Three-dimensional OCT for the Evaluation of Chorioretinal Diseases
Multiple perspectives mean more options.
ZACHARY M. BODNAR, MD • LEVENT AKDUMAN, MD
Since its introduction, optical coherence tomography has rapidly become the gold standard for noninvasive, cross-sectional imaging of the retina and vitreoretinal interface in a large number of vitreoretinal diseases. Many spectral-domain OCT devices can produce three-dimensional, volumetric renderings with up-to 2 µm of axial resolution that provides unparalleled visualization of the 3D microstructure of the vitreous, vitreoretinal interface, retina, and retinal pigment epithelium.1
Three-dimensional retinal imaging offers an advantage over cross-sectional OCT in that one need not rely on a few B-scans but may instead quickly visualize a large retinal volume. 3D images can be used to generate tomograms of arbitrary position and orientation in accordance with diagnostic intent, and they enable quantitative measurement, or morphometry, of the intraretinal layers, facilitating early detection and response to treatment in diseases, such as diabetic retinopathy.
These images can also be useful in surgical planning by allowing the surgeon to examine vitreoretinal pathology from multiple perspectives.
APPLICATIONS
Disorders of the Vitreous and Vitreomacular Interface
The International Vitreomacular Traction Study Group has recently introduced an OCT-based anatomical classification for diseases of the vitreomacular interface, including vitreomacular adhesion, vitreomacular traction, and macular hole.2 The standard treatment for VMT is watchful waiting, followed by pars plana vitrectomy to lyse the VMAs when symptomatic. With FDA approval of ocriplasmin (Jetrea, ThromboGenics, Iselin, NJ) in 2012, pharmacologic vitreolysis has become an option for the treatment of VMT in select cases.
Zachary M. Bodnar, MD, is a third-year resident, and Levent Akduman, MD, serves on the faculty of the Department of Ophthalmology at St. Louis University in Missouri. Neither author reports any financial interests in products mentioned in this article. Dr. Akduman can be reached via e-mail at akdumanl@slu.edu.
Results of the phase 3 clinical trials of ocriplasmin indicated that pharmacologic vitreolysis results in a higher rate of nonsurgical resolution of VMA when the adhesion’s diameter was 1,500 µm or less (37.4% vs 14.6%; P=.001) than in cases with more extensive adhesions.3 Closure rates of full-thickness macular holes after ocriplasmin injection were also correlated with the width of the macular hole at baseline.3-5
Three-dimensional OCT can provide a view of VMA and macular holes on multiple axes, and it is very helpful in identifying multifocal adhesions. These abilities make it a useful tool in selecting patients for observation vs pharmacologic vitreolysis or vitrectomy, which may become increasingly important because reports of transient vision loss, dyschromatopsia, and disruption of the ellipsoid zone after injection of ocriplasmin could indicate potential toxicity, and vitrectomy as a surgical intervention certainly exposes patients to surgical risks.6,7
Several studies have characterized the ultrastructure of VMT using 3D-OCT, and the results of the OASIS trial, which further examined selection criteria for ocriplasmin injection, may reveal other ways that 3D-OCT could be beneficial in the evaluation of VMT.8-11 Figure 1 (page 26) shows 3D-OCT of an eye with macular pucker and pseudohole formation.
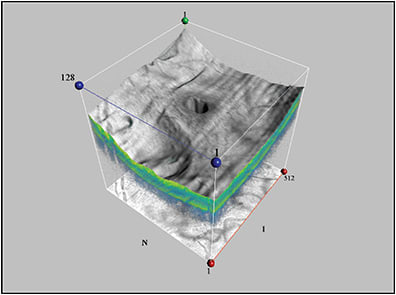
Figure 1. Macular pucker with pseudohole.
Epiretinal membranes that are clinically apparent may or may not be visually significant. Many vitreoretinal surgeons will use the presence of edema or hole formation as additional criteria for proceeding with PPV for macular pucker. It has been shown that distortion of the inner nuclear layer was correlated with symptoms of metamorphopsia. Michalewski et al found that central retinal thickness on 3D-OCT was correlated with visual acuity.10,12,13
Three-dimensional OCT can help determine whether an ERM is causing distortion of the outer retinal layers or is limited to wrinkling of the internal limiting membrane and nerve fiber layer, and it can also be used to locate free edges.14
Similarly, folding of the ILM may be detected only via 3D-OCT in some cases of DME, although the clinical significance of this finding with regard to the planning of vitrectomy vs laser vs medical treatment is not yet understood.15
Three-dimensional OCT can visualize structures within the preretinal vitreous. Kennelly et al described a method for objectively quantifying the shadowing effect of vitreous floaters and vitreous opacities using 3D-OCT.16 Hasegawa et al used 3D-OCT to image a membrane enclosing a hemorrhage in a case of Valsalva retinopathy, which they proposed was fusion of the ILM and posterior hyaloid.17
Vitreoretinal Dystrophies and Degenerations and Congenital Anomalies
In a study by Sayanagi et al, the detection rate of inner segment/outer segment defects, ERMs, macular holes, paravascular microholes, retinal microfolds, and ILM detachments in patients with myopic foveoschisis were all higher when 3D cube scans were used compared to five-line raster scans, although the results were only statistically significant for retinal microfolds.18
Three-dimensional OCT may therefore be useful in surgical planning for myopic foveoschisis to identify the need for ILM peeling and strategies to seal paravascular microholes, which can impact the visual outcome of vitrectomy.
Additionally, 3D choroidal thickness maps have demonstrated decreased choroidal thickness in highly myopic eyes compared to eyes that are closer to emmetropia and that the relative thickness of the choroid in the foveal region is greater in high myopes, which may provide insight into the pathogenesis of this disease.19
Saxena et al described the 3D-OCT imaging findings of individuals with typical and late-onset Stargardt disease (defined as beginning in the late second decade or third decade of life).20 They reported the presence of pisciform flecks diffusely distributed throughout the macula within the outer nuclear, RPE, and sub-RPE layers in typical Stargardt disease, whereas flecks were observed only in paravascular regions of the RPE in late-onset Stargardt disease.
They also reported regional differences in macular atrophy between typical and late-onset Stargardt disease. However, these findings have yet to be replicated in any large case series.
Retinochoroidal anastomosis causes the appearance of dome-shaped RPE elevations on 3D-OCT.21 In this manner, 3D-OCT can be used to identify early choroidal neovascularization in AMD, and it is comparable in sensitivity to fluorescein and indocyanine green angiography. In fact, 3D-OCT may reveal retinochoroidal anastomosis before it becomes apparent via conventional angiography.
In addition, Krebs et al used 3D-OCT to demonstrate that VMA and vitreopapillary adhesion may be important in the pathogenesis of CNV in exudative AMD.22 Figure 2 depicts 3D-OCT of dense subretinal fibrosis and CNV in an eye with advanced AMD.
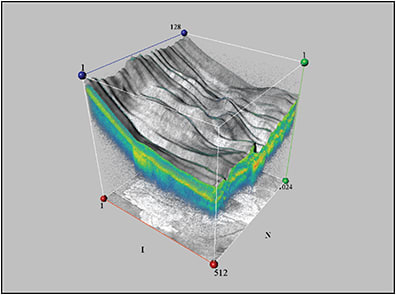
Figure 2. Subretinal scarring and fibrosis secondary to AMD.
Similarly, 3D-OCT with segmentation analysis can identify the pathologic features of polypoidal vasculopathy, including abnormal vascular networks beneath the RPE and hemorrhagic pigment epithelial detachments. One can appreciate a mesh-like configuration of pathologic vessels beneath polypoidal lesions on 3D-OCT. Further, 3D-OCT can image occult lesions that would otherwise only be detected on FA.23
Katome et al used 3D-OCT to demonstrate direct communication among the subretinal fluid pocket, optic disc pit, and subarachnoid space in a case of optic disc pit maculopathy, while Michalewski et al saw connections between optic disc pits and both the vitreous and perineural spaces, suggesting that the fluid may have both liquefied vitreous and cerebrospinal fluid components.24,25
Three-dimensional OCT can identify peripapillary retinal breaks that are not detectable by clinical exam due to obscuration by glial tissue in morning glory disc anomalies. These breaks may not be apparent on 2D-OCT alone. Similarly, 3D-OCT implicates vitreous traction in the pathogenesis of retinal breaks in optic disc colobomas.26
Other interesting uses have been proposed. A case report by Saxena et al illustrated how the 3D geometry of myelinated retinal nerve fibers may create posterior shadowing and relative scotomata.27
Three-dimensional OCT can reveal cavities in X-linked retinoschisis that extend up to the optic nerve head and beyond into the nasal retina.28 Figure 3 shows two views of the macula in an eye with X-linked retinoschisis.
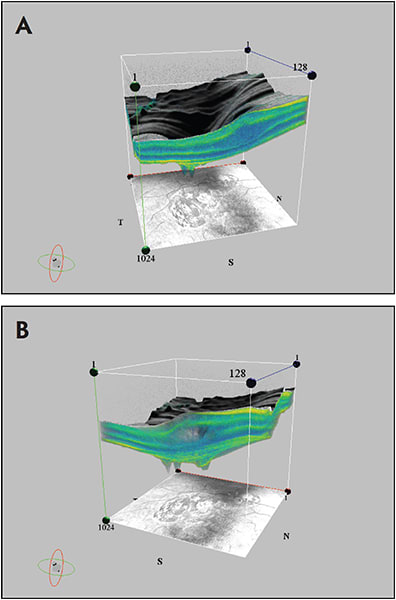
Figure 3. Schisis cavity and foveal contour in x-linked retinoschisis (A); another view of the schisis cavity from the same eye (B).
Proliferative Diabetic Retinopathy
OCT is excellent for viewing the 3D structure of proliferative diabetic membranes. Points of adhesion between the membrane and the retina can be clearly identified. Iwasaki et al described the use of 3D-OCT for the surgical planning of PPV for PDR and tractional retinal detachment.29
Central Serous Chorioretinopathy
Central serous chorioretinopathy is generally self-limiting, but prolonged and recurrent disease can occur, sometimes leading to permanently poor vision. 3D-OCT reveals differences in the 3D configuration of the subretinal fluid in acute and persistent CSC.
The mean peak high of the subretinal fluid and the ratio of the greatest basal diameter of the subretinal fluid are greater in chronic CSC than in acute CSC. Additionally, inferior elongation of subretinal fluid is observed more frequently in persistent than in spontaneously resolving CSC.30
Three-dimensional OCT can visualize RPE cell proliferation and the deposition of fibrin-like material in CSC, as well as focal choroidal excavations that are not easily detected by 2D-OCT.31 3D-OCT can also precisely localize the points of serous leakage within CSC lesions, as demonstrated by Wang et al.32
Although the clinical significance of these findings is unclear, the findings could help to further characterize the morphological and topographic changes in retinal anatomy secondary to CSC and could provide additional understanding of the pathogenesis and pathophysiology of this disorder.
Retinal and Choroidal Neoplasias
While unlikely to replace histopathologic diagnosis anytime soon, high-resolution OCT images correlate well with light-microscopic descriptions of the cellular architecture of intraocular tissues. These images can be used to perform a noninvasive “optical biopsy” of suspicious tissues.
Unlike tissue biopsy, OCT can reveal the 3D cellular architecture of both normal and neoplastic tissue. Shields et al published an excellent review of the OCT findings with numerous types of ocular tumors and dysplasias, including choroidal nevi, melanocytoses, melanomas, metastases, hemangiomas, lymphomas, and osteomas.33 They also reported OCT imaging characteristics of retinal tumors including retinoblastoma, astrocytic hamartoma, hemangioblastoma, and CHRPE.
Various authors have catalogued the topographical and intralesional patterns of OCT imaging that seem to be characteristic of certain intraocular tumors.34,35 However, few reports of 3D-OCT imaging of intraocular tumors exist.
Saxena et al reported a case of choroidal metastasis of breast adenocarcinoma, in which 3D-OCT revealed retinal elevation, serous retinal detachment, RPE hyperplasia, and the presence of particles with low reflectivity within the outer photoreceptor layer.36 A similar case of metastatic non–small-cell lung cancer revealed marked elevation of the retina over the mass, as well as irregularities of the RPE.
OCT imaging of optic disc melanocytomas typically shows a hyper-reflective anterior surface and posterior optical shadowing. The dark pigmentation of these lesions probably prevents light transmission and visualization of the interior details of the tumor.
Nevertheless, 3D-OCT can produce useful images of the adjacent retinal tissue, which can differentiate melanocytomas from other optic disc tumors and can be used to monitor the growth of these lesions over time. 3D-OCT can also be used to visualize retinal nerve fiber layer thinning that corresponds to visual field defects secondary to these tumors.37
Three-dimensional OCT continues to elucidate the morphology and behavior of intraocular tumors. These findings could help to differentiate benign melanocytic lesions from choroidal melanomas and primary intraocular tumors from metastatic disease. It is important to note, however, that OCT should not supersede ultrasound in the evaluation of intraocular tumors, which has superior depth penetration.38,39
Postoperative Evaluation
Favorable outcomes after rhegmatogenous retinal detachment repair and macular hole surgery can be highly dependent on the efficacy of gas tamponade. Gas tamponade produces a characteristic area of hyporeflectivity on 3D-OCT images of the inner segment/outer segment junction viewed en face, corresponding to a region of signal attenuation from the gas/inner retinal interface. This area can be used to confirm adequate compression in the region of interest, although position-dependent evaluation is limited because patients must sit upright during image acquisition.40
Infectious Disease
Saxena et al used 3D-OCT to visualize the intraretinal changes secondary to a choroidal tuberculoma, including granularity of the outer plexiform layer, RPE hyperplasia, and subfoveal neuroretinal detachment. Serial 3D-OCT was used to document the response to antituberculosis therapy in the form of decreased retinal elevation and resolution of subretinal fluid.41
Emerging Developments
The potential of 3D-OCT has only begun to be explored. Improved rendering and visualization technology, as well as new modalities of image acquisition, will likely increase the relevance 3D-OCT in the study and clinical management of vitreal and chorioretinal disease.
Glittenberg et al created a ray-traced, 3D display system for Cirrus HD-OCT (Carl Zeiss Meditec, Dublin, CA) that improves visualization of subtle structures within the vitreous and vitreoretinal interface.42 Aaker et al proposed a virtual reality environment for visualizing 3D renderings of OCT images.43 Techniques to produce 3D-OCT images in true color have been investigated and could enable 3D microscopy.44
Optical microangiography is an OCT-based imaging technology that segments and images retinal and choroidal blood vessels in three dimensions down to the capillary level.45 This technology could one day play a pivotal role in evaluating and treating choroidal and retinal vasculopathies, including exudative AMD, diabetic retinopathy, and many others.
CONCLUSIONS
Although most ophthalmologists rely on 2D cross-sectional OCT in routine practice, 3D-OCT can provide additional diagnostic information that is not readily apparent on cross-sectional OCT or clinical exam. 3D-OCT has been explored for evaluating vitreoretinal and chorioretinal diseases in some limited settings, but as visualization technology improves and becomes more accessible to the clinical ophthalmologist, it has the potential to become a powerful tool for the evaluation of intraocular disease at the tissue level, as well as a flexible surgical guidance tool for the vitreoretinal surgeon. RP
REFERENCES
1. Drexler W, Fujimoto JG. State-of-the-art retinal optical coherence tomography. Prog Retin Eye Res. 2008;27:45-88.
2. Duker JS, Kaiser PK, Binder S, et al. The International Vitreomacular Traction Study Group classification of vitreomacular adhesion, traction, and macular hole. Ophthalmology. 2013;120:2611-2619.
3. Stalmans P, Benz MS, Gandorfer A, et al. Enzymatic vitreolysis with ocriplasmin for vitreomacular traction and macular holes. N Engl J Med. 2012;367:606-615.
4. Stefanini FR, Maia M, Falabella P, et al. Profile of ocriplasmin and its potential in the treatment of vitreomacular adhesion. Clin Ophthalmol. 2014;8:847-856.
5. Syed YY, Dhillon S. Ocriplasmin: a review of its use in patients with symptomatic vitreomacular adhesion. Drugs. 2013;73:1617-1625.
6. Kim JE. Safety and complications of ocriplasmin: ocriplasmin, ocriplasmin; oh, how safe art thou? JAMA Ophthalmol. 2014;132:379-380.
7. Tibbetts MD, Reichel E, Witkin AJ. Vision loss after intravitreal ocriplasmin: correlation of spectral-domain optical coherence tomography and electroretinography. JAMA Ophthalmol. 2014;132:487-490.
8. ClinicalTrials.gov. Ocriplasmin for Treatment for Symptomatic Vitreomacular Adhesion/(VMT) Including Macular Hole (OASIS). ClinicalTrials.gov Web site. Available at: http://ClinicalTrials.gov/ct2/show/NCT01429441. Accessed March 29, 2015.
9. Koizumi H, Spaide RF, Fisher YL, Freund KB, Klancnik JM Jr, Yannuzzi LA. Three-dimensional evaluation of vitreomacular traction and epiretinal membrane using spectral-domain optical coherence tomography. Am J Ophthalmol. 2008;145:509-517.
10. Kumagai K, Hangai M, Furukawa M, Larson E, Ogino N. Three-dimensional foveal shape changes after asymptomatic macular posterior vitreous detachment. Clin Ophthalmol. 2013;7:751-756.
11. Saxena S, Manisha, Meyer CH, Akduman L. Three-dimensional spectral domain optical coherence tomography in vitreomacular traction. BMJ Case Rep. 2014;2014.
12. Okamoto F, Sugiura Y, Okamoto Y, Hiraoka T, Oshika T. Associations between metamorphopsia and foveal microstructure in patients with epiretinal membrane. Invest Ophthalmol Vis Sci. 2012;53:6770-6775.
13. Michalewski J, Michalewska Z, Cisiecki S, Nawrocki J. Morphologically functional correlations of macular pathology connected with epiretinal membrane formation in spectral optical coherence tomography (SOCT). Graefes Arch Clin Exp Ophthalmol. 2007;245:1623-1631.
14. Elbendary AM. Three-dimensional characterization of epiretinal membrane using spectral domain optical coherence tomography. Saudi J Ophthalmol. 2010;24:37-43.
15. Abe S, Yamamoto T, Kashiwagi Y, Kirii E, Goto S, Yamashita H. Three-dimensional imaging of the inner limiting membrane folding on the vitreomacular interface in diabetic macular edema. Jpn J Ophthalmol. 2013;57:553-562.
16. Kennelly KP, Morgan JP, Keegan DJ, Connell PP. Objective assessment of symptomatic vitreous floaters using optical coherence tomography: a case report. BMC Ophthalmol. 2015;15:22-23.
17. Hasegawa T, Ooto S, Yoshimura N. Spectral-domain optical coherence tomography for three-dimensional visualization of the detached membrane in Valsalva retinopathy. Jpn J Ophthalmol. 2010;55:67-85.
18. Sayanagi K, Pelayes DE, Kaiser PK, Singh AD. 3D spectral domain optical coherence tomography findings in choroidal tumors. Eur J Ophthalmol. 2011;21:271-275.
19. Ohsugi H, Ikuno Y, Oshima K, Tabuchi H. 3-D choroidal thickness maps from EDI-OCT in highly myopic eyes. Optom Vis Sci. 2013;90:599-606.
20. Saxena S, Mishra N, Meyer CH. Three-dimensional spectral domain optical coherence tomography in Stargardt disease and fundus flavimaculatus. J Ocul Biol Dis Inform. 2012;5:13-18.
21. Querques G, Avellis FO, Querques L, Massamba N, Bandello F, Souied EH. Three dimensional spectral domain optical coherence tomography features of retinal-choroidal anastomosis. Graefes Arch Clin Exp Ophthalmol. 2012;250:165-173.
22. Krebs I, Glittenberg C, Zeiler F, Binder S. Spectral domain optical coherence tomography for higher precision in the evaluation of vitreoretinal adhesions in exudative age-related macular degeneration. Br J Ophthalmol. 2011;95:1415-1418.
23. Abe S, Yamamoto T, Haneda S, et al. Three-dimensional features of polypoidal choroidal vasculopathy observed by spectral-domain OCT. Ophthalmic Surg Lasers Imaging. 2010;9:1-6.
24. Katome T, Mitamura Y, Hotta F, Mino A, Naito T. Swept-source optical coherence tomography identifies connection between vitreous cavity and retrobulbar subarachnoid space in patient with optic disc pit. Eye (Lond). 2013;27:1325-1326.
25. Michalewski J, Michalewska Z, Nawrocki J. Spectral domain optical coherence tomography morphology in optic disc pit associated maculopathy. Ind J Ophthalmol. 2014;62:777-781.
26. Chang S, Gregory-Roberts E, Chen R. Retinal detachment associated with optic disc colobomas and morning glory syndrome. Eye (Lond). 2012;26:494-500.
27. Saxena S, Jain A. Three-dimensional optical coherence tomography of the optic nerve head with myelinated nerve fibers. J Ocul Biol Dis Informatics. 2011;4:145-148.
28. Saxena S, Manisha, Meyer CH. Three-dimensional spectral domain optical coherence tomography in X linked foveal retinoschisis. BMJ Case Rep. 2013;pii:bcr2012007661.
29. Iwasaki T, Miura M, Matsushima C, Yamanari M, Makita S, Yasuno Y. Three-dimensional optical coherence tomography of proliferative diabetic retinopathy [video report]. Br J Ophthalmol. 2008;92:713
30. Ahn S, Oh J, Oh J, Oh IK, Kim SW, Huh K. Three-dimensional configuration of subretinal fluid in central serous chorioretinopathy. Invest Ophthalmol Vis Sci. 2013;54:5944-5952.
31. Saxena S, Sinha N, Sharma S. Three-dimensional imaging by spectral domain optical coherence tomography in central serous chorioretinopathy with fibrin. J Ocul Biol Dis Inform. 2011;4:149-153.
32. Wang GH, Zhang J, Zhang D, Lv FL, Wang LX. Value of three-dimensional optical coherence tomography and fundus photochromy in correlating the fluorescein leaking sites of acute central serous chorioretinopathy. Med Princ Pract. 2011;20:283-286.
33. Shields C, Pellegrini M, Ferenczy SR, Shields JA. Enhanced depth imaging optical coherence tomography of intraocular tumors. Retina. 2014:1495-1512
34. Arevalo JF, Fernandez CF, Garcia RA. Optical coherence tomography characteristics of choroidal metastasis. Ophthalmology. 2005;112:1612-1619.
35. Torres VL, Brugnoni N, Kaiser PK, Singh AD. Optical coherence tomography enhanced depth imaging of choroidal tumors. Am J Ophthalmol. 2011;151:586-593.
36. Saxena S, Jain A, Akduman L. Three-dimensional spectral domain optical coherence tomography of retina in choroidal metastasis due to breast and lung carcinoma. J Ocul Biol Dis Informatics. 2012;5:9-12.
37. Punjabi OS, Lin CF, Chung HS, Gill MK. Melanocytoma of the optic disc associated with visual field defects: clinical features and imaging characteristics. Ophthalmic Surg Lasers Imaging. 2011;42:e75-e80.
38. Marschall S, Sander B, Mogensen M, Jorgensen TM, Andersen PE. Optical coherence tomography-current technology and applications in clinical and biomedical research. Anal Bioanal Chem. 2011;400:2699-2720.
39. DiBernardo CW, Parekh P, Handa JT, Goldberg, MF. The suboptimal role of optical coherence tomography in diagnosis choroidal metastasis in the macula. Retin Cases Brief Rep. 2010;4:397-400.
40. Muqit MM, Akram I, Turner GS, Stanga PE. Fourier-domain optical coherence tomography imaging of gas tamponade following macular hole surgery. Ophthalmic Surg Lasers Imaging. 2010;41:e1-e6.
41. Saxena S, Singhal V, Akduman L. Three-dimensional spectral domain optical coherence tomography imaging of the retina in choroidal tuberculoma. BMJ Case Rep. 2013;2013.
42. Glittenberg C, Krebs I, Falkner-Radler C, et al. Advantages of using a ray-traced, three-dimensional rendering system for spectral domain cirrus HD-OCT to visualize subtle structures of the vitreoretinal interface. Ophthalmic Surg Lasers Imaging. 2009;40:127-134.
43. Aaker GD, Gracia L, Myung JS, et al. Volumetric three-dimensional reconstruction and segmentation of spectral-domain OCT. Ophthalmic Surg Lasers Imaging. 2011;42(Suppl):S116-S120.
44. Yu L, Kim MK. Full-color three-dimensional microscopy by wide-field optical coherence tomography. Opt Express. 2005;12:6632-6641.
45. An L, Wang RK. In vivo volumetric imaging of vascular perfusion within human retina and choroids with optical micro-angiography. Opt Express. 2008;16:11438-11452.