Widefield OCT: Current and Future Applications
When retinal pathology goes beyond the macula, imaging can pose a challenge.
ASHLEIGH LEVISON, MD • JUSTIS P. EHLERS, MD
The introduction of optical coherence tomography has transformed the diagnosis and management of vitreoretinal disorders.1 OCT has become the most important imaging technique used in the everyday practice of retina.
The technology provides noninvasive, high-resolution, cross-sectional images of the eye that we are unable to obtain through any other available method.2,3 Since its original development, the capabilities of OCT imaging have significantly broadened. Advanced technology has increased the speed, resolution, and depth of imaging.4
Many factors, including the patient’s eye movements, can affect the quality and resolution of OCT images.4 Consequently, the speed of image acquisition plays a vital role in the resolution of the images obtained.
FROM TIME DOMAIN TO SWEPT SOURCE
When OCT technology was first introduced for imaging of the retina, the axial resolution was approximately 15 µm. However, OCT technology has improved significantly, allowing for imaging with axial resolution of up to 3 µm.2,5,6
The original OCT technology commercially available in 2002 was time-domain OCT. It used an infrared light source with a wavelength of approximately 840 nm. The speed of imaging with TD-OCT was limited by the required movement of a mirror to change the optical path of the reference beam.1,3 This requirement limited the overall sampling density and resolution of images with only a few thousand A-scans acquired per second.3
Ashleigh Levison, MD, is a retina fellow at the Cole Eye Institute of the Cleveland Clinic in Ohio. Justis P. Ehlers, MD, is assistant professor of ophthalmology at the Cole Eye Institute. Neither author reports any financial interests in any products mentioned in this article. Dr. Ehlers’ e-mail is ehlersj@ccf.org.
The newer technology of spectral-domain OCT or Fourier-domain OCT does not use a moving mirror but instead uses an array of detectors (ie, a spectrometer), so scanning speeds are now 50 to 200 times faster than with TD-OCT.
This increase in speed has allowed SD-OCT to have greater resolution with significantly shorter acquisition times.4 In addition, SD-OCT helps minimize motion artifact and provides volumetric analysis with three-dimensional imaging capabilities.1,7,8
Among the most recent technologies to become available is swept source (SS)-OCT. SS-OCT uses a 1,050- to 1,060-nm light source and is able to obtain 100,000 to 400,000 A-scans/second.9
The advantages of SS-OCT over SD-OCT include a further reduction in motion artifact and a reduction in the impact of cataracts and other ocular opacities. The increased wavelength of SS-OCT allows for imaging at increased depth with improved visualization of choroidal details.6,10
THE EXPANSION INTO THE PERIPHERY AND WIDEFIELD OCT
As OCT technology continues to expand for both SD-OCT and SS-OCT, an emerging area of interest is widefield OCT. Recent advances in widefield technology in other imaging modalities, such as angiography and autofluorescence, have opened new doors to understanding disease pathogenesis and underlying pathophysiology.
Currently, the majority of available OCT machines provide 6- to 9-mm B-scan lengths, and the current software is also primarily geared toward macular imaging.
However, retinal pathology is often not isolated to a small cube in the macula, and in many cases, it is not limited to the macula itself. Either expansion of B-scan length or targeted peripheral OCT to an area of interest may improve visualization of extramacular vitreoretinal pathologies.
For example, by having the patient adjust his or her gaze, the physician can obtain images outside the central macula. However, image size is still limited, and the resolution may decrease.
Image Montage and Device Adaptation
Various methods can be used to increase the effective area scanned with an OCT device. To obtain OCT images over a wider area, multiple images can be montaged together. Gregori et al imaged patients with X-linked retinoschisis using multiple SD-OCT images that were montaged together to create an image 50º horizontal and 35º vertical of the posterior pole.11
While this technique can provide more detailed information over a greater area, it takes more time and significant effort to create montaged images. The mounting of an OCT machine to a slit lamp has also been previously shown to successfully expand the potential area of retina that can be imaged, compared to the range of commercially available OCT devices.12
Recent developments in widefield OCT have now made it possible to take wider scan-length OCT B-scans without having to montage the pictures together.
Widefield Scanning
Recently, commercial widefield OCT has become available in the United States with the Optovue (Fremont, CA) Avanti RTVue-XR. This widefield system uses SD technology and can obtain 70,000 A-scans/second. The Avanti RTVue-XR can create 12 mm x 9 mm B-scans, and its active eye tracking enhances image stability. The Optovue system offers 3-µm digital resolution, providing detailed imaging of both the choroid and the retina (Figure 1, page 39).13
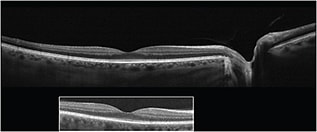
Figure 1. This image demonstrates the difference in imaging width between widefield SD-OCT and standard OCT (inset).
No other machines using widefield technology are currently FDA-approved. Other widefield OCT devices that use both SD- and SS-OCT technologies are under development. Because of the higher scanning speed of the SS-OCT 1,060-nm system, wider scans can be obtained in the same acquisition time as a standard scan on the SD system.14 These faster systems can collect between 684,000 and 1,368,700 A-scans/second for outstanding volumetric acquisition speed.10
CLINICAL APPLICATIONS
The application of widefield OCT and its adoption into clinical practice may provide significant benefits in the imaging of retinal disorders. First, widefield OCT will likely enhance visualization of macular disorders that span greater areas than standard scan widths. Second, application of OCT technology to the retinal periphery could expand our understanding of peripheral vitreoretinal diseases.
These advancements in OCT image acquisition will hopefully have a positive impact on both clinical and surgical care, as well as on future research into retinal disorders.
Macular Pathology
Macular pathology can be multifocal, and regions of disease may be physically separated by more than the standard B-scan length, making it impossible to image macular pathology comprehensively without montaging.
For example, in multifocal central serous retinopathy, widefield technology may provide improved visualization of multiple pigment epithelial detachments and numerous foci of subretinal fluid.
Peripapillary choroidal neovascular membranes represent another pathology, the clinical care of which may be positively affected by the use of widefield OCT. Often, the extent and relative location of subretinal fluid associated with peripapillary CNV can be difficult to assess with standard B-scan lengths. Utilizing widefield OCT to include the fovea and optic nerve simultaneously may allow for better monitoring of the proximity of subretinal fluid to the fovea, as well as treatment response.
Vitreoretinal Relationships
Widefield OCT has the potential to aid in the surgical planning of vitreoretinal surgical cases as well. The vitreoretinal relationship in pathologic conditions, such as vitreomacular traction or proliferative diabetic retinopathy, may be difficult to delineate with standard OCT scan lengths. Widefield OCT may outline these relationships better, from both cross-sectional and three-dimensional perspectives.
The ability to image the fovea in addition to the optic nerve and other areas of vitreoretinal adhesion (eg, vascular arcades) with widefield OCT may result in a better understanding of the relationship of the hyaloid and the retina and could improve surgical planning.
Research studies looking at the use of intraoperative OCT are under way.15 Experience has shown thus far that it may have great utility in vitreoretinal surgery and could alter the course of surgery and/or postoperative care.16,17 Current intraoperative OCT systems are limited to standard scan length.
As widefield OCT technology improves, the translation of this technology to the operating room may also enhance feedback to surgeons through better visualization of the impact of surgical maneuvers at areas distant from a specific location of interest.
The Retinal Periphery
The retinal periphery remains an area of exploration from an OCT standpoint. Targeted OCT of standard scan length, as well as utilization of peripheral widefield OCT, could enhance our diagnostic capabilities. OCT scans of the peripheral retina would likely aid in the diagnosis and follow-up of peripheral retinal disorders.
In certain circumstances, the clinical exam cannot definitively delineate the anatomic layer of an abnormality of interest. Being able to perform OCT imaging of the area of interest may help with diagnosis and planning for care.
For example, differentiation of retinal detachments and retinoschisis can be difficult at times based on the clinical exam alone. Targeted peripheral OCT may allow for the clear delineation of the retinal layers and improved diagnosis of splitting of retinal layers vs full-thickness retinal elevation.
The application of widefield OCT to this clinical situation may also improve localization of the retinal pathology relative to the fovea and facilitate documentation of disease progression.
Characterization of choroidal lesions (eg, malignancies) could also improve using targeted peripheral OCT or widefield OCT. OCT patterns were recently described that outlined key features that may be diagnostic of particular choroidal lesions.18
CLINICAL CASE EXAMPLES
The following three cases represent clinical scenarios in which targeted peripheral OCT or widefield OCT might be beneficial.
Peripheral CSR
A 38-year-old man complained of a three-day history of an inferotemporal scotoma in his right eye. His vision was 20/20 in both eyes. On clinical examination, he had a PED with indeterminate subretinal fluid superonasal to the optic nerve in the right eye.
Spectral-domain OCT confirmed both a PED and subretinal fluid. The clinical exam, OCT, and fluorescein angiography were consistent with the diagnosis of peripheral CSR. Targeting the OCT to the area of interest allowed for confirmation of the diagnosis.
The availability of widefield OCT may have allowed for imaging of the PED and optic nerve within the same scan. Targeted OCT allows for improved assessment of stability or progression of the extent of subretinal fluid at follow-up (Figure 2, page 39).
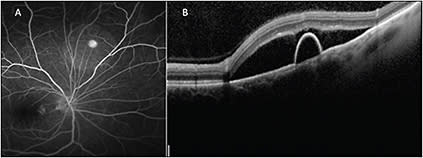
Figure 2. Fluorescein angiography (A) shows a pinpoint area of hyperfluorescence consistent with the diagnosis of CSR. A peripheral targeted SD-OCT B-scan (B) shows both subretinal fluid and a pigment epithelial detachment in a patient with peripheral CSR.
Choroidal Melanoma
A 33-year-old woman presented with a complaint of a scotoma in her right eye. Her referring ophthalmologist was concerned about an exudative retinal detachment. The patient’s visual acuity was 20/20 in the affected eye.
Targeted OCT to the area of interest demonstrated that she had a large choroidal lesion with mild subretinal fluid that, after workup, was determined to be a choroidal melanoma (Figure 3, page 40).
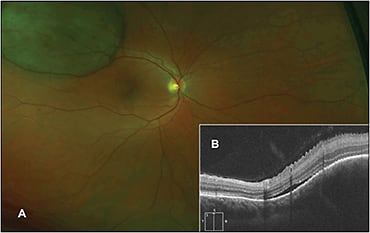
Figure 3. Fundus photo (A) of a superotemporal mass in the right eye. The targeted peripheral SD-OCT (B) shows a large choroidal elevation with minimal subretinal fluid.
In this case, OCT helped to guide the diagnosis and helped to delineate the primary location of the lesion (ie, the choroid).
Retinoschisis vs Retinal Detachment
A 69-year-old woman without any visual complaints underwent a routine eye exam. She had significant elevation of the retinal surface in both eyes (OD>OS). A diagnosis of retinoschisis was highest on the differential list. Targeted OCT confirmed the diagnosis of retinoschisis vs a retinal detachment (Figure 4, page 41).
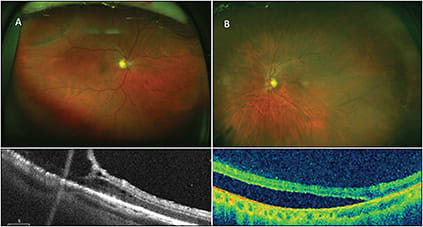
Figure 4. Fundus photograph (A) with superotemporal retinoschisis. Targeted peripheral SD-OCT beneath the photo shows splitting of the retinal layers consistent with retinoschisis. Fundus photograph (B) of an inferotemporal retinal detachment. Targeted peripheral SD-OCT beneath confirms the presence of subretinal fluid, confirming the diagnosis of retinal detachment.
CONCLUSION
We have already witnessed the transformational power of OCT for the diagnosis and management of vitreoretinal diseases, particularly macular disease. The introduction and integration of widefield OCT and/or targeted peripheral OCT into the practice of retina may also have a significant impact on the understanding and management of multifocal macular diseases, vitreoretinal interface pathologies, and peripheral retinal conditions. Only time will reveal the overall impact of this technology on the practice of retina. RP
REFERENCES
1. Major JC, Wykoff CC, Mariani AF, Chen E, Croft DE, Brown DM: Comparison of spectral-domain and time-domain optical coherence tomography in the detection of neovascular age-related macular degeneration activity. Retina. 2014;34:48-54.
2. Fujimoto JG, Pitris C, Boppart SA, Brezinski ME: Optical coherence tomography: an emerging technology for biomedical imaging and optical biopsy. Neoplasia. 2000;2:9-25.
3. Ryan SJ, Puliafito CA, Davis JL, Parel JM, Milne P. Retina. 4th ed. Philadelphia, PA: Elsevier Mosby; 2006.
4. Klein T, Wieser W, Reznicek L, Neubauer A, Kampik A, Huber R. Multi-MHz retinal OCT. Biomed Opt Express. 2013;4:1890-1908.
5. Yannuzzi LA, Ober MD, Slakter JS, et al. Ophthalmic fundus imaging: today and beyond. Am J Ophthalmol. 2004;137:511-524.
6. Grulkowski I, Liu JJ, Potsaid B, et al. Retinal, anterior segment and full eye imaging using ultrahigh speed swept source OCT with vertical-cavity surface emitting lasers. Biomed Opt Express. 2012;3:2733-2751.
7. Adhi M, Duker JS. Optical coherence tomography — current and future applications. Curr Opin Ophthalmol. 2013;24:213-221.
8. Sull AC, Vuong LN, Price LL, et al. Comparison of spectral/Fourier domain optical coherence tomography instruments for assessment of normal macular thickness. Retina. 2010;30:235-245.
9. Michaelson S. Emerging innovations in OCT technology. Retin Physician. 2013;10(6):62-65.
10. Raiji V, Walsh A, Sadda S. Future directions in retinal optical coherence tomography. Retin Physician. 2012:9(4):33-37.
11. Gregori NZ, Lam BL, Gregori G, et al. Wide-field spectral-domain optical coherence tomography in patients and carriers of X-linked retinoschisis. Ophthalmology; 2013;120:169-174.
12. Stehouwer M, Verbraak FD, de Vries HR, van Leeuwen TG. Scanning beyond the limits of standard OCT with a Fourier domain optical coherence tomography integrated into a slit lamp: the SL SCAN-1. Eye (Lond). 2011;25:97-104.
13. Optovue: Avanti RTVue XR - Widefield En Face OCT. Brochure.
14. Povazay B, Hermann B, Hofer B, et al. Wide-field optical coherence tomography of the choroid in vivo. Invest Ophthalmol Vis Sci. 2009;50:1856-1863.
15. Ehlers JP, Tao YK, Farsiu S, Maldonado R, Izatt JA, Toth CA. Integration of a spectral domain optical coherence tomography system into a surgical microscope for intraoperative imaging. Invest Ophthalmol Vis Sci. 2011;52:3153-3159.
16. Ehlers JP, Ohr MP, Kaiser PK, Srivastava SK. Novel microarchitectural dynamics in rhegmatogenous retinal detachments identified with intraoperative optical coherence tomography. Retina. 2013;33:1428-1434.
17. Ehlers JP, Xu D, Kaiser PK, Singh RP, Srivastava SK. Intrasurgical dynamics of macular hole surgery: an assessment of surgery-induced ultrastructural alterations with intraoperative optical coherence tomography. Retina. 2013;34:213-221.
18. Shields CL, Kaliki S, Rojanaporn D, Ferenczy SR, Shields JA. Enhanced depth imaging optical coherence tomography of small choroidal melanoma: comparison with choroidal nevus. Arch Ophthalmol. 2012;130:850-856.