The Third Dimension: Advantages of 3D-OCT in Retina
Unprecedented detail of perfusion and other structures
ELAD MOISSEIEV, MD • SUSANNA PARK, MD, PhD • GLENN YIU, MD, PhD JOHN S. WERNER, PhD • ROBERT J. ZAWADZKI, PhD
Optical coherence tomography is currently the most rapidly growing retinal imaging modality in ophthalmology. By measuring the echo time delay and magnitude of back-scattered or back-reflected light, OCT technology can almost instantaneously generate cross-sectional images with an axial resolution of up to 2 µm for research-grade instruments. These cross-sectional B-scan images of the retina are analogous to histologic sections and serve as “optical biopsies” of their target tissues.1,2
The first in vivo cross-sectional OCT images of the retina in human patients were reported in 1993 using an early-generation, time-domain OCT instrument.3,4 With the advent of the newer-generation spectral-domain (also called Fourier-domain) OCT instruments,5-7 rapid high-resolution image stacks across the macula could be obtained, allowing for three-dimensional reconstruction of the entire macula.8-10
With the current commercial SD-OCT instruments, high-resolution 3D-OCT imaging of the retina can be performed easily in routine clinical practice, and it has become an integral part of the diagnosis and management of patients with retinal diseases.2,11
This article reviews the utility of structural 3D-OCT imaging of the retina in the evaluation of patients with retinal or choroidal disorders. In addition, with functional 3D-OCT imaging, it is also possible to study retinal and choroidal perfusion in detail not possible with two-dimensional angiography.
Elad Moisseiev, MD, is a fellow, and Susanna Park, MD, PhD, Glenn Yiu, MD, PhD, John S. Werner, PhD, and Robert J. Zawadzki, PhD, serve on the faculty of the Department of Ophthalmology & Vision Science, University of California Davis Eye Center in Sacramento. None of the authors reports any financial interests in any products mentioned in this article. Dr. Zawadzki can be reached via e-mail at rjzawadzki@ucdavis.edu.
BASICS OF OCT IMAGING
The transformation of acquired OCT data into the images used by clinicians involves multiple steps of signal recognition, processing, and averaging. Commercial SD-OCT instruments are equipped with various image processing algorithms so that desired images are readily available for viewing by clinicians.
The various OCT imaging options for the retina are as follows (with some examples shown in Figure 1).
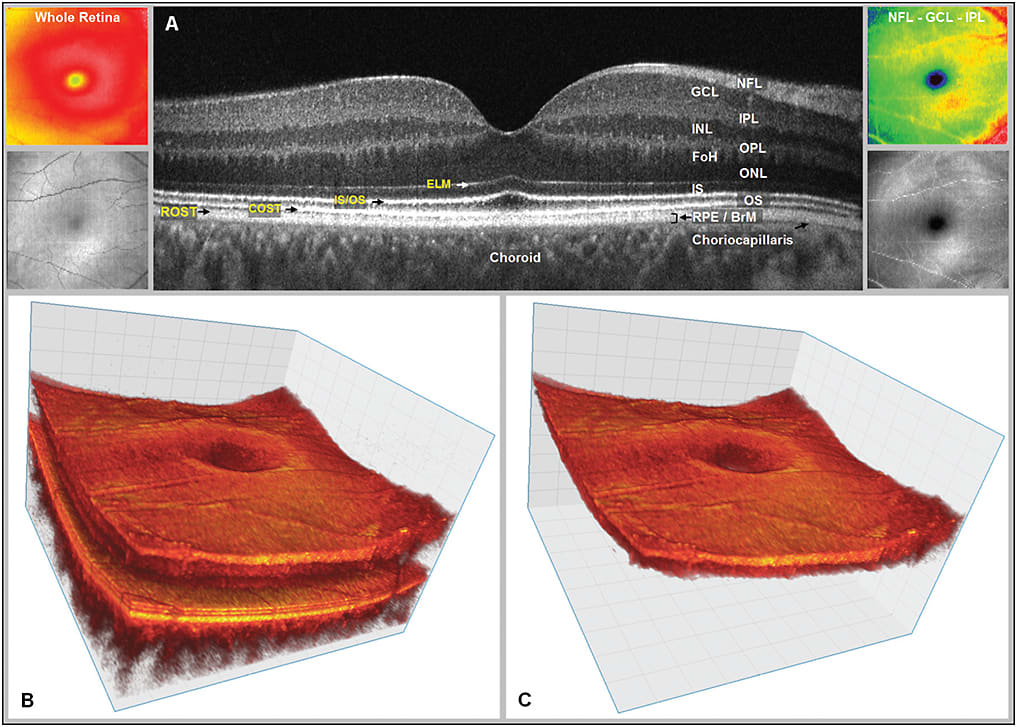
Figure 1. Visualization examples of the same 3D OCT data set. A) Fourier-domain optical coherence tomography (FD-OCT) B-scan of the fovea from a normal 34-year-old volunteer acquired with research grade FD-OCT instrument scanning 5 mm laterally. Abbreviations: NFL - nerve fiber layer; GCL- ganglion cell layer; IPL - inner plexiform layer; INL - inner nuclear layer; OPL - outer plexiform layer; FoH - fibers of Henle; ONL - outer nuclear layer; IS - inner segment; OS - outer segment layer; RPE - retinal pigment epithelium, BrM – Bruch’s membrane. The external limiting membrane (ELM), inner/outer segment junction (IS/OS), cone photoreceptor outer segment tips (COST) and rod photoreceptor outer segment tips (ROST) and choriocapillaris may also be seen. ROST and RPE appear as one layer in the fovea but are visible as separate layers in the periphery. The fibers of Henle cannot be clearly distinguished from the ONL in this image. The Bruch’s membrane cannot be separated from RPE on this image, but have been confirmed using the same OCT system for imaging retinal dystrophies. B) Volumetric reconstruction of central foveal region from 100 B-scans from the same volunteer in (A). Small insets on the left show total retina thickness map (top) and OCT fundus view (en face (C-scan) projection) for this data set (bottom). C) Volumetric reconstruction of segmented three-layer inner retinal complex extracted from volume (B). Small insets on the right show three-layer complex thickness map (top) and OCT fundus view (en face (C-scan) projection) for three-layer complex only (bottom).
IMAGES COURTESY OF NATURE PUBLISHING GROUP
• B-scan: A typical 2D cross-sectional image of the retina provides visualization of the various retinal layers. With commercial SD-OCT instruments, the highest-resolution B-scan image can be obtained using the single line-scan option. The B-scan can be horizontal, vertical, or oriented at any angle.
• 3D imaging: The entire macula is reconstructed using serial horizontal (or vertical) B-scan images, typically separated by 15 to 60 µm. The resulting 3D image is sometimes referred to as a “cube.” Once the retina is imaged in three dimensions, it can be analyzed from different angles to better characterize the abnormality, and volumetric measurements can be obtained.
• C-scan: A 2D image can be reconstructed from the 3D-OCT data to provide “en face” visualization of a specific retinal layer in the scanned area.
• Enhanced depth imaging (EDI): By changing the focus depth of the OCT instrument, improved visualization of deeper tissues, such as the choroidal vasculature and the suprachoroidal space, may be achieved.12-14
• OCT angiography (OCTA): Using new software and methods of data processing, such as split spectrum amplitude decorrelation (SSADA) and phase variance, noninvasive imaging of the retinal and choroidal blood flow is also possible using SD-OCT.15-21 The data are acquired as a volume and can be visualized in C-scan mode.
Three-dimensional OCT offers several advantages over standard 2D imaging. By incorporating a third dimension, 3D-OCT provides more information regarding the structure and the relative position and orientation of pathologic lesions. This additional information improves our diagnosis and understanding of the pathogenesis of various retinal conditions.22
Additionally, 3D-OCT imaging of the retina enables volume measurement in areas of interest within the retina. Volume measurements allow for more accurate quantitative measurement of pathologic changes in the retina, which can be used as biomarkers or outcome measurements for certain types of therapy.
CLINICAL APPLICATIONS
Reconstruction by 3D-OCT of a detailed structural map in patients with vitreoretinal interface disorders, choroidal neovascularization, macular edema, and other conditions has been shown to provide additional information that is not achievable from standard 2D cross-sectional B-scan OCT images.9,23
Improved imaging is obviously a diagnostic advantage and may also contribute to clinical decision-making. For example, Figure 2 shows 3D visualization of a patient with retinal angiomatous proliferation.
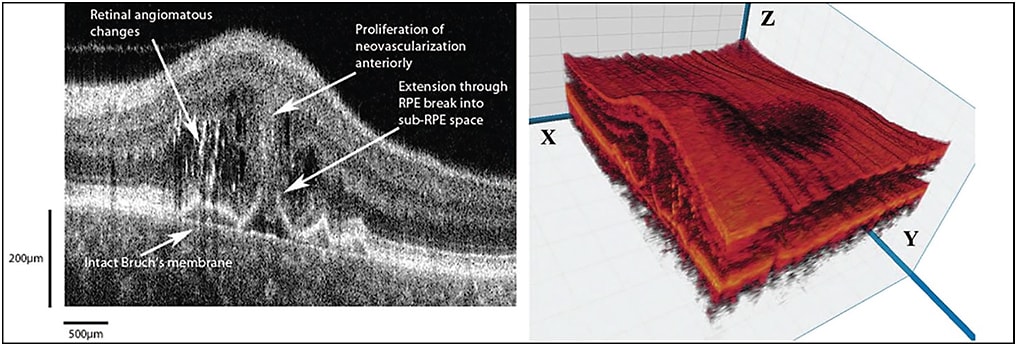
Figure 2. Visualization of the retina of a patient with RAP. Left: shows FD-OCT B-scan shows the extension from the intraretinal neovascularization through a break in the RPE to an early lesion in the sub-RPE space. Note that Bruch’s membrane is still intact. Right: 3D reconstruction of the macula with cut through the RAP complex. The B-scan is taken from the x-z plane.
IMAGES COURTESY OF WOLTERS KLUWER
Exudative AMD
Using 3D-OCT and C-scan, a close correlation was noted between the size and location of the subretinal hyper-reflective lesion seen on OCT and CNV membrane imaged using fluorescein angiography.25
In addition, using phase-variance OCTA, blood flow within a hyper-reflective subretinal lesion could be imaged in eyes with active untreated exudative age-related macular degeneration.26 Thus, using OCT and OCTA, the size and location of CNV membranes associated with AMD or other macular disorders may be determined noninvasively at diagnosis and following therapy.
In addition, associated changes within the retina can be visualized in 3D, including pigment epithelial detachment (PED), subretinal fibrosis, subretinal fluid (SRF), and macular edema.9,27,28
Volumetric quantification of intraretinal, subretinal, and sub–retinal pigment epithelium fluid by 3D-OCT in patients with exudative AMD following therapy may be more accurate measures of response to therapy than macular thickness measurements.29
Accurate monitoring of response to treatment is important in determining treatment efficacy and retreatment strategies.16 3D reconstruction of OCTA data has also demonstrated decreases in blood flow within CNV membranes in eyes with exudative AMD after anti-VEGF therapy.30
This new imaging technique may provide an alternative, possibly superior, method of monitoring treatment response in eyes with exudative AMD. By following blood flow changes within the CNV membrane using OCTA, impending exudation recurrence may be detected earlier, leading to earlier intervention and improved preservation of vision.31,32
Nonexudative AMD
3D-OCT has been used in eyes with dry AMD to quantify the volume of drusen, which may be used as a measurement of disease progression. Figure 3 shows 3D visualization of a drusen from a patient with AMD.
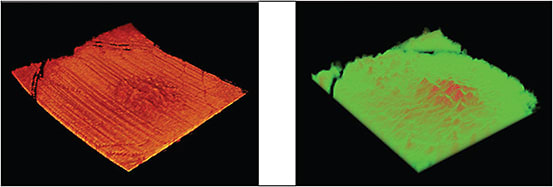
Figure 3. Left: 3D visualization of the retina of a patient with AMD showing only drusen after segmentation of the RPE and photoreceptor layers; right: 3D representation of the thickness map of the structure shown in the left image.
IMAGES COURTESY OF SPIE
In addition, the progression of nonexudative AMD, leading to macular thinning and atrophy, can be quantified by measuring changes in macular volume.34-36 Volume measurements of the RPE and drusen complex were found to predict two-year progression of intermediate AMD in AREDS2.37
By incorporating 3D-OCTA, the relative changes in choroidal circulation in AMD eyes can be visualized.38,39 In eyes with geography atrophy from nonexudative AMD, OCTA demonstrated inner choroidal ischemia. Figure 4 shows depth color-coded visualization of inner choroidal (choriocapillaris) ischemia in patients with AMD.
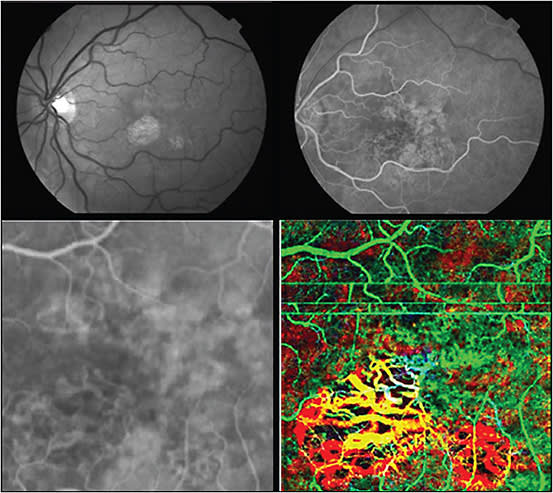
Figure 4. Dry AMD with geographic atrophy and focal loss of choriocapillaris shown using OCTA. Top left shows red free photo; top right is laminar flow phase of fluorescein angiography (FA). Lower left is high magnification FA image of foveal region; lower right shows a 3 x 3 mm PC-OCT and OCT angiography images, with depth color-coded image of same region. (Green is superficial/anterior choroidal region, which includes horizontal motion artifacts. Yellow is deeper into choroid, and red is the deepest imaging plane.) All slices are fixed distances relative to the anterior surface of retina. Depth color coded en face (C-scan) representation of volumetric data sets offers intuitive and easy to follow presentation of complex volumetric data.
IMAGES COURTESY OF ELSEVIER
By visualizing choroidal blood flow changes with OCTA, the relative contribution of choroidal ischemia to AMD progression can be determined. Such studies will improve our understanding of AMD pathogenesis and perhaps provide additional information regarding the risk of progression of AMD.
Macular Edema Associated With DME and RVO
Standard 2D B-scan OCT images are typically used in clinical practice to diagnose macular edema associated with diabetic retinopathy or retinal vein occlusion, as well as the need for treatment. It has been shown that 3D-OCT may demonstrate subtle folds of the internal limiting membrane in eyes with diabetic macular edema that are not seen with 2D imaging.
Such tractional changes are important to diagnose because traction can contribute to macular thickening seen on OCT and complicate the diagnosis and management of DME.40 Because traction can result in macular thickening unresponsive to medical therapy, 3D-OCT imaging would be helpful in evaluating such cases.
Macular thickness and volume are two OCT parameters that vary independently in patients with DME in response to treatment.41 It has been suggested that increased macular thickness and volume on OCT may be predictive of diabetic patients progressing to clinical DME42 and may be used to determine the frequency of monitoring patients.
In addition, cystoid macular volume and total macular volume have been shown to correlate better with visual acuity in eyes with DME than central macular thickness.43 Thus, macular volume measurements using 3D-OCT data may become the preferred OCT parameter to obtain and follow in the diagnosis and management of DME eyes.
Similarly, in retinal vein occlusion, total macular volume and cystoid macular volume have been shown to be correlated better with VA than central macular thickness in eyes with macular edema.43 Hence, 3D-OCT may be used for monitoring macular edema in eyes with RVO for response to treatment and progression of retinal ischemia.
Finally, 3D-OCT is also capable of demonstrating areas of retinal nonperfusion and the dynamic leakage of intraretinal fluids and exudation in patients with RVOs and diabetic retinopathy.44,45 Using 3D and C-scan OCTA, any macular ischemia can be analyzed in detail. Microvascular changes, such as microaneurysms, and areas of retinal nonperfusion can also be imaged.
Central Serous Chorioretinopathy
Three-dimensional OCT imaging has been shown to be useful for the diagnosis of central serous chorioretinopathy, including the localization of PEDs and sources of leakage.46-48 3D-OCT can quantify the height and greatest basal diameter and volume of SRF associated with various stages of CSC. It has been used to show that mean peak height of the SRF and the ratio between mean peak height and greatest basal diameter of SRF were significantly greater in patients with acute CSC than in those with chronic CSC.49
Thus, 3D-OCT may be a useful tool to distinguish chronic CSC cases that require intervention from more acute cases. In addition, 3D-OCT may also assist in directing laser treatment of extrafoveal lesions.
Vitreoretinal Interface Disorders
Another potential application of 3D-OCT imaging is in the preoperative planning of surgical intervention for vitreoretinal interface disorders, such as vitreomacular traction, macular hole (MH), and epiretinal membrane.
Three-dimensional OCT can provide a comprehensive view of the surface topography and anatomical configuration of VMT, with localization of the exact point of attachment of vitreous to retina, which may be useful when planning vitrectomy and relief of macular traction.50 The detailed analysis of the vitreomacular interface with 3D-OCT may allow for improved patient selection and monitoring of patients undergoing treatment for VMT and macular hole therapy, whether intravitreal ocriplasmin (Jetrea, ThromboGenics, Iselin, NJ) or vitrectomy is used.
In eyes with ERM or other tractional membranes, 3D-OCT imaging may also provide valuable preoperative information, such as the configuration and points of retinal attachment of the membrane, the presence of posterior vitreous detachment, and areas where membrane peeling could be initiated more easily.51-53
In addition, 3D-OCT allows for more detailed preoperative evaluation of parameters such as photoreceptor defects and cystic spaces, which may have clinically useful prognostic value in patients with ERM.54 3D-OCT has also been used to visualize inner retinal surface changes following ILM and macular hole surgery. These inner retinal defects were correlated with functional changes noted on microperimetry. Figure 5 shows 3D visualization of the inner retinal surface in a patient with a surgically closed macular hole.
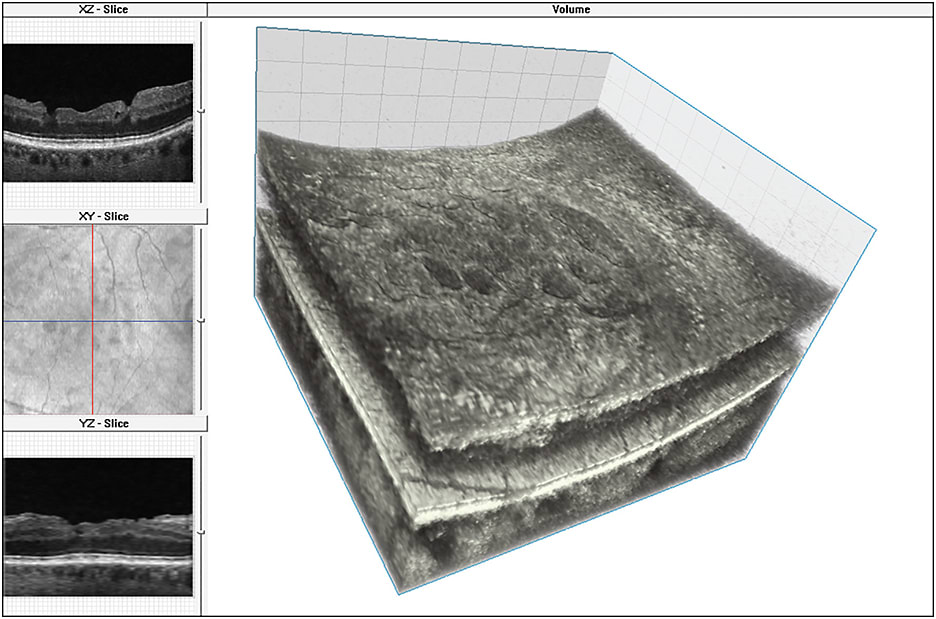
Figure 5. 3D FD-OCT image of an eye with surgically closed macular hole with ILM peeling as viewed on volume visualization and image processing software; top left: XZ plane (B-scan) shows macular surface irregularity, middle left: the en face projection view (XZ plane) of the volume showing the extent of the macular surface irregularity relative to the retinal vessels, bottom left: the “virtual” B-scan in YZ plane reconstructed from volume through section of macula with less surface irregularity and right: the 3D FD-OCT volume with surface irregularities.
IMAGES COURTESY OF WOLTERS KLUWER
Finally, 3D-OCTA has been used to measure total and inner macular volume, which were correlated with visual outcomes in eyes following macular hole surgery.55
CONCLUSION
Currently, the most commonly used OCT images in clinical decision-making are 2D cross-sectional images of the retina. However, most modern SD-OCT instruments can acquire data that can be used for 3D reconstruction and quantitative analysis.
The additional information provided with 3D-OCT imaging can improve the diagnosis and management of many common retinal conditions. As OCTA becomes commercially available, 3D and C-scan imaging mode will likely become the preferred modes for OCT image analysis.
REFERENCES
1. Huang D, Swanson EA, Lin CP, et al. Optical coherence tomography. Science. 1991;254:1178-1181.
2. Drexler W, Fujimoto JG. State-of-the-art retinal optical coherence tomography. Prog Retin Eye Res. 2008;27:45-88.
3. Fercher AF, Hitzenberger CK, Drexler W, Kamp G, Sattmann H. In-vivo optical coherence tomography. Am J Ophthalmol. 1993;116:113-115.
4. Swanson EA, Izatt JA, Hee MR, et al. In-vivo retinal imaging by optical coherence tomography. Optics Lett. 1993;18:1864-1866.
5. Leitgeb R, Hitzenberger CK, Fercher AF. Performance of fourier domain vs. time domain optical coherence tomography. Optics Express. 2003;11:889-894.
6. Choma MA, Sarunic MV, Yang C, Izatt JA. Sensitivity advantage of swept source and Fourier domain optical coherence tomography. Optics Express. 2003;11:2183-2189.
7. De Boer JF, Cense B, Park BH, Pierce MC, Tearney GJ, Bouma BE. Improved signal-to-noise ratio in spectral-domain compared with time-domain optical coherence tomography. Optics Lett. 2003;28:2067-2069.
8. Wojtkowski M, Srinivasan V, Fujimoto JG, et al. Three-dimensional retinal imaging with high-speed ultrahigh-resolution optical coherence tomography. Ophthalmology. 2005;112:1734-1746.
9. Alam S, Zawadzki RJ, Choi S, et al. Clinical application of rapid serial fourier-domain optical coherence tomography for macular imaging. Ophthalmology. 2006;113:1425-1431.
10. Srinivasan VJ, Wojtkowski M, Witkin AJ, et al. High-definition and 3-dimensional imaging of macular pathologies with high-speed ultrahigh-resolution optical coherence tomography. Ophthalmology. 2006;113:2054-2065.
11. Kostanyan T, Wollstein G, Schuman JS. New developments in optical coherence tomography. Curr Opin Ophthalmol. 2015;26:110-115.
12. Spaide RF, Koizumi H, Pozzoni MC. Enhanced depth imaging spectral-domain optical coherence tomography. Am J Ophthalmol. 2008;146:496-500.
13. Mrejen S, Spaide RF. Optical coherence tomography: Imaging of the choroid and beyond. Surv Ophthalmol. 2013;58:387-429.
14. Yiu G, Pecen P, Sarin N, et al. Characterization of the choroid-scleral junction and suprachoroidal layer in healthy individuals on enhanced-depth imaging optical coherence tomography. JAMA Ophthalmol. 2014;132:174-181.
15. Makita S, Hong Y, Yamanari M, Yatagai T, Yasuno Y. Optical coherence angiography. Optics Express. 2006;14:7821-7840.
16. Matsunaga D, Yi J, Puliafito CA, Kashani AH. OCT angiography in healthy human subjects. Ophthalmic Surg Lasers Imaging Retina. 2014;45:510-515.
17. Schwartz DM, Fingler J, Kim DY, et al. Phase-variance optical coherence tomography: a technique for noninvasive angiography. Ophthalmology. 2014;121:180-187.
18. Leitgeb RA, Szkulmowski M, Blatter C, Wojtkowski M. Doppler Fourier domain optical coherence tomography for label-free tissue angiography. In: Drexler W, Fujimoto JG, eds. Optical Coherence Tomography: Technology and Applications. New York, NY; Springer; 2015:1321-1352.
19. Reif R, Wang RK. Optical microangiography based on optical coherence tomography. In: Drexler W, Fujimoto JG, eds. Optical Coherence Tomography: Technology and Applications. New York, NY; Springer; 2015:1373-1397.
20. Tan O, Jia Y, Wei E, Huang D. Clinical applications of Doppler OCT and OCT angiography. In: Drexler W, Fujimoto JG, eds. Optical Coherence Tomography: Technology and Applications. New York, NY; Springer; 2015:1413-1428.
21. Gorczynska I, Migacz JV, Zawadzki RJ, Capps AG, Werner JS. Comparison of amplitude-decorrelation, speckle-variance and phase-variance OCT angiography methods for imaging the human retina and choroid. Biomed Optics Express. 2016;7:911-942.
22. Bodnar ZM, Akduman L. Three-dimensional OCT for the evaluation of chorioretinal diseases. Retin Physician. 2015;12(4):25-29.
23. Gerendas BS, Waldstein SM, Simader C, et al. Three-dimensional automated choroidal volume assessment on standard spectral-domain optical coherence tomography and correlation with the level of diabetic macular edema. Am J Ophthalmol. 2014;158:1039-1048.
24. Truong SN, Alam S, Zawadzki RJ, et al. High resolution Fourier-domain optical coherence tomography of retinal angiomatous proliferation. Retina. 2007;27:915-925.
25. Park SS, Truong SN, Zawadzki RJ, et al. High resolution Fourier-domain optical coherence tomographic imaging of choroidal neovascular membranes. Invest Ophthalmol Vis Sci. 2010;51:4200-4206.
26. Schwartz DM, Fingler J, Kim DY, et al. Phase-contrast optical coherence tomography: a new technique for non-invasive angiography. Ophthalmology. 2014;121:180-187.
27. Menke MN, Dabov S, Sturm V. Features of age-related macular degeneration assessed with three-dimensional Fourier-domain optical coherence tomography. Br J Ophthalmol. 2008;92:1492-1497.
28. de Bruin DM, Burnes DL, Loewenstein J, et al. In vivo three-dimensional imaging of neovascular age-related macular degeneration using optical frequency domain imaging at 1050 nm. Invest Ophthalmol Vis Sci. 2008;49:4545-4552.
29. Golbaz I, Ahlers C, Stock G, et al. Quantification of the therapeutic response of intraretinal, subretinal, and subpigment epithelial compartments in exudative AMD during anti-VEGF therapy. Invest Ophthalmol Vis Sci. 2011;52:1599-1605.
30. Nehemy MB, Brocchi DN, Veloso CE. Optical coherence tomography angiography imaging of quiescent choroidal neovascularization in age-related macular degeneration. Ophthalmic Surg Lasers Imaging Retina. 2015;46:1056-1057.
31. Huang D, Jia Y, Rispoli M, Tan O, Lumbroso B. Optical coherence tomography angiography of time course of choroidal neovascularization in response to anti-angiogenic treatment. Retina. 2015;35:2260-2264.
32. Kuehlewein L, Sadda SR, Sarraf D. OCT angiography and sequential quantitative analysis of type 2 neovascularization after ranibizumab therapy. Eye (Lond). 2015;29:932-935.
33. Zawadzki RJ, Fuller AR, Zhao M, et al. 3D OCT imaging in clinical settings: toward quantitative measurements of retinal structures. Proc SPIE. 2006;6138:1-11.
34. Ueda-Arakawa N, Ooto S, Ellabban AA, et al. Macular choroidal thickness and volume of eyes with reticular pseudodrusen using swept-source optical coherence tomography. Am J Ophthalmol. 2014;157:994-1004.
35. Iwama D, Hangai M, Ooto S, et al. Automated assessment of drusen using three-dimensional spectral-domain optical coherence tomography. Invest Ophthalmol Vis Sci. 2012;53:1576-1583.
36. Steinberg JS, Göbel AP, Fleckenstein M, Holz FG, Schmitz-Valckenberg S. Reticular drusen in eyes with high-risk characteristics for progression to late-stage age-related macular degeneration. Br J Ophthalmol. 2015;99:1289-1294.
37. Folgar FA, Yuan EL, Sevilla MB, et al; Age Related Eye Disease Study 2 Ancillary Spectral-Domain Optical Coherence Tomography Study Group. Drusen volume and retinal pigment epithelium abnormal thinning volume predict 2-year progression of age-related macular degeneration. Ophthalmology. 2016;123:39-50.
38. Kim DY, Fingler J, Zawadzki RJ, et al. Optical imaging of the chorioretinal vasculature in the living human eye. Proc Natl Acad Sci U S A. 2013;110:14354-14359.
39. Ferrara D, Waheed NK, Duker JS. Investigating the choriocapillaris and choroidal vasculature with new optical coherence tomography technologies. Prog Retin Eye Res. 2015 Oct 23. [Epub ahead of print]
40. Abe S, Yamamoto T, Kashiwagi Y, Kirii E, Goto S, Yamashita H. Three-dimensional imaging of the inner limiting membrane folding on the vitreomacular interface in diabetic macular edema. Jpn J Ophthalmol. 2013;57:553-562.
41. Lima-Gómez V, Blanco-Hernández DM. Disparity between foveal thickness and macular volume in diabetic macular edema. Cir Cir. 2012;80:25-30.
42. Sasaki M, Kawashima M, Kawasaki R, et al. Association of serum lipids with macular thickness and volume in type 2 diabetes without diabetic macular edema. Invest Ophthalmol Vis Sci. 2014;55:1749-1753.
43. Mimouni M, Nahum Y, Levant A, Levant B, Weinberger D. Cystoid macular edema: a correlation between macular volumetric parameters and visual acuity. Can J Ophthalmol. 2014;49:183-187.
44. Schmidt-Erfurth UM, Stock G, Pruente C, Ahlers C. Three-dimensional angiographic imaging of leakage in branch retinal vein occlusion. Acta Ophthalmol. 2010;88:181-187.
45. Kuehlewein L, An L, Durbin MK, Sadda SR. Imaging areas of retinal nonperfusion in ischemic branch retinal vein occlusion with swept-source OCT microangiography. Ophthalmic Surg Lasers Imaging Retina. 2015;46:249-252.
46. Ferrara D, Mohler KJ, Waheed N, et al. En face enhanced-depth swept-source optical coherence tomography features of chronic central serous chorioretinopathy. Ophthalmology. 2014;121:719-726.
47. Saxena S, Meyer CH, Sharma SR. Topographic assessment of retinal pigment epithelium detachment in central serous chorioretinopathy by three-dimensional optical coherence tomography single-layer retinal pigment epithelium map. J Ocul Biol Dis Info. 2013;5:44-47.
48. Wang GH, Zhang J, Zhang D, Lv FL, Wang LX. Value of three-dimensional optical coherence tomography and fundus photochromy in correlating the fluorescein leaking sites of acute central serous chorioretinopathy. Med Princ Pract. 2011;20:283-286.
49. Ahn SE, Oh J, Oh JH, Oh IK, Kim SW, Huh K. Three-dimensional configuration of subretinal fluid in central serous chorioretinopathy. Invest Ophthalmol Vis Sci. 2013;54:5944-5952.
50. Saxena S, Manisha, Meyer CH, Akduman L. Three-dimensional spectral domain optical coherence tomography in vitreomacular traction. BMJ Case Rep. 2014 Jun 17;2014.
51. Koizumi H, Spaide RF, Fisher YL, Freund KB, Klancnik JM Jr, Yannuzzi LA. Three-dimensional evaluation of vitreomacular traction and epiretinal membrane using spectral-domain optical coherence tomography. Am J Ophthalmol. 2008;145:509-517.
52. Elbendary AM. Three-dimensional characterization of epiretinal membrane using spectral domain optical coherence tomography. Saudi J Ophthalmol. 2010;24:37-43.
53. Iwasaki T, Miura M, Matsushima C, Yamanari M, Makita S, Yasuno Y. Three-dimensional optical coherence tomography of proliferative diabetic retinopathy. Br J Ophthalmol. 2008;92:713.
54. Michalewski J, Michalewska Z, Cisiecki S, Nawrocki J. Morphologically functional correlations of macular pathology connected with epiretinal membrane formation in spectral optical coherence tomography (SOCT). Graefes Arch Clin Exp Ophthalmol. 2007;245:1623-1631.
55. Pilli S, Zawadzki RJ, Werner JS, Park SS. Visual outcome correlates with inner macular volume in eyes with surgically closed macular hole. Retina. 2012;32:2085-2095.