||
MANAGEMENT OF CME | ||||
Drug | Adult dosage | Indicated IRD | Notes | |
Dorzolamide 2% | 1 gtt TID | RP53,54, Usher53 | Responders more likely to have arRP or greater initial retinal thickness,4 risk of rebound CME with prolonged use53 | |
Acetazolamide | 500 mg PO qd 250 mg PO BID | RP54, Usher55 | Risk of rebound CME with prolonged use55,59 | |
Triamcinolone acetonide | 0.1 mL (4 mg) intravitreal | RP56 | For CME refractory to topical or oral therapy | |
Abbreviations: IRD, inherited retinal dystrophy; DHA, docosahexaenoic acid; IU, international units; qd, daily; gtt, drop; TID, three times daily; QID, four times daily; BID, twice daily; RP, retinitis pigmentosa; XLRP, X-linked RP; STGD, Stargardt dystrophy; arRP, autosomal recessive RP; CME, cystoid macular edema. |
On the contrary, the formation of toxic A2E is a target for neuroprotective treatment strategies in ABCA4-Stargardt dystrophy. Indeed, a novel oral synthetic retinoid, ALK-001, has been shown to prevent retinoid dimerization and formation of A2E, and is currently in phase 2 clinical trials (NCT02230228, NCT02402660).
Other supplementation strategies for neuroprotection include the use of lutein, zeaxanthin, and docosahexaenoic acid (DHA), which have all been observed to be abnormally low in the blood of patients with RP (Table 1).13,14 Lutein and zeaxanthin are the two main carotenoids in macular pigment that are thought to reduce oxidative damage by absorbing blue light.15
The omega-3 fatty acid DHA is the most abundant OS phospholipid constituent that can act as an antioxidant and mediate cell death. Supplementation with either lutein or DHA has been shown to slow the decline of visual fields in patients with RP or X-linked RP, respectively.14,16-19 The effect of DHA in Stargardt dystrophy is currently being studied in phase 1 clinical trials (NCT00060749).
Another example of a semitargeted approach is valproic acid, which was initially identified in high-throughput studies screening for drugs that could potentially mitigate the misfolding of rhodopsin due to mutations in RHO associated with autosomal dominant RP.20
Retrospective case series reporting off-label use of oral valproic acid in patients with various types of IRD have revealed contradictory results.21,22 The effect of valproic acid in autosomal dominant RP will hopefully be elucidated in phase 2 clinical trials currently nearing completion (NCT01233609).
In addition to oral supplements and medications, the strategy of slowing retinal degeneration can also be delivered intraocularly, utilizing cell and gene-based platforms. While the holy grail and future of stem cell therapy is the revival of visual function by retinal tissue replacement (see Strategy #3), most cell-based clinical trials use various derived stem cells with the intention of slowing degeneration perhaps via neurotrophic interactions or secretion of retinal protective factors.
The first cell-based clinical trials using ciliary neurotrophic factor (CNTF)-secreting encapsulated cell implants to promote photoreceptor survival showed no significant benefits in patients with RP or achromatopsia,23-25 although long-term follow-up studies are ongoing.
Current clinical trials studying the neurotrophic or neuroprotective effects of other cell-based therapies include the intravitreal injection of autologous CD34+ bone marrow-derived stem cells (NCT01736059), or the intravitreal and subretinal delivery of retinal progenitor cells (NCT02320812, NCT02464436).
While clinical trials for gene-based delivery of neurotrophic factors is not yet active, one promising technique currently being developed will use viral vectors to deliver a gene, Nxnl1, which encodes two isoforms of the rod-derived cone viability factor that promotes cone cell survival and reduces oxidative stress byproducts.26
STRATEGY #2: STOPPING DISEASE PROGRESSION
Stopping disease progression in IRD consists of the direct intervention of the genetic mutation through retinal gene replacement and/or gene suppression techniques. Thus, this strategy is intrinsically only applicable to cases of IRD for which the genetic etiology is known and the diagnosis has been confirmed with genetic testing.
Autosomal and X-linked recessive IRDs are typically associated with a loss-of-function protein, which is well-suited for treatment with retinal gene replacement. By comparison, autosomal dominant IRDs are fundamentally more difficult to treat, requiring not only retinal gene replacement, but also the development of even more sophisticated techniques to suppress the dominant negative or toxic effects of the mutant gene. Thus, recessive IRDs are the focus of current retinal gene therapy clinical trials, which are discussed as follows (Table 2).
DISEASE GENE/(VECTOR) | PHASE | STATUS | SPONSOR: US CLINICAL TRIAL SITES | NCT# |
---|---|---|---|---|
Leber congenital amaurosis (LCA) | ||||
RPE65 / (AAV2) | I | Active, not recruiting | Samuel G. Jacobson: U. Florida, UPenn | 00481546 |
RPE65 / (AAV2) | I | Active, not recruiting | Spark Therapeutics: CHOP | 00516477 |
RPE65 / (AAV2) | I/II | Active, not recruiting | AGTC: OHSU Casey Eye Institute, U. Mass | 00749957 |
RPE65 / (AAV2) | III | Active, not recruiting | Spark Therapeutics: CHOP, U. Iowa | 00999609 |
Choroideremia (CHM) | ||||
CHM / (AAV2) | I/II | Recruiting | Spark Therapeutics: Mass Eye and Ear, U. Penn, CHOP | 02341807 |
CHM / (AAV2) | II | Recruiting | Byron Lam: Bascom Palmer | 02553135 |
X-linked retinoschisis (XLRS) | ||||
RS1 / (AAV8) | I/II | Recruiting | NEI: NIH Clinical Center | 02317887 |
RS1 / (AAV2tYF) | I/II | Recruiting | AGTC: OHSU Casey Eye Institute, Mass Eye and Ear, U. Michigan, Retina Foundation of the Southwest | 02416622 |
Achromatopsia (ACHM) | ||||
CNGB3 / (AAV2tYF) | I/II | Recruiting | AGTC: OHSU Casey Eye Institute, VitreoRetinal Associates, Bascom Palmer, Pangere Center, Medical College of Wisconsin | 02599922 |
Stargardt (STGD) | ||||
ABCA4 / (EIAV) | I/II | Recruiting | Sanofi: OHSU Casey Eye Institute, Baylor | 01367444 |
Usher syndrome (USH) | ||||
MYO7A / (EIAV) | I/II | Recruiting | Sanofi: OHSU Casey Eye Institute | 01505062 |
Retinitis pigmentosa (RP) – Optogenetics | ||||
Channelrhodopsin-2 (ChR2) / (AAV) | I/II | Recruiting | RetroSense Therapeutics: Retina Foundation of the Southwest | 02556736 |
Modified from Ku et al.61 Abbreviations: IRD, inherited retinal degenerations; RPE65, retinal pigment epithelium-specific protein 65kDa; AAV, adeno-associated virus; ABCA4, ATP-binding cassette, sub-family A, member 4; EIAV, equine infectious anemia virus; MYO7A, myosin VIIA; CHM, choroideremia, rab escort protein 1; RS1, retinoschisin 1; CNGB3, cyclic nucleotide gated channel beta 3; U. Florida, University of Florida, U. Penn, University of Pennsylvania; CHOP, Children’s Hospital of Philadelphia; AGTC, Applied Genetic Technologies Corp.; OHSU, Oregon Health & Science University; U. Mass, University of Massachusetts, Worcester; U. Iowa, University of Iowa; Mass Eye and Ear, Massachusetts Eye and Ear Infirmary; Bascom Palmer, Bascom Palmer Eye Institute; NEI, National Eye Institute; NIH, National Institutes of Health; U. Michigan, University of Michigan; VitreoRetinal Associates, VitreoRetinal Associates, Gainesville, FL; Pangere Center, Pangere Center for Inherited Retinal Diseases. |
In 2008, three independent first-in-human retinal gene therapy phase 1 clinical trials were initiated at University College London (UCL), Children’s Hospital of Philadelphia (CHOP), and the Universities of Pennsylvania and Florida (UPenn/UF). Retinal gene replacement therapy utilizing adeno-associated virus (AAV) expressing RPE65 was injected into the subretinal space of one eye in patients with autosomal recessive LCA associated with mutations in RPE65.27-29 Short-term results from all three phase 1 trials reported not only safety, but improved sensitivity to light.
Phase 1/2 dose-escalation studies from CHOP and UPenn/UF further demonstrated improvement in visual acuity, sensitivity, and navigational testing.30-32 After 9-12 months post-treatment, four patients at UPenn/UF even developed a shift in fixation preference to the site of the subretinal injection.33,34 Furthermore, three patients at CHOP underwent subsequent treatment of the contralateral eye without significant inflammatory response, signifying the relative safety of repeated vector exposure.35
Not surprisingly, some limitations of this first generation RPE65 retinal gene replacement have arisen in the long-term results of the phase 1/2 trials. At UPenn/UF, three patients who had the largest improvement of regional retinal sensitivity at 1-3 years also had subsequent contraction of retinal sensitivity at 5-6 years post-treatment.36
A similar trend was noted at the three-year follow-up in all patients treated at UCL.27 The highly anticipated phase 3 results from CHOP are still pending publication, though a recent press release reported improved bilateral mobility testing after one year post-treatment.37
The success of these three pivotal clinical trials in RPE65-LCA have paved the way for the development of retinal gene replacement phase 1/2 clinical trials for other autosomal recessive IRDs (Stargardt dystrophy, Usher syndrome, achromatopsia) and X-linked recessive IRDs (choroideremia, retinoschisis). Similar to LCA, gene delivery for choroideremia, X-linked retinoschisis (XLRS), and achromatopsia also utilize the most commonly-used vector, AAV. However, the ABCA4 and MYO7A genes associated with Stargardt dystrophy and Usher syndrome type 1B, respectively, exceed the 4.7 kb cassette size limit of the AAV vector.
Consequently, gene therapy for ABCA4-Stargardt dystrophy and MYO7A-Usher syndrome require the greater capacity of the equine infectious anemia virus (EIAV) vector. Nearly all retinal gene therapy requires vitreoretinal surgery to deliver the gene-encoding vector to the outer retina via a subretinal injection. The only exception is for XLRS, in which vector delivery to the macula and schisis areas is sufficient with an intravitreal injection.38,39
Results of early findings from these ongoing studies have yet to be published. However, the choroideremia gene therapy clinical trials originated from the United Kingdom, where studies sponsored by the University of Oxford have already demonstrated improved retinal sensitivity in five of six patients and improved visual acuity in patients with the worst baseline.40
While clinical trials for retinal gene replacement in recessive IRD are expanding, promising techniques for the correction and suppression of genetic mutations in autosomal dominant IRD (e.g. RNA-interference, zinc finger nucleases, TALENs, and CRISPR/Cas9) continue to be developed and will undoubtedly be brought to clinical trials in the very near future.41-44
STRATEGY #3: REVIVING VISUAL FUNCTION
Reviving visual function in patients with IRD will require the replacement of lost retinal tissue or the artificial substitution of photoreceptor function. These strategies generally apply to patients with more advanced disease and vision loss, who are less likely to benefit from neuroprotection or gene therapy.
The ultimate goal of stem cell research is the ability to generate stable differentiated viable tissues, which can be anatomically and functionally reintegrated back into the body. This is distinct from other stem cell-based research aimed at delivering neutrophic factors to slow retinal degeneration (see Strategy #1). With regard to the revival of visual function in IRD, the current focus of stem cell therapy has been to deliver stem cell-derived RPE cells back into regions of atrophy.
There is currently one phase 1/2 clinical trial with the goal of implanting human embryonic stem cell-derived RPE cells into the subretinal space of patients with Stargardt dystrophy (NCT01345006). Published results so far have revealed anatomical changes in the implant area that are suggestive of RPE-like engraftment.45
Specifically, the treated retina showed increased pigmentation that correlated with a hyper-reflective layer along Bruch’s membrane on OCT, but these characteristics are currently of uncertain significance. Confirmation that the pigmented area truly represents viable and functional RPE cells will require further exploration.
Substitution for photoreceptor function is another way to revive visual function in patients with advanced disease. The two methodologies currently in clinical trials are optogenetics and retinal prosthetics.
Optogenetics utilizes gene therapy techniques to induce retinal bipolar or ganglion cells to express light-sensitive opsins with the goal of replacing the visual function of the degenerated photoreceptors.46,47 For patients with RP, there is currently a phase 1/2 clinical trial for optogenetics in which the gene for channelrhodopsin-2, a cation channel derived from the green alga Chlamydomonas reinhardtii, is delivered within an AAV vector via intravitreal injection (Table 2).
Retinal prosthetics are devices that substitute for degenerated photoreceptor function by electrically stimulating the visual pathway at the level of the residual inner retina. The Argus II (Second Sight, Sylmar, CA) system consists of an epiretinal 60-electrode array, which stimulates the retina using visual information fed from a glass-mounted camera and video processing unit.
The result of this electrical stimulation of the retina translates to the perception of patterned phosphenes, representing contextual information for navigation. Indeed, ongoing phase 2 studies in these patients have shown safety,48 improved mobility,49 and spatial motor skills.50 This is the first retinal prosthetic that has been FDA-approved for humanitarian use in patients with RP and bare light perception in the worst-seeing eye.
TREATMENT OF CYSTOID MACULAR EDEMA IN IRD
Chronic cystoid macular edema (CME) can be relatively common in RP (~11-49% of cases),51,52 as well as Usher syndrome. The pathophysiology of CME in IRD is still unknown, but it is thought to be distinct from other common causes of CME such as that due to diabetes, uveitis, or neovascularization.
While there is no gold standard for treatment, retrospective case series have shown that topical or oral carbonic anhydrase inhibitors and intravitreal corticosteroids can reduce CME in RP (Table 1).53-56
The use of intravitreal anti-vascular endothelial growth factor (VEGF) agents may also reduce CME in RP.57 However, its widespread use is tempered by the fact that VEGF levels are already lower than normal in the eyes of patients with RP.58
Moreover, there are unknown long term consequences of anti-VEGF in IRDs pertaining to vascular attenuation and retinal atrophy. That being said, the side effects of the aforementioned medications must constantly be weighed against the benefits of treatment, which are often limited by tachyphylaxis and the lack of correlation with visual function improvement.53,59
There is a need for additional therapeutic options for CME in IRD, and the following agents are currently in clinical trials: topical interferon gamma-1b (NCT02338973), oral minocycline (NCT02140164), and intravitreal aflibercept (NCT02661711).
SUMMARY
Inherited retinal degenerations are a heterogeneous group of disorders in which accurate diagnosis can be inherently challenging due to the relatively low prevalence, high variability of presentation, and need for specialized visual diagnostic equipment.
Even though the technology for genetic testing has advanced greatly over the past years, a high degree of clinical suspicion and pretest probability is still vital for guiding genetic testing and interpreting the results.
The early diagnosis and genetic confirmation of IRD is not only important so patients may be offered a choice of participation in clinical trials, but any potential treatment will likely yield maximum benefits during the early phase of the disease.
As the era of targeted therapies becomes a reality, the goal of arresting the disease process and restoring retina function will ultimately require combinations of all strategies tailored to each patient with IRD. RP
REFERENCES
1. Hartong DT, Berson EL, Dryja TP. Retinitis pigmentosa. Lancet. 2006;368:1795-1809.
2. Consugar MB, Navarro-Gomez D, Place EM, et al. Panel-based genetic diagnostic testing for inherited eye diseases is highly accurate and reproducible, and more sensitive for variant detection, than exome sequencing. Genet Med. 2015;17:253-261.
3. Zhao L, Wang F, Wang H, et al. Next-generation sequencing-based molecular diagnosis of 82 retinitis pigmentosa probands from Northern Ireland. Hum Genet. 2015;134:217-230.
4. Chiang JP, Lamey T, McLaren T, et al. Progress and prospects of next-generation sequencing testing for inherited retinal dystrophy. Expert Rev Mol Diagn. 2015;15:1269-1275.
5. Perusek L, Maeda T. Vitamin A derivatives as treatment options for retinal degenerative diseases. Nutrients. 2013;5:2646-2666.
6. Koenekoop RK, Sui R, Sallum J, et al. Oral 9-cis retinoid for childhood blindness due to Leber congenital amaurosis caused by RPE65 or LRAT mutations: an open-label phase 1b trial. Lancet. 2014;384:1513-1520.
7. Allikmets R, Singh N, Sun H, et al. A photoreceptor cell-specific ATP-binding transporter gene (ABCR) is mutated in recessive Stargardt macular dystrophy. Nat Genet. 1997;15:236-246.
8. Cremers FP, van de Pol DJ, van Driel M, et al. Autosomal recessive retinitis pigmentosa and cone-rod dystrophy caused by splice site mutations in the Stargardt’s disease gene ABCR. Hum Mol Genet. 1998;7:355-362.
9. Martinez-Mir A, Paloma E, Allikmets R, et al. Retinitis pigmentosa caused by a homozygous mutation in the Stargardt disease gene ABCR. Nat Genet. 1998;18:11-12.
10. Weng J, Mata NL, Azarian SM, et al. Insights into the function of Rim protein in photoreceptors and etiology of Stargardt’s disease from the phenotype in abcr knockout mice. Cell. 1999;98:13-23.
11. Berson EL, Rosner B, Sandberg MA, et al. A randomized trial of vitamin A and vitamin E supplementation for retinitis pigmentosa. Arch Ophthalmol. 1993;111:761-772.
12. Massof RW, Fishman GA. How strong is the evidence that nutritional supplements slow the progression of retinitis pigmentosa? Arch Ophthalmol. 2010;128:493-495.
13. Aleman TS, Cideciyan AV, Windsor EA, et al. Macular pigment and lutein supplementation in ABCA4-associated retinal degenerations. Invest Ophthalmol Vis Sci. 2007;48:1319-1329.
14. Hoffman DR, Birch DG. Docosahexaenoic acid in red blood cells of patients with X-linked retinitis pigmentosa. Invest Ophthalmol Vis Sci. 1995;36:1009-1018.
15. Krinsky NI, Landrum JT, Bone RA. Biologic mechanisms of the protective role of lutein and zeaxanthin in the eye. Annu Rev Nutr. 2003;23:171-201.
16. Bahrami H, Melia M, Dagnelie G. Lutein supplementation in retinitis pigmentosa: PC-based vision assessment in a randomized double-masked placebo-controlled clinical trial [NCT00029289]. BMC Ophthalmol. 2006;6:23.
17. Berson EL, Rosner B, Sandberg MA, et al. Clinical trial of lutein in patients with retinitis pigmentosa receiving vitamin A. Arch Ophthalmol. 2010;128:403-411.
18. Hoffman DR, Hughbanks-Wheaton DK, Pearson NS, et al. Four-year placebo-controlled trial of docosahexaenoic acid in X-linked retinitis pigmentosa (DHAX trial): a randomized clinical trial. JAMA Ophthalmol. 2014;132:866-873.
19. Hoffman DR, Hughbanks-Wheaton DK, Spencer R, et al. Docosahexaenoic acid slows visual field progression in X-linked retinitis pigmentosa: ancillary outcomes of the DHAX Trial. Invest Ophthalmol Vis Sci. 2015;56:6646-6653.
20. Noorwez SM, Ostrov DA, McDowell JH, et al. A high-throughput screening method for small-molecule pharmacologic chaperones of misfolded rhodopsin. Invest Ophthalmol Vis Sci. 2008;49:3224-3230.
21. Clemson CM, Tzekov R, Krebs M, et al. Therapeutic potential of valproic acid for retinitis pigmentosa. Br J Ophthalmol. 2011;95:89-93.
22. Bhalla S, Joshi D, Bhullar S, et al. Long-term follow-up for efficacy and safety of treatment of retinitis pigmentosa with valproic acid. Br J Ophthalmol. 2013;97:895-899.
23. Sieving PA, Caruso RC, Tao W, et al. Ciliary neurotrophic factor (CNTF) for human retinal degeneration: phase I trial of CNTF delivered by encapsulated cell intraocular implants. Proc Natl Acad Sci U S A. 2006;103:3896-3901.
24. Talcott KE, Ratnam K, Sundquist SM, et al. Longitudinal study of cone photoreceptors during retinal degeneration and in response to ciliary neurotrophic factor treatment. Invest Ophthalmol Vis Sci. 2011;52:2219-2226.
25. Zein WM, Jeffrey BG, Wiley HE, et al. CNGB3-achromatopsia clinical trial with CNTF: diminished rod pathway responses with no evidence of improvement in cone function. Invest Ophthalmol Vis Sci. 2014;55:6301-6308.
26. Byrne LC, Dalkara D, Luna G, et al. Viral-mediated RdCVF and RdCVFL expression protects cone and rod photoreceptors in retinal degeneration. J Clin Invest. 2015;125:105-116.
27. Bainbridge JW, Smith AJ, Barker SS, et al. Effect of gene therapy on visual function in Leber’s congenital amaurosis. N Engl J Med. 2008;358:2231-2239.
28. Maguire AM, Simonelli F, Pierce EA, et al. Safety and efficacy of gene transfer for Leber’s congenital amaurosis. N Engl J Med. 2008;358:2240-2248.
29. Hauswirth WW, Aleman TS, Kaushal S, et al. Treatment of leber congenital amaurosis due to RPE65 mutations by ocular subretinal injection of adeno-associated virus gene vector: short-term results of a phase I trial. Hum Gene Ther. 2008;19:979-990.
30. Maguire AM, High KA, Auricchio A, et al. Age-dependent effects of RPE65 gene therapy for Leber’s congenital amaurosis: a phase 1 dose-escalation trial. Lancet. 2009;374:1597-1605.
31. Jacobson SG, Cideciyan AV, Ratnakaram R, et al. Gene therapy for leber congenital amaurosis caused by RPE65 mutations: safety and efficacy in 15 children and adults followed up to 3 years. Arch Ophthalmol. 2012;130:9-24.
32. Testa F, Maguire AM, Rossi S, et al. Three-year follow-up after unilateral subretinal delivery of adeno-associated virus in patients with Leber congenital Amaurosis type 2. Ophthalmology. 2013;120:1283-1291.
33. Cideciyan AV, Aguirre GK, Jacobson SG, et al. Pseudo-fovea formation after gene therapy for RPE65-LCA. Invest Ophthalmol Vis Sci. 2015;56:526-537.
34. Boye SE, Boye SL, Lewin AS, et al. A comprehensive review of retinal gene therapy. Mol Ther. 2013;21:509-519.
35. Bennett J, Ashtari M, Wellman J, et al. AAV2 gene therapy readministration in three adults with congenital blindness. Sci Transl Med. 2012;4:120ra15.
36. Jacobson SG, Cideciyan AV, Roman AJ, et al. Improvement and decline in vision with gene therapy in childhood blindness. N Engl J Med. 2015;372:1920-1926.
37. Spark Therapeutics Announces Positive Top-line Results From Pivotal Phase 3 Trial of SPK-RPE65 for Genetic Blinding Conditions [press release]. Philadelphia, PA; 2015.
38. Molday RS, Kellner U, Weber BH. X-linked juvenile retinoschisis: clinical diagnosis, genetic analysis, and molecular mechanisms. Prog Retin Eye Res. 2012;31:195-212.
39. Park TK, Wu Z, Kjellstrom S, et al. Intravitreal delivery of AAV8 retinoschisin results in cell type-specific gene expression and retinal rescue in the Rs1-KO mouse. Gene Ther. 2009;16:916-926.
40. MacLaren RE, Groppe M, Barnard AR, et al. Retinal gene therapy in patients with choroideremia: initial findings from a phase 1/2 clinical trial. Lancet. 2014;383:1129-1137.
41. Millington-Ward S, Chadderton N, O’Reilly M, et al. Suppression and replacement gene therapy for autosomal dominant disease in a murine model of dominant retinitis pigmentosa. Mol Ther. 2011;19:642-649.
42. Miller JC, Holmes MC, Wang J, et al. An improved zinc-finger nuclease architecture for highly specific genome editing. Nat Biotechnol. 2007;25:778-785.
43. Christian M, Cermak T, Doyle EL, et al. Targeting DNA double-strand breaks with TAL effector nucleases. Genetics. 2010;186:757-761.
44. Ran FA, Hsu PD, Wright J, et al. Genome engineering using the CRISPR-Cas9 system. Nat Protoc. 2013;8:2281-2308.
45. Schwartz SD, Regillo CD, Lam BL, et al. Human embryonic stem cell-derived retinal pigment epithelium in patients with age-related macular degeneration and Stargardt’s macular dystrophy: follow-up of two open-label phase 1/2 studies. Lancet. 2015;385:509-516.
46. Bi A, Cui J, Ma YP, et al. Ectopic expression of a microbial-type rhodopsin restores visual responses in mice with photoreceptor degeneration. Neuron. 2006;50:23-33.
47. Thyagarajan S, van Wyk M, Lehmann K, et al. Visual function in mice with photoreceptor degeneration and transgenic expression of channelrhodopsin 2 in ganglion cells. J Neurosci. 2010;30:8745-8758.
48. Ho AC, Humayun MS, Dorn JD, et al. Long-Term results from an epiretinal prosthesis to restore sight to the blind. Ophthalmology. 2015;122:1547-1554.
49. Humayun MS, Dorn JD, da Cruz L, et al. Interim results from the international trial of Second Sight’s visual prosthesis. Ophthalmology. 2012;119:779-788.
50. Ahuja AK, Dorn JD, Caspi A, et al. Blind subjects implanted with the Argus II retinal prosthesis are able to improve performance in a spatial-motor task. Br J Ophthalmol. 2011;95:539-543.
51. Pruett RC. Retinitis pigmentosa: clinical observations and correlations. Trans Am Ophthalmol Soc. 1983;81:693-735.
52. Adackapara CA, Sunness JS, Dibernardo CW, et al. Prevalence of cystoid macular edema and stability in oct retinal thickness in eyes with retinitis pigmentosa during a 48-week lutein trial. Retina. 2008;28:103-110.
53. Genead MA, Fishman GA. Efficacy of sustained topical dorzolamide therapy for cystic macular lesions in patients with retinitis pigmentosa and usher syndrome. Arch Ophthalmol. 2010;128:1146-1150.
54. Liew G, Moore AT, Webster AR, et al. Efficacy and prognostic factors of response to carbonic anhydrase inhibitors in management of cystoid macular edema in retinitis pigmentosa. Invest Ophthalmol Vis Sci. 2015;56:1531-1536.
55. Thobani A, Fishman GA. The use of carbonic anhydrase inhibitors in the retreatment of cystic macular lesions in retinitis pigmentosa and X-linked retinoschisis. Retina. 2011;31:312-315.
56. Scorolli L, Morara M, Meduri A, et al. Treatment of cystoid macular edema in retinitis pigmentosa with intravitreal triamcinolone. Arch Ophthalmol. 2007;125:759-764.
57. Yuzbasioglu E, Artunay O, Rasier R, et al. Intravitreal bevacizumab (Avastin) injection in retinitis pigmentosa. Curr Eye Res. 2009;34:231-237.
58. Salom D, Diaz-Llopis M, Garcia-Delpech S, et al. Aqueous humor levels of vascular endothelial growth factor in retinitis pigmentosa. Invest Ophthalmol Vis Sci. 2008;49:3499-3502.
59. Apushkin MA, Fishman GA, Grover S, et al. Rebound of cystoid macular edema with continued use of acetazolamide in patients with retinitis pigmentosa. Retina. 2007;27:1112-1118.
60. Querques G, Benlian P, Chanu B, et al. DHA supplementation for late onset Stargardt disease: NAT-3 study. Clin Ophthalmol. 2010;4:575-580.
61. Ku CA, Hariprasad SM, Pennesi ME. Gene therapy trial update: A primer for vitreoretinal specialists. Ophthalmic Surg Lasers Imaging Retina. 2016;47:6-12.