Heads-up Vitreoretinal Surgery: Emerging Technology in Surgical Visualization
The future of retinal surgery
YASHA S. MODI, MD • JUSTIS P. EHLERS, MD
The utilization of heads-up display (HUD) as a tool to improve efficiency and precision has a storied history dating back to the late 1940s and beginning with military aviation. Early attempts with HUD technology in aviation were designed to improve target visualization in the darkness for fighter pilots. The premise of the technology is to provide accessible, real-time information directly in the operator’s field of view, thus eliminating distracting and potentially compromising head-down or side movements.
This technology advanced tremendously in the 1970s and began providing fighter pilots information about speed, altitude, navigational information, and runway aim points. This new information afforded pilots greater situational awareness and accuracy in their maneuvers.
While the initial drawbacks of this technology included occasional “tunnel vision” as pilots initially fixated primarily on the HUD, the eventual learning curve outweighed the drawbacks of the technology. In the modern era, commercial airplanes and select automobiles utilize HUD to provide the operator accessible information that can be rapidly assimilated to aid in decision-making.
ADVANCES IN SURGERY
The application of HUD technology to complex or multifaceted surgical maneuvers seems intuitively beneficial, because the intended goal is to provide multiple data streams immediately to the surgeon. While maximizing the information available, limiting surgeon distraction and providing surgery-critical information will be vital.
Yasha S. Modi, MD, is a vitreoretinal surgery fellow at the Cole Eye Institute of the Cleveland Clinic and has no financial interest in products mentioned here. Justis P. Ehlers, MD is on the faculty of Cole and is a consultant for Zeiss and Alcon. He can be reached at ehlersj@ccf.org
Relative to the aviation/automobile industry, the application of HUD technology to medicine remains in its infancy, with a slow, albeit steady, implementation. Experiments incorporating HUD into cardiopulmonary bypass,1 diabetic limb-salvage surgery,2 diagnostic transesophageal echocardiography,3 and stereotactic neurosurgery4 have demonstrated feasibility with this technology, and HUD applications will likely continue to expand.
In ophthalmic surgery, a surgical strategy is often derived preoperatively and then carried forward in the operating room with limited direct availability of preoperative imaging within the surgical field. This process prompted the development of HUD incorporation into cataract surgery to allow for imported preoperative data to virtually overlay the surgical sight. The ability to include HUD information for cataract surgery has now become more commonplace in multiple microscope systems.
One such product is the Surgical Navigation System (Cirle, Miami, FL), which provides three-dimensional image guidance through the oculars of the surgical microscope. The intended goal is to improve accuracy in the following steps: placement of the corneal incision; maintaining capsulorhexis sizing and centration; placement of limbal relaxing incisions; and alignment of toric lenses.
Other commercial options include the Zeiss Cataract Suite with Callisto System (Carl Zeiss Meditec, Dublin, CA) and the Alcon (Fort Worth, TX) LuxOR Refractive Surgery suite with VERION image-guided system integration.
MOVING HUD AWAY FROM THE MICROSCOPE
Further development of HUD visualization has been directed toward obviating the need to look through standard microscope oculars. TrueVision 3D Surgical (Santa Barbara, CA) has developed a stereoscopic high-definition monitor to project the surgical field. This transformation to a total digital platform may enable increased flexibility for integrating additional data streams for surgeon feedback (Figure 1). Potential options for image integration include preoperative data and live intraoperative imaging.
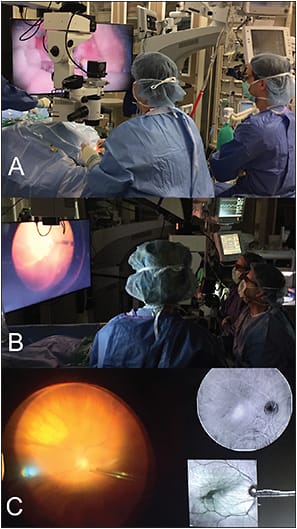
Figure 1. TrueVision 3D surgical visualization system. A, B) TrueVision system for heads-up vitreoretinal surgery utilizing an adapted Leica microscope. C) Example of multi-modality image display during real-time surgery utilizing the TrueVision system.
COURTESY OF TRUEVISION 3D SURGICAL AND THE AUTHOR
In its current configuration, the system requires the use of 3D glasses and a large, high-definition 4,000-pixel monitor to perform surgeries that allow for high-definition information to each eye. Additional system enhancements will include a 4,000-pixel camera to improve overall resolution and visualization.
Advantages of this technology include the potentially improved ergonomics of the surgical position, which may relieve tension on the neck and lower back, a 3D view with excellent depth perception, allowing for precise membrane peeling, and a digitally amplified camera signal that allows for visualization of the retina with lower-intensity light.
Although there are significant advantages, there are some challenges that must be considered. First, the image obtained from the microscope is projected to the monitor, thus necessitating the presence of both the microscope and the TrueVision system (both of which have large footprints) in the OR.
While the image obtained through the 3D glasses offers adequate depth perception to safely facilitate membrane peeling and complex surgical maneuvers, image quality continues to be refined to reproduce colors and stereo information maximally.
HUD INCORPORATION OF INTRAOPERATIVE IMAGING
Incorporation of imaging modalities into HUD to aid real-time surgical decision-making may prove to be a valuable addition that enhances surgical outcomes. The use of intraoperative optical coherence tomography (iOCT) has been previously described in the literature in surgical cases, including macular holes, epiretinal membranes, rhegmatogenous and tractional retinal detachments, vitreomacular traction, and retinopathy of prematurity.5-15
Microscope-integrated iOCT systems described in the literature include microscope add-ons that are placed within the optical path of the microscope (eg, the Duke and Cleveland Clinic prototypes), as well as systems built into the microscope footprint (eg, the Zeiss Rescan 700).
Microscope integration with HUD in the ocular viewing system is currently present in the Rescan 700 and the Haag-Streit (Mason, OH) iOCT system, as well as in multiple research systems (eg, the Duke and Cleveland Clinic prototypes) (Figure 2).
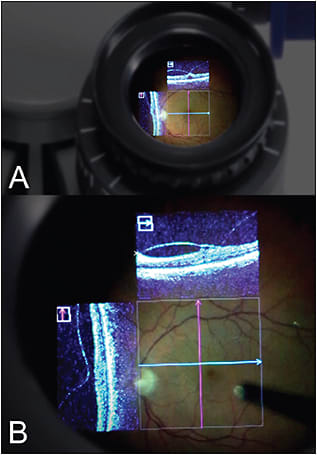
Figure 2. Heads-up OCT data within the microscope ocular on the Zeiss Rescan 700. A) View of microscope ocular. B) View within ocular showing en face microscope view with overlay of vertical and horizontal OCT scans. OCT aiming beam is noted in the middle with the horizontal and vertical crosshairs.
COURTESY OF CARL ZEISS MEDITEC AND THE AUTHOR
Seamless integration of iOCT data into HUD may provide significant advantages, including rapid scan localization, immediate surgeon feedback about residual membranes, and potentially, visualization of real-time tissue-instrument interaction.
The two-year results of one of the largest prospective studies evaluating the utility of iOCT were published last year in the American Journal of Ophthalmology.8 This study evaluated a total of 256 posterior-segment surgical cases. A surgeon feedback form was determined to assess the utility of this technology to the surgeon.
In 43% of cases (63/146 procedures), the use of iOCT enhanced surgeon understanding of the surgical anatomy, as well as surgical decision-making. Questions that remain to be answered include how iOCT will affect long-term surgical outcomes and how the outcomes compare to patients undergoing surgery without iOCT.
More recently, the DISCOVER study one-year results demonstrated the potential impact of microscope-integrated iOCT on surgeon decision-making in membrane peeling cases utilizing HUD surgeon feedback.16
THE FUTURE OF IMAGING TECHNOLOGIES INTO HUD
Further advancements in HUD technology will likely involve more seamless integration of HUD into novel surgical visualization systems, potentially obviating the need for the traditional surgical microscope in the OR. Increased integration will progressively decrease the footprint of the visualization system and allow for implementation, even in smaller ORs.
Additionally, as the technology improves, multimodal image integration, including iOCT, and potentially registered vascular imaging (eg, OCT angiography, fluorescein angiography overlays) may maximize surgeon information and enhance the individualized surgical care of patients.
Current research efforts are directed toward making iOCT surgical tools that may allow for real-time HUD OCT-guided surgical maneuvers. Special prototype surgical tools with unique materials to minimize light scattering are currently being investigated and tested.10 This technology may be highly beneficial in complex membrane cases, to accurately initiate dissection planes and to limit collateral damage.
Ultimately, the goals are to aid surgeon decision-making through better understanding of intraoperative tissue changes and to tailor our surgeries to the individual to maximize surgical outcomes.
CONCLUSIONS
Heads-up display in ophthalmic surgery and particularly in vitreoretinal surgery is slowly gaining traction as an alternative to the traditional approaches we currently employ. While there may be an initial learning curve, lessons from aviation, the automobile industry, and other surgical fields have suggested that the delay will not deter the adoption of this technology and instead could potentially enhance surgical capabilities. Additionally, image integration with iOCT may serve a valuable function to improve our intraoperative understanding of surgical cases. RP
REFERENCES
1. Austin JW. Evaluation of a “heads-up” display for cardiopulmonary bypass. J Extra Corpor Technol. 2000;32:49-53.
2. Armstrong DG, Rankin TM, Giovinco NA, et al. A heads-up display for diabetic limb salvage surgery: a view through the google looking glass. J Diabetes Sci Technol. 2014;8:951-956.
3. Platt MJ. Heads up display. Br J Anaesth. 2004;92:602-603.
4. Eljamel MS. Frameless stereotactic neurosurgery: two steps towards the Holy Grail of surgical navigation. Stereotact Funct Neurosurg. 1999;72:125-128.
5. Binder S, Falkner-Radler CI, Hauger C, et al. Feasibility of intrasurgical spectral-domain optical coherence tomography. Retina. 2011;31:1332-1336.
6. Chavala SH, Farsiu S, Maldonado R, et al. Insights into advanced retinopathy of prematurity using handheld spectral domain optical coherence tomography imaging. Ophthalmology 2009;116:2448-2456.
7. Dayani PN, Maldonado R, Farsiu S, Toth CA. Intraoperative use of handheld spectral domain optical coherence tomography imaging in macular surgery. Retina. 2009;29:1457-1468.
8. Ehlers JP, Dupps WJ, Kaiser PK, et al. The Prospective Intraoperative and Perioperative Ophthalmic ImagiNg with Optical CoherEncE TomogRaphy (PIONEER) Study: 2-year results. Am J Ophthalmol. 2014;158:999-1007.
9. Ehlers JP, Ohr MP, Kaiser PK, Srivastava SK. Novel microarchitectural dynamics in rhegmatogenous retinal detachments identified with intraoperative optical coherence tomography. Retina. 2013;33:1428-1434.
10. Ehlers JP, Srivastava SK, Feiler D, et al. Integrative advances for OCT-guided ophthalmic surgery and intraoperative OCT: microscope integration, surgical instrumentation, and heads-up display surgeon feedback. PLoS One. 2014;9:e105224.
11. Ehlers JP, Tam T, Kaiser PK, et al. Utility of intraoperative optical coherence tomography during vitrectomy surgery for vitreomacular traction syndrome. Retina. 2014;34:1341-1346.
12. Ehlers JP, Tao YK, Srivastava SK. The value of intraoperative optical coherence tomography imaging in vitreoretinal surgery. Curr Opin Ophthalmol. 2014;25:221-227.
13. Ehlers JP, Xu D, Kaiser PK, et al. Intrasurgical dynamics of macular hole surgery: an assessment of surgery-induced ultrastructural alterations with intraoperative optical coherence tomography. Retina. 2014;34:213-221.
14. Ray R, Baranano DE, Fortun JA, et al. Intraoperative microscope-mounted spectral domain optical coherence tomography for evaluation of retinal anatomy during macular surgery. Ophthalmology. 2011;118:2212-2217.
15. Tao YK, Srivastava SK, Ehlers JP. Microscope-integrated intraoperative OCT with electrically tunable focus and heads-up display for imaging of ophthalmic surgical maneuvers. Biomed Opt Express. 2014;5:1877-1885.
16. Ehlers JP, Goshe J, Dupps WJ, et al. Determination of feasibility and utility of microscope-integrated optical coherence tomography during ophthalmic surgery: The DISCOVER study RESCAN results. JAMA Ophthalmol. 2015;133:1124-1132.