Peer Reviewed
Visual Cycle Modulation: A Novel Therapeutic Approach For Treatment of GA In Dry AMD
Has the “holy grail” of retina been found?
NATHAN L. MATA, PhD • RYO KUBOTA, MD, PhD • PRAVIN U. DUGEL, MD
Over the last decade, as treatments have emerged to slow the progression of wet AMD, the focus of new drug development has shifted to treatments for geographic atrophy, the most severe manifestation of dry AMD. Although our knowledge of GA pathophysiology is still evolving, numerous investigational agents to treat GA have been developed based on hypothesized etiologies.
Of particular note is an orally available small molecule, which is intended to reduce the accumulation of vitamin A–based toxins as a means of slowing the progression of GA lesions. This is compound, designated emixustat hydrochloride, is a new molecular entity currently being evaluated in a multicenter phase 2b/3 trial as a treatment for GA associated with dry AMD (ClinicalTrials.gov identifier: NCT01802866).
Emixustat is the first agent in a class of novel pharmacological compounds, called visual cycle modulators, which are designed specifically to target enzymes of the visual cycle. In this review, various aspects of emixustat are examined.
VITAMIN A-BASED TOXINS IN THE PATHOPHYSIOLOGY OF GA
A prominent early feature of GA is the aberrant accumulation of autofluorescent cellular debris, or lipofuscin, within the retinal pigment epithelium. Lipofuscin is an amalgamation of lipids, proteins, and vitamin A derivatives that forms during daily phagocytic uptake of spent photoreceptor outer segment tips of the retina.1
Nathan L. Mata, PhD, is Principal Clinical Research Scientist at Acucela, Inc., in Seattle, WA. Ryo Kubota, MD, PhD, chairman, president, and CEO of Acucela. Pravin U. Dugel, MD, is managing partner of Retinal Consultants of Arizona in Phoenix and a founding member of Spectra Eye Institute in Sun City, AZ. Drs. Mata and Kubota report significant financial interest, and Dr. Dugel reports minimal financial interest in Acucela.Dr. Dugel can be reached via e-mail at pdugel@gmail.com. |
The unique light-emitting nature of lipofuscin allows for noninvasive imaging by fundus autofluorescence photography. FAF imaging in GA patients shows areas of intense autofluorescence surrounding the leading edges of GA lesions.2-4
Importantly, this autofluorescence has been shown to precede the spreading of lesions and the death of retinal tissue,5,6 suggesting the presence of cytotoxic compounds within ocular lipofuscin.
The most prominent autofluorescent compounds identified in human ocular lipofuscin are bisretinoids, such as N-retinylidene-N-retinylethanolamine (A2E). A2E, a condensation product of two molecules of alltrans retinal and ethanolamine, is a well-characterized cytotoxin, which has been shown to have several deleterious effects on RPE cells, including generation of reactive oxygen species,7,8 impairment of lysosomal degradative functions,9,10 induction of proapoptotic proteins,11 complement activation,12 and upregulation of vascular endothelial growth factor in the RPE and choroid.13 The myriad cytotoxicities associated with A2E have implicated it in the pathophysiology of GA.
VISUAL CYCLE MODULATION
A2E is generated as a by product of vitamin A flux through the visual cycle.14 Therefore, approaches to reducing A2E accumulation have been directed at modulation of the enzymes that mediate intracellular processing of vitamin A in the visual cycle.
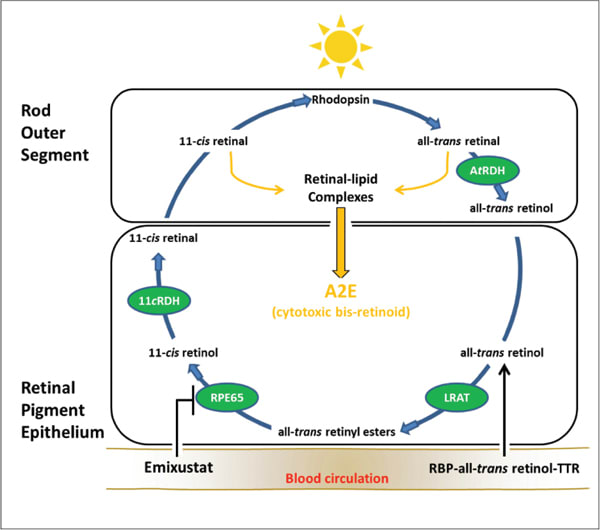
Figure 1. Visual cycle modulation with emixustat. A schematic illustration of the visual cycle and the target of emixustat are shown. Enzymatic processing within the visual cycle begins with delivery of vitamin A (all-trans retinol) from the blood circulation as a ternary complex bound to retinol binding protein (RBP) and transthyretin (TTR). Upon entry into the RPE, all-trans retinol is converted to a retinyl ester through the activity of lecithin retinol acyl transferase (LRAT). The resulting all-trans retinyl esters represent a storage form of vitamin A, upon which RPE65 acts. Following conversion to 11-cis retinol, an 11-cis specific retinol dehydrogenase (11cRDH) catalyzes the formation of the visual chromophore 11-cis retinal. The visual chromophore is then delivered to rod and cone outer segments, where it combines with opsins to form visual pigments (eg, rhodopsin). Light activation of rhodopsin initiates the process of visual transduction and liberates all-trans retinal as a photoproduct. Reduction of all-trans retinal, via all-trans retinal dehydrogenase (AtRDH), produces all-trans retinol, which is transferred back to the RPE for recycling. During periods of continued light exposure, 11-cis and all-trans retinal levels increase in outer segment membranes, leading to the formation of retinal-lipid complexes, which are precursors of A2E. Emixustat reduces levels of 11-cis and all-trans retinal by limiting the rate of vitamin A processing through inhibition of RPE65. Modulation of the visual cycle in this manner leads to a reduction of the retinal-lipid complexes and A2E.
The isomerase of the visual cycle, RPE-specific 65-kDa protein (RPE65), has been a preferred target for modulating the visual cycle, based upon its localized expression in the eye and its unique role in the generation of visual chromophore.15–19
Indeed, in animal models of excessive A2E accumulation, compounds that inhibit the activity of RPE65 are effective in reducing A2E levels, and they significantly ameliorate retinal pathology.15,18
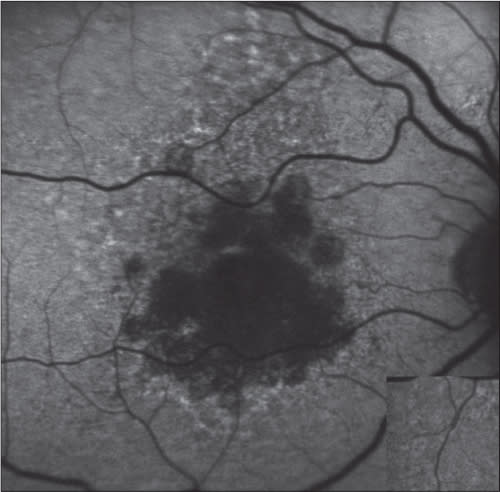
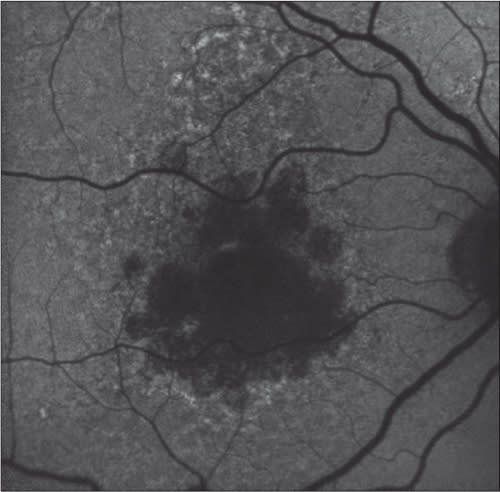
Figure 2. Fundus autofluorescence taken at baseline (left) and at day 90 (right). This patient was treated with 5 mg emixustat and had a rod B-wave suppression of ~60%.
RPE65 acts on stores of vitamin A (all-trans retinyl esters) contained within the RPE to generate a lightsensitive retinoid (11-cis retinol). This critical step in the visual cycle is rate-limiting for the generation of visual chromophore, 11-cis retinal.
Photobleaching of 11-cis retinal liberates all-trans retinal, which is subsequently returned to the RPE for recycling. During periods of continued light exposure, the high levels of 11-cis and all-trans retinal in photoreceptor outer segment membranes facilitate the formation of retinal-lipid complexes, which are precursors of A2E.
The normal diurnal process of outer segment disc shedding and phagocytosis directs the retinallipid complexes to phagolysosomes of the RPE, where the A2E molecule is formed and accumulates (Figure 1, page 21).
Emixustat was designed as an antagonist of RPE65 to reduce 11-cis and all-trans retinal levels in the retina and slow the biosynthesis of A2E. By inhibiting the activity of RPE65, emixustat essentially reduces the rate of vitamin A processing in the visual cycle. The developers of emixustat believe that reduction of A2E through visual cycle modulation may be useful in preserving the health and integrity of the retina.
PHARMACODYNAMIC AND PHARMACOKINETIC PROPERTIES
An obligate effect of emixustat treatment is reduced rod photoreceptor activity, due to reduced visual chromophore levels in the retina. This effect serves as a pharmacodynamic marker of emixustat activity in the eye and can be readily assessed by ERG measurement.
Data from the phase 1 safety study of emixustat, which showed a dose-dependent suppression of rod photoreceptor recovery following a photobleach, are consistent with the proposed mechanism of action.24 Pharmacokinetic data from this same study showed dose-proportionate increases in peak plasma concentrations, with maximal concentrations being achieved at ~4 hours following emixustat administration. The mean terminal elimination half-life ranged from four to six hours.
Importantly, emixustat’s effects on rod photoreceptor activity was measurable within hours after initial dosing and was sustained for at least 24 hours (Figure 2). Emixustat appears to act quickly at the target site (RPE65), and its effect on rod photoreceptors is sustained beyond the time point of elimination in plasma. This pharmacokinetic profile supports a once-daily regimen for emixustat treatment.
CONCLUDING REMARKS
Modulation of vitamin A levels in the eye, to reduce the accumulation of retinal toxins and preserve retinal tissue, is the intended effect of emixustat. The ability of emixustat to target RPE65 specifically, as a means of slowing the production of A2E and related bis-retinoids, provides a unique opportunity to evaluate the hypothesis that these compounds play a role in progression of GA lesions.
The mechanism of emixustat’s action and assessment of its pharmacologic activity by ERG offer distinct advantages. For example, a comparison of the pharmacologic activity of emixustat with treatment efficacy at the conclusion of the current phase 2b/3 study may reveal informative correlations between rod photoreceptor function and lesion growth. These data may be useful for future clinical development efforts in GA and phenotypically related retinopathies (eg, Stargardt disease). RP
REFERENCES
1. Katz ML, Robison WG Jr. What is lipofuscin? Defining characteristics and differentiation from other autofluorescent lysosomal storage bodies. Arch Gerontol Geriatr. 2002;34:169-184.
2. Schmitz-Valckenberg S, Bindewald-Wittich A, Dolar-Szczasny J, et al. Correlation between the area of increased autofluorescence surrounding geographic atrophy and disease progression in patients with AMD. Invest Ophthalmol Vis Sci. 2006;47:2648-2654.
3. Schmitz-Valckenberg S, Bultmann S, Dreyhaupt J, et al. Fundus autofluorescence and fundus perimetry in the junctional zone of geographic atrophy in patients with age-related macular degeneration. Invest Ophthalmol Vis Sci. 2004;45:4470-4476.
4. Schmitz-Valckenberg S, Fleckenstein M, Helb HM, et al. In vivo imaging of foveal sparing in geographic atrophy secondary to age-related macular degeneration. Invest Ophthalmol Vis Sci. 2009;50:3915-3921.
5. Holz FG, Bindewald-Wittich A, Fleckenstein M, Dreyhaupt J, Scholl HP, Schmitz-Valckenberg S; FAM Study Group. Progression of geographic atrophy and impact of fundus autofluorescence patterns in age-related macular degeneration. Am J Ophthalmol. 2007;143:463-472.
6. Schmitz-Valckenberg S, Fleckenstein M, Scholl HP, et al. Fundus autofluorescence and progression of age-related macular degeneration. Surv Ophthalmol. 2009;54:96-117.
7. Sparrow JR, Zhou J, Ben-Shabat S, Vollmer H, Itagaki Y, Nakanishi K. Involvement of oxidative mechanisms in blue-light-induced damage to A2E-laden RPE. Invest Ophthalmol Vis Sci. 2002;43:1222-1227.
8. Lamb LE, Simon JD. A2E: a component of ocular lipofuscin. Photochem Photobiol. 2004;79:127-136.
9. Finnemann SC, Leung LW, Rodriguez-Boulan E. The lipofuscin component A2E selectively inhibits phagolysosomal degradation of photoreceptor phospholipid by the retinal pigment epithelium. Proc Natl Acad Sci U S A. 2002;99:3842-3847.
10. Bermann M, Schutt F, Holz FG, Kopitz J. Does A2E, a retinoid component of lipofuscin and inhibitor of lysosomal degradative functions, directly affect the activity of lysosomal hydrolases? Exp Eye Res. 2001;72:191-195.
11. Suter M, Remé C, Grimm C, et al. Age-related macular degeneration. The lipofusion component N-retinyl-N-retinylidene ethanolamine detaches proapoptotic proteins from mitochondria and induces apoptosis in mammalian retinal pigment epithelial cells. J Biol Chem. 2000;275:39625-39630.
12. Radu RA, Hu J, Yuan Q, et al. Complement system dysregulation and inflammation in the retinal pigment epithelium of a mouse model for Stargardt macular degeneration. J Biol Chem. 2011;286:18593-18601.
13. Iriyama A, Inoue Y, Takahashi H, Tamaki Y, Jang WD, Yanagi Y. A2E, a component of lipofuscin, is pro-angiogenic in vivo. J Cell Physiol. 2009;220(2):469-75.
14. Sparrow JR, Fishkin N, Zhou J, et al. A2E, a by product of the visual cycle. Vision Res. 2003;43(28):2983-90.
15. Radu RA, Mata NL, Nusinowitz S, Liu X, Sieving PA, Travis GH. Treatment with isotretinoin inhibits lipofuscin accumulation in a mouse model of recessive Stargardt’s macular degeneration. Proc Natl Acad Sci U S A. 2003;100:4742-4747.
16. Golczak M, Kuksa V, Maeda T, Moise AR, Palczewski K. Positively charged retinoids are potent and selective inhibitors of the trans-cis isomerization in the retinoid (visual) cycle. Proc Natl Acad Sci U S A. 2005;102:8162-8167.
17. Maeda A, Maeda T, Golczak M, et al. Effects of potent inhibitors of the retinoid cycle on visual function and photoreceptor protection from light damage in mice. Mol Pharmacol. 2006;70:1220-1209.
18. Golczak M, Maeda A, Bereta G, et al. Metabolic basis of visual cycle inhibition by retinoid and nonretinoid compounds in the vertebrate retina. J Biol Chem. 2008;283:9543-9554.
19. Sparrow JR, Boulton M. RPE lipofuscin and its role in retinal pathobiology. Exp Eye Res. 2005;80:595-606.
20. Hinterhuber G, Cauza K, Brugger K, et al. RPE65 of retinal pigment epithelium, a putative receptor molecule for plasma retinol-binding protein, is expressed in human keratinocytes. J Invest Dermatol. 2004;122:406-413.
21. Znoiko SL, Crouch RK, Moiseyev G, Ma JX. Identification of the RPE65 protein in mammalian cone photoreceptors. Invest Ophthalmol Vis Sci. 2002;43:1604-1609.
22. Ma J, Xu L, Othersen DK, Redmond TM, Crouch RK. Cloning and localization of RPE65 mRNA in salamander cone photoreceptor cells1. Biochim Biophys Acta. 1998;1443:255-261.
23. Tang PH, Buhusi MC, Ma JX, Crouch RK. RPE65 is present in human green/red cones and promotes photopigment regeneration in an in vitro cone cell model. J Neurosci. 2011;31:18618-18626.
24. Kubota R, Boman NL, David R, Mallikaarjun S, Patil S, Birch D. Safety and effect on rod function of ACU-4429, a novel small-molecule visual cycle modulator. Retina. 2012;32:183-188.