Intraoperative OCT Today and Tomorrow: The PIONEER Study Two-year Results and Beyond
JUSTIS P. EHLERS, MD
Over the last several years, intraoperative optical coherence tomography (iOCT) has transitioned from a theoretical research tool to a potential real-time surgical assistive device. Numerous vitreoretinal surgical conditions and procedures have now been described in the literature with iOCT, including but not limited to macular holes, epiretinal membranes, retinal detachments, vitreomacular traction, and retinopathy of prematurity.1-8
The high-resolution cross-sectional information provided by OCT creates a unique feedback system for vitreoretinal surgery. Tissue planes, architectural alterations, and residual membranes can all be visualized utilizing iOCT. Surgical utility and specific clinical applications continue to be explored by investigators.
PIONEER STUDY
Over the last three years, the Prospective Intraoperative and Perioperative Ophthalmic ImagiNg with Optical CoherEncE TomogRaphy (PIONEER) study has been conducted at the Cole Eye Institute.
PIONEER is an exploratory study to investigate clinical feasibility, the impact on surgeon decision-making, and the potential safety of a microscope-mounted portable iOCT system. The microscope mount couples the OCT system to foot-pedal control (Figure 1). This increased stability allows for surgeon control of X-Y-Z translational motion.
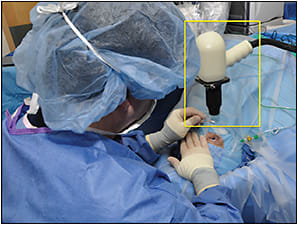
Figure 1. Microscope-mounted portable intraoperative OCT system (yellow box).
The final enrollment for PIONEER includes more than 700 eyes. The two-year results were recently published in the American Journal of Ophthalmology.1 Over 24 months, nearly 550 eyes were enrolled with 275 eyes in the anterior-segment arm and 256 eyes in the posterior-segment arm.
Justis P. Ehlers, MD, is assistant professor of ophthalmology and codirector of intraoperative OCT research in the Retina Service at the Cole Eye Institute of the Cleveland Clinic. He reports financial interests in Bioptigen and Carl Zeiss Meditec. His e-mail address is EHLERSJ@ccf.org.
The vast majority of eyes (98%) could be imaged; however, the image quality varied considerably. Lens status did not appear to impact the ability to perform iOCT in the posterior-segment group. The largest proportion of eyes were pseudophakic (87 eyes) or phakic eyes undergoing concurrent cataract extraction (109 eyes).1
In the PIONEER study, macular indications for surgery, such as macular hole (23%) and ERM (35%), were most common. Retinal detachment and proliferative diabetic retinopathy were also frequent indications for surgery. In membrane peeling cases, iOCT successfully demonstrated completion of peel or residual membranes (Figure 2).
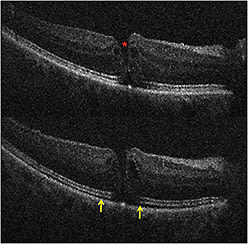
Figure 2. Intraoperative OCT and macular holes. (Top) Preincision intraoperative OCT B-scan revealing full-thickness macular hole (red asterisk). (Bottom) Postpeel intraoperative OCT B-scan revealing expansion of the subretinal hyporeflectivity band between the photoreceptor layers and the retinal pigment epithelium (yellow arrows).
Macular Surgeries
In macular hole cases, alterations in hole geometry were noted, and increased hyporeflectivity in the subretinal space was demonstrated, similar to previous studies.1,4,6 Partially peeled internal limiting membrane was often identified as a curled, hyperreflective, thin band on the B-scan.1,6
For ERM cases, residual membranes could be identified, and alterations to retinal architecture were also noted (Figure 3, page 28).1,4 Following membrane peeling, changes in the distance between the photoreceptor layers and the retinal pigment epithelium were qualitatively visualized, similar to previous descriptions.1,4,9 These included subtle changes in the hyporeflective band between the photoreceptor layers and the RPE, as well as more significant changes in full-thickness retina.1,9
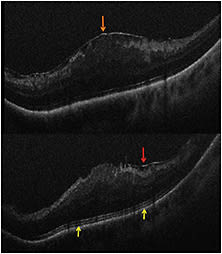
Figure 3. Intraoperative OCT and ERM. (Top) Preincision intraoperative OCT B-scan revealing prominent epiretinal membrane (orange arrow). (Bottom) Postpeel intraoperative OCT B-scan revealing expansion of the subretinal hyporeflectivity band between the photoreceptor layers and the retinal pigment epithelium (yellow arrows). A residual membrane is also identified (red arrow).
Vitreomacular traction is an additional posterior-segment condition that is amenable to iOCT feedback. The PIONEER study and other reports have successfully obtained visualization following hyaloid elevation, allowing the surgeon to identify occult full-thickness macular holes or confirm inner retinal continuity, which can directly impact surgeon decision-making by directing ILM peeling and/or gas tamponade.1,8
PDR and RD
Cases of PDR and vitreous hemorrhage were also included in the PIONEER study. Utilizing iOCT in vitreous hemorrhage cases may be particularly helpful due to the surgeon’s inability to know the preoperative macular status (eg, presence of membranes, macular edema).
For complex PDR cases, iOCT may provide critical information on surgical planes and identification of residual traction. Rhegmatogenous retinal detachments were also a frequent diagnosis in the PIONEER study (13%). The PIONEER study confirmed previously published findings of frequent persistent subretinal fluid under perfluorocarbon tamponade (Figure 4, page 29).1,5,9
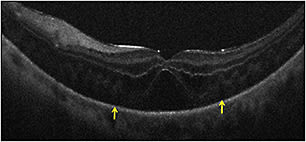
Figure 4. Intraoperative OCT and retinal detachment. Intraoperative OCT B-scan following perfluorocarbon liquid tamponade reveals residual subretinal fluid (yellow arrows).
Ongoing analysis will examine the functional relationships of these findings. One previous study suggested that the retinal configuration under perfluorocarbon may have prognostic information for visual outcomes.5
iOCT Utility and Safety
The PIONEER study utilized surgeon feedback forms to assess the impact of iOCT on surgeon decision-making. In 43% of cases with membrane peeling, surgeons reported that the iOCT impacted their understanding of the surgical configuration and/or surgical procedure. In 13% of cases, iOCT revealed occult residual membrane that the surgeon had not identified that required peeling.1
A significant question in iOCT is the time burden required to perform the procedure. In PIONEER, the typical time required to perform a scan session (ie, a series of OCT scans performed during a single pause of surgery) was less than four minutes. The median number of scans performed in the posterior-segment portion of the study was 10 volume scans.
In the vast majority of cases, this represents a significantly higher number than would be needed from a clinical standpoint, because these were obtained based on a standard research scanning protocol.1 The study utilized a microscope-mounted iOCT system and a dedicated research coordinator.
In the posterior-segment arm of PIONEER, there were no cases of endophthalmitis or serious adverse events. Because this was a surgical study, there was an expected baseline rate of observed adverse events, including elevated intraocular pressure, hypotony, and epithelial defects.1
INTEGRATION AND THE FUTURE
PIONEER represents the largest study to date examining the feasibility, utility, and safety of iOCT. However, the PIONEER study utilized an external OCT system for intraoperative imaging. Although image quality can be excellent, and feedback can be quick, an external system still requires surgery to be paused.
Additionally, the system can be difficult to aim, focus, and direct to the area of interest. The future of iOCT as a widely used surgical tool will likely rely on integration into the surgical microscope and other integrative technological advances.
Numerous integrated systems have now been described in the literature. Ongoing clinical studies with integrated systems, including the DISCOVER study at the Cleveland Clinic, will help to define the role of these systems better in surgical care.10
The integrated systems in the literature include microscope add-ons that are placed within the optical path of the microscope (eg, Duke prototype, Cleveland Clinic prototype), as well as built-in systems that have minimal impact on the microscope footprint (eg, Carl Zeiss Meditec’s RESCAN 700 [Dublin, CA]).7,9-13
Microscope integration may provide significant advantages, including rapid scan localization, real-time tissue-instrument interaction visualization, and immediate feedback to the surgeon.
Initial early research systems have been described; however, more recently, commercial systems are being developed for more widespread availability. Carl Zeiss Meditec and Haag-Streit (Köniz, Switzerland) both have integrated systems that are available in Europe. FDA clearance is still pending in the United States. Bioptigen, Inc. (Durham, NC) also just announced plans for a microscope-integrated prototype.
Changes to Displays and Instrumentation
Heads-up display will be a key feature of real-time feedback. The heads-up display could feasibly provide surgeon-critical information regarding the OCT data stream and the surgical environment, allowing for rapid adaptation to potential changes in surgical approach. Both the Zeiss RESCAN 700 and the Cole Eye Institute integrated prototype utilize heads-up display technology.9,10,13
Surgical instrumentation will likely need to evolve for maximum benefit from iOCT. Current metallic instrumentation results in significant obscuration of the underlying tissue due to extensive shadowing.11
Additionally, the light-scattering properties typically minimize the visualization of the instrument on iOCT. Prototype development of novel surgical tools with unique materials have been described, which maximize visualization of underlying tissues while maintaining visualization of the tool itself.13 These instruments may help to facilitate true real-time OCT-guided surgical interventions.
Software development with surgical pathology-specific indications may help to guide surgeon decision-making and help facilitate our understanding of the changes in anatomy that occur during surgical manipulations, such as changes in fluid interfaces or macular hole geometry.6,14,15 Further development is needed and is under way in clinical outcomes prediction and modeling with iOCT software solutions.
CONCLUSIONS
The field of iOCT is currently undergoing exciting changes and developments. Significant recent iterative advances, including microscope integration, heads-up display systems, and surgical instrument prototyping, have the potential to transform iOCT from a research tool to a widespread surgical assistive device.
The PIONEER two-year results validate the potential of this technology in surgeon feedback and the need for continued research into the role for iOCT and its potential for maximizing outcomes in ophthalmic surgery. RP
REFERENCES
1. Ehlers JP, Dupps WJ, Kaiser PK, et al. The Prospective Intraoperative and perioperative Ophthalmic imagiNg with optical coherEncE tomogRaphy (PIONEER) study: 2-year results. Am J Ophthalmol. 2014 Jul 28. [Epub ahead of print]
2. Dayani PN, Maldonado R, Farsiu S, Toth CA. Intraoperative use of handheld spectral domain optical coherence tomography imaging in macular surgery. Retina. 2009;29:1457-1468.
3. Chavala SH, Farsiu S, Maldonado R, Wallace DK, Freedman SF, Toth CA. Insights into advanced retinopathy of prematurity using handheld spectral domain optical coherence tomography imaging. Ophthalmology. 2009;116:2448-2456.
4. Ray R, Baranano DE, Fortun JA, et al. Intraoperative microscope-mounted spectral domain optical coherence tomography for evaluation of retinal anatomy during macular surgery. Ophthalmology. 2011;118:2212-2217.
5. Ehlers JP, Ohr MP, Kaiser PK, Srivastava SK. Novel microarchitectural dynamics in rhegmatogenous retinal detachments identified with intraoperative optical coherence tomography. Retina. 2013;33:1428-1434.
6. Ehlers JP, Xu D, Kaiser PK, Singh RP, Srivastava SK. Intrasurgical dynamics of macular hole surgery: an assessment of surgery-induced ultrastructural alterations with intraoperative optical coherence tomography. Retina. 2014;34:213-221.
7. Binder S, Falkner-Radler CI, Hauger C, Matz H, Glittenberg C. Feasibility of intrasurgical spectral-domain optical coherence tomography. Retina. 2011;31:1332-1336.
8. Ehlers JP, Tam T, Kaiser PK, Martin DF, Smith GM, Srivastava SK. Utility of intraoperative optical coherence tomography during vitrectomy surgery for vitreomacular traction syndrome. Retina. 2014;34:1341-1346.
9. Ehlers JP, Tao YK, Srivastava SK. The value of intraoperative optical coherence tomography imaging in vitreoretinal surgery. Curr Opin Ophthalmol. 2014;25:221-227.
10. Ehlers JP, Kaiser PK, Srivastava SK. Intraoperative optical coherence tomography using the RESCAN 700: preliminary results from the DISCOVER study. Br J Ophthalmol. 2014 Apr 29. [Epub ahead of print]
11. Ehlers JP, Tao YK, Farsiu S, Maldonado R, Izatt JA, Toth CA. Integration of a spectral domain optical coherence tomography system into a surgical microscope for intraoperative imaging. Invest Ophthalmol Vis Sci. 2011;52:3153-3159.
12. Tao YK, Srivastava SK, Ehlers JP. Microscope-integrated intraoperative OCT with electrically tunable focus and heads-up display for imaging of ophthalmic surgical maneuvers. Biomed Opt Express. 2014;5:1877-1885.
13. Ehlers JP, Srivastava SK, Feiler D, Noonan A, Rollins A, Tao YK. Integrative advances for OCT-guided ophthalmic surgery and intraoperative OCT: microscope integration, surgical instrumentation, and heads-up display surgeon feedback. PLoS One. 2014 (in press).
14. Xu D, Dupps WJ, Srivastava SK, Ehlers JP. Automated volumetric analysis of interface fluid in Descemet stripping automated endothelial keratoplasty utilizing intraoperative optical coherence tomography. Invest Ophthalmol Vis Sci. 2014 Aug 7. [Epub ahead of print]
15. Xu D, Yuan A, Kaiser PK, et al. A novel segmentation algorithm for volumetric analysis of macular hole boundaries identified with optical coherence tomography. Invest Ophthalmol Vis Sci. 2013;54:163-169.