Peripapillary Changes In Pathologic Myopia
Swept-source OCT can help image changes in high myopia.
Kyoko Ohno-Matsui, MD, PhD
Highly myopic eyes, also referred to as eyes with pathologic myopia, have characteristic morphologic changes in the optic nerve head (ONH), including scleral crescents, tilted optic discs, and secondary or acquired macrodiscs.1-7 These characteristics appear to arise mainly from a mechanical expansion of the papillary and peripapillary regions.
Visual field defects that are not explained by myopic fundus lesions are not uncommon in highly myopic patients. We recently showed that significant visual field defects, defined as a decrease of ≥10% of the outermost V4 isopter, were present in 13.2% of highly myopic eyes.8
However, the mechanism by which VF defects develop has not been clarified; nor have the kinds of structural abnormalities that occur on and around the optic nerve (ON) in highly myopic eyes related to VF defects. Unfortunately, it has been difficult to observe the deeper tissues within the ONH or posterior to the lamina cribrosa using conventional spectral domain optical coherence tomography (OCT), and the number of histopathologic studies of the ONH in human cadaver eyes is limited.3,9,10
SWEPT-SOURCE OCT
Swept-source OCT uses a frequency swept laser as a light source.11 In practice, it has less roll-off of sensitivity with tissue depth than conventional SD-OCT. The current swept-source OCT instruments also use longer wavelengths, generally in the 1-µm range, which has improved their ability to penetrate deeper into tissues than conventional SD-OCT instruments.12,13
With the capability to image deeper anatomic structures in the eye with swept-source OCT, evaluations of posterior structures are potentially possible. Particularly in highly myopic eyes with thin choroids, the entire thickness of the sclera can be observed. In severely myopic eyes, even the orbital fat tissue can be observed.
Thus, by using swept-source OCT, we have analyzed the papillary and peripapillary region of eyes with pathologic myopia.
OCT FINDINGS IN PATHOLOGIC MYOPIA
Peripapillary Intrachoroidal Cavitation
A peripapillary intrachoroidal cavitation (ICC) is a yellowish-orange lesion located inferior to the optic nerve in highly myopic eyes (Figure 1).14-20 Usually, in eyes with ICCs, the optic disc is tilted. This condition was originally reported to be peripapillary detachment of pathologic myopia (PDPM).15 However, later studies, using more recent versions of OCT instruments, have shown that this was not a detachment of the retinal pigment epithelium but an ICC.18
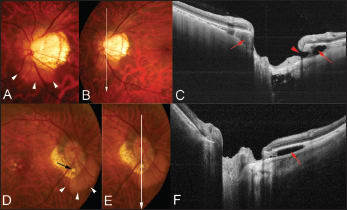
Figure 1. Peripapillary intrachoroidal cavitation accompanying by an inner retinal defect. A. Color fundus photograph showing a tilted optic disc and temporal conus. A yellowish-orange lesion suggestive of ICC is observed below the optic disc (arrowheads). B. A scanned line by swept-source OCT is indicated as a line with an arrow. C. OCT slice scanned along the line in B shows ICC (arrows) above and below the optic nerve. Hyporeflective space suggesting an existence of fluid is observed within the ICC below the optic nerve. An inner retinal defect (arrowhead) is observed along the margin of the ICC. D. Color fundus photograph showing a tilted optic disc and temporal conus. A yellowish-orange lesion suggestive of ICC is observed below the optic disc (arrowheads). An inner retinal defect is seen (arrow), and the retinal vein is bent toward the ICC cavity through this defect. E. A scanned line by swept-source OCT is indicated as a line with an arrow. F. OCT slice scanned along the line in E shows an ICC (arrows) below the optic nerve. Hyporeflective space suggesting the existence of fluid is observed within the ICC.
We have reported that peripapillary ICCs were observed in 5% (32/631) of eyes with pathologic myopia, and 71% of the eyes with peripapillary ICCs had glaucomatous VF defects.16
Using enhanced depth imaging (EDI)-OCT21 and swept-source OCT, Spaide14 and we16,17 found full-thickness defects in the retina at the interior border of the conus in some eyes. By looking deep into the scleral level, Spaide found that the sclera was bowed posteriorly under the region of the ICC, while the overlying retina-RPE-Bruch's membrane complex showed little, if any, deformation.14
In eyes with no inner retinal defect at the inferior border of the tilted optic disc, there was triangular thickening of the choroid at the base, near the border of the optic nerve (Figure 2). The base of this triangular choroidal thickening seemed to be associated with stretching of the region between the choroid and the optic nerve, a location where the bordering tissue of Jacoby is originally seen (Figure 2).
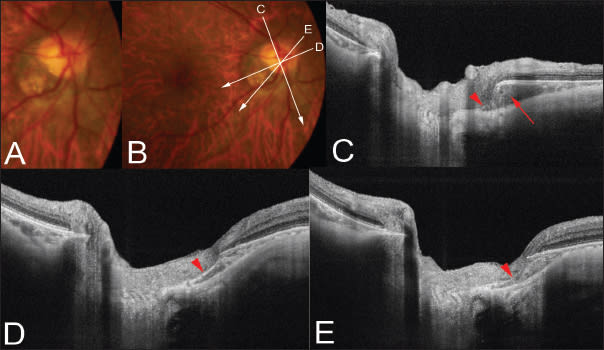
Figure 2. Peripapillary intrachoroidal cavitation. A. Color fundus photograph showing a tilted optic disc and temporal conus. A yellowish-orange lesion suggestive of ICC is not obvious in this patient. B. Scanned lines by swept-source OCT are indicated as lines with arrows. C. OCT slice scanned along the line in B shows the ICC (arrow) as thickening of the choroid below the optic nerve. The remnants of the border tissue of Jacoby are observed (arrowhead). D. OCT slice scanned along the line in B shows the ICC as a stretching of the border of the tissue of Jacoby (arrowhead). E. The stretched border of the tissue of Jacoby seems to be disrupted in this section (arrowhead).
Furthermore, in more severe cases, a defect in the bordering tissue of Jacoby was seen (Figure 2). The fluid space was observed within the ICC, and there was direct communication between vitreous cavity and the ICC through the inner retinal defect at ICC margin (Figure 1).
Spaide suggested that although the IOP is uniformly distributed throughout the eye, the deformation of the posterior wall of the fundus would be more pronounced in the area of the conus because of the thinness or a lack of overlying layers, compared with more normal regions temporal to the conus.14 Further, such pronounced deformations are considered to cause defects in the border of the tissue of Jacoby.
Like retinal detachments caused by congenital optic pits, we reported a case in which macular retinal detachment developed, accompanied by an ICC.22 An OCT examination showed that there was a full-thickness defect in the retina overlying the ICC, the vitreous cavity was connected to the ICC through this defect, and the ICC was continuous with the retinal detachment through the subretinal path at the conus area.
Thus, peripapillary ICCs not only cause VF defects, but they also cause macular retinal detachment, impairing the central vision.
Enlargement of SAS and Communication Between the Vitreous and SAS
By histomorphological analyses of human cadaver eyes, Jonas found that in highly myopic eyes, the peripapillary region consisted of an elongated peripapillary scleral flange (sclera between the optic nerve margin and dura) associated with scleral flange thinning and a retrobulbar cerebrospinal fluid space extending into the retroparapapillary region.23 However, it has been impossible to visualize the perioptic SAS in situ in human patients.
Using EDI-OCT, Park and colleagues recently showed that the subarachnoid space (SAS) around the optic nerve was identified with varying degrees of clarity in 25 of 139 eyes (18%). In these 25 eyes, 17 had high myopia and extensive PPA.24
We used swept-source OCT and showed that the periocular SAS could be observed in 124 of 133 eyes (93%) with pathologic myopia (the average axial length was 30.4 ±1.8 mm).25 The optic SAS appeared to be dilated in highly myopic eyes. The SAS was triangular, with the base toward the eye surrounding the optic nerve in the region of the scleral flange (Figure 3).
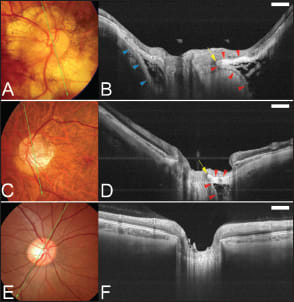
Figure 3. Visualization of the subarachnoid space (SAS) around the optic nerve in eyes with pathologic myopia. A. Color fundus photograph showing an optic disc with a large annular crescent. B. Swept-source OCT slice scanned along the green line in A shows the SAS as a hyporeflective space along both the upper and lower borders of the ON. The SAS is triangular, with the base toward the eye. The peripapillary sclera is continuous with the pia mater along the inner boundary of the SAS (red arrowheads). The SAS protrudes toward the ON at the transition point of the peripapillary sclera and the pia mater (arrow). The peripapillary sclera is also continuous with the dura mater along the outer boundary of the SAS (blue arrowheads). The arachnoid trabeculae are seen as wide, linear streaks within the SAS. C. Fundus photograph showing an optic disc with a temporal conus. A yellowish lesion indicating an intrachoroidal cavitation can be seen inferior to the conus. D. Swept-source OCT slice scanned along the green line in C shows the SAS as a triangular hyporeflective space with the base directed toward the eye. The peripapillary sclera is continuous with the pia mater along the inner boundary of the SAS (red arrowheads). The SAS protrudes toward the ON at the transition point of the peripapillary sclera and the pia mater (arrow). The arachnoid trabeculae have a reticular pattern inside the SAS. E. Fundus photograph of an emmetropic eye. F. Swept-source OCT slice scanned along the green line in E does not show any space indicating the SAS along the ON. Scale bar, 1 mm.
On B-scan images, the arachnoid trabeculae inside the SAS were clearly observed as a pattern of reticular lines and dots interspersed with hyporeflective zones consistent with fluid (Figure 3). The sclera on the peripapillary SAS was thinned, and the thickness of the thinnest region of peripapillary sclera above the SAS (scleral flange thickness) was 190.6 ±51.2 µm. In one myopic patient, there appeared to be direct communication between the intraocular cavity and the SAS through pit-like pores (Figure 4).
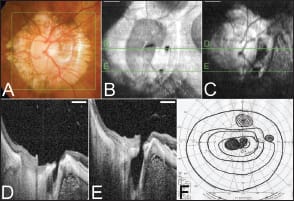
Figure 4. Direct communication between the intraocular space and the subarachnoid space. A. Color fundus photograph of the optic disc showing a large annular conus. B, C. En face cross-sectional images scanned in the area shown as a green square in A at different levels, showing that there are two pit-like pores temporal to the optic disc (B) and that these pores are continuous with the SAS at a deeper level (C). D, E. B-scan images acquired by 3D scan protocol showing communication between the intraocular space and the SAS temporal to the optic disc within the area of the myopic conus. F. Visual fields from Goldmann perimetry show a central and a paracentral scotoma in addition to the nasal step. Scale bar, 1 mm.
What kinds of effect would the enlargement of the perioptic SAS in a highly myopic eye have? Pressure is equal to force per unit area, and as such, the force load on the lamina is increased in proportion to the enlargement of the surface area exposed. The lamina itself may be less likely to resist deformation because of thinning.
Thus, the expanded area of exposure to cerebrospinal pressure, along with thinning of the posterior eye wall, may increase the pressure load on the peripapillary sclera, as well as on the lamina cribrosa. This force has the potential to increase the glaucoma susceptibility in highly myopic eyes.
ACQUIRED PITS IN THE OPTIC DISC AREA AND THE CONUS AREA
Optic nerve pits are common in highly myopic eyes. Examination of our swept-source OCT images showed that optic disc pits or conus pits were present in 32 of 198 highly myopic eyes (16.2%).26 The pits were located in the optic disc area (optic disc pits) in 11 of 32 eyes (Figure 5) and in the conus area (conus pits) in 22 of 32 eyes (Figure 6).
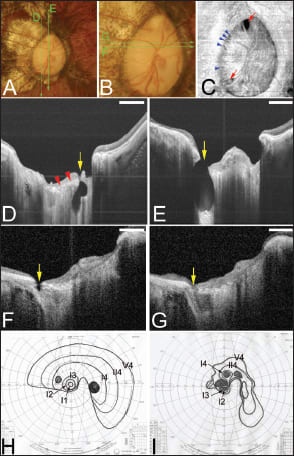
Figure 5. Deeply excavated pit-like structures within the optic disc area. Bar, 1 mm. A. Right fundus of a 45-year-old woman shows an oval optic disc and a temporal conus. The refractive error was ·18.0 D, and the axial length was 29.4 mm. The optic disc area was 30.5 mm2 based on the axial length. The green lines are the scanned lines of the swept-source OCT images shown in D and E. B. Magnified view of the optic disc shows a large optic disc with saucer-like cupping. The green lines are the scanned lines of the swept-source OCT images shown in F and G. C. En face view of the optic disc reconstructed from 3D images of swept-source OCT shows two large pits at the upper and lower poles of the optic disc (arrows). These pits have a triangular shape, and their bases are directed to the edge of the optic disc. Multiple pit-like structures are also observed along the temporal margin of the optic disc (arrowheads). D. B-scan swept-source OCT image of the scanned line shown in A shows a deep pit (arrow) in the inferior pole of the disc extending posteriorly beyond the lamina cribrosa. The inner surface of the lamina is indicated by arrowheads. The nerve fiber overlying the pit is disrupted. E. B-scan swept-source OCT image of the scanned line shown in A shows an ovalshaped, deeply excavated pit (arrow) at the superior pole of the optic disc with a wide opening. The lamina is torn off from the peripapillary sclera at the site of the pit, and the nerve fibers overlying the pit are discontinuous at the site of the pit. The depth of the pit from its opening is 1,142 µm. F. B-scan swept-source OCT image across the scanned line shown in B shows a shallow pit (arrow) along the temporal margin of the optic disc. G. Section adjacent to that shown in F shows a discontinuity of the lamina and peripapillary sclera at the junction site (arrow). A hyporeflective space is observed posterior to the junction site. H and I. Visual fields from Goldmann perimetry at the initial examination (H) and 10 years later (I) show that the defects are progressive. Scale bars, 1 mm.
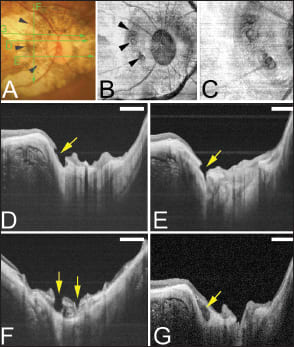
Figure 6. Conus pits present on the temporal side of the scleral ridge in an eye with type IX staphyloma (Curtin's classification). A. Photograph of right fundus of a 64-year-old woman shows an oval disc with a large annular conus. A scleral ridge is indicated by arrowheads. The axial length of this eye was 32.8 mm with an intraocular lens implanted. Bar, 1 mm. The green lines show the area scanned by swept-source OCT for the images shown in D through G. B. En face image of the optic disc area reconstructed from three-dimensional swept-source OCT images shows multiple pits on the slope inside the ridge at approximately same distance from the optic disc margin (arrowheads). C. Magnified view of B shows a collection of pits just temporal to the scleral ridge. D. B-scan swept-source OCT image shows that the pit is present on the inner slope of the ridge (arrow). The peripapillary sclera and overlying nerve fiber tissue are discontinuous on the pit site. E. Another pit can be seen inferotemporal to the optic disc. F. Vertical OCT scan temporal to the optic disc shows multiple pits (arrows), and the tissue around the pits looks like a sclera schisis. G. Swept-source OCT section adjacent to the pits shows a hyporeflective space resembling a scleral schisis (arrow).
The eyes with optic nerve pits were significantly more myopic and had longer axial length than highly myopic eyes without any kind of pits in the ON area. The continuity of the retinal nerve fiber layer was disrupted in the area overlying the ON pits (regardless of optic disc pits or conus pits).
Optic disc pits. The optic disc pits existed in the superior or inferior border of the optic disc. The openings of most of the pits were not circular but were rather triangular, with the base at the outer edge of the optic disc and the apex pointing toward the center of the disc (Figure 5).
The eyes with optic disc pits had significantly larger optic disc areas and conus areas than those without any type of ON pits. The average disc area of the eyes with optic disc pits was as large as 7.2 ±2.1 mm2. The depth of the optic disc pits varied from shallow, that is, the bottom of the pit located within the lamina cribrosa, to deeply excavated, sometimes with a depth of the pit >1,000 µm from the plane of the pit opening to the bottom.
Optic disc pits have been reported in other conditions, such as congenital optic disc pits15 and acquired pits in advanced glaucoma.27-30 However, the nerve head enlargement seen in eyes with congenital optic disc pits appeared different from secondary megalodiscs in highly myopic eyes. In the early stage of optic disc pits, the lamina appeared to be torn from the peripapillary sclera along the margin of the optic nerve (Figures 5F and 5G).
To explain how optic disc pits develop, we hypothesized that the optic disc is first enlarged by a mechanical expansion of the papillary region in highly myopic eyes and becomes megalodisc-like (Figure 7). This mechanical expansion of the optic disc area results in stretching and expanding of the lamina cribrosa.
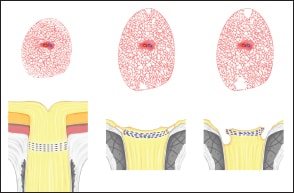
Figure 7. Hypothetical scheme showing how optic disc pits develop. Upper panels show the images of en face view of the optic disc and peripapillary area. Lower panels show the B-scan swept-source OCT images. The optic disc area is first enlarged by a mechanical expansion of the papillary region in highly myopic eyes and becomes megalodisc-like (upper middle image). After the mechanical expansion, the lamina cribrosa is also stretched and expanded. Because of the continuous mechanical expansion of the lamina and surrounding peripapillary sclera, the lamina is eventually torn from the peripapillary sclera at the junction site, especially at the superior and inferior poles of the optic disc. This stage is observed by swept source OCT as hyporeflective gap at the junction between the lamina cribrosa and the peripapillary sclera (lower middle mage). Later, with further increase of the distance of the gap, the nerve fiber overlying this gap is disrupted, and this stage is observed as optic disc pits by swept source OCT (lower right image).
Because of mechanical tension on the lamina and surrounding peripapillary sclera, with the myopic thinning of these structures, the lamina might dehisce from the peripapillary sclera, particularly at the superior and inferior poles, which are sites of decreased connective tissue support.31
This stage is observed by swept-source OCT as a hyporeflective gap at the junction of the lamina cribrosa and the peripapillary sclera. Further increases of the gap seemed to be associated with disruption of the overlying nerve fibers or herniation of these fibers into the defect.
Conus pits. Conus pits26 have never been reported. We showed that conus pits are observed in highly myopic eyes with type IX staphyloma as per Curtin.32 Type IX staphyloma is characterized by a protruding ridge formation temporal to the optic disc within a wide area of staphyloma.
The conus pits were usually multiple, and they developed nasal to the ridge and existed on the slope of the ridge (Figure 6). A condition resembling peripapillary scleral schisis was found in sections adjacent to these types of pits (Figure 6G).
We reported that posterior staphyloma not only deepens with age but also changes its shape.33 With increasing age, a transition from type I or II staphyloma to type IX staphyloma occurs by the development of a ridge-like protrusion temporal to the optic disc.33
The development of this ridge-like protrusion results in stretching of the peripapillary sclera nasal to the ridge and a condition similar to scleral schisis. Then, a disruption of the continuity of the superficial layer of the sclera in the area of a scleral schisis is considered to result in the formation of conus pits (Figure 8).
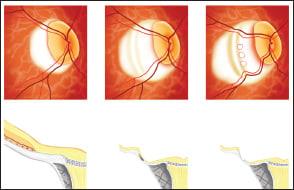
Figure 8. Hypothetical schema showing the formation of conus pits inside the ridge of an eye with type IX staphyloma. Upper panel shows the en face image of the optic disc and peripapillary area. Lower panel shows the B-scan swept source optical coherence tomographic (OCT) image. The secondary development of the ridge is due to a transition to type IX staphyloma with increasing age, and the peripapillary sclera inside the ridge is markedly stretched resulting in scleral schisis (lower middle image). Then, with further stretching of the peripapillary sclera, probably due to an increased depth of the staphyloma, disruption is finally caused in the continuity of the superficial layer of the sclera in the area of the scleral schisis, resulting in conus pit formation (lower right image).
In some other cases, the short posterior ciliary arteries (SPCAs) penetrate through the sclera in the area of the conus pits, suggesting that the emissary canal by which the SPCAs entered the globe has been enlarged by mechanical expansion of the peripapillary region.
Clinical Significance of Acquired Pits
The clinical importance of the pits probably lies in the disruption of the nerve fiber overlying the pits. In addition to the discontinuity of nerve fiber tissue overlying the pits, the lamina was dehisced from the peripapillary sclera at the site of optic disc pits.
Dehisced lamina cribrosa due to optic disc pits and discontinuous peripapillary sclera due to conus pits could cause a condition similar to that of the material of a trampoline with its springs broken.
FUTURE PERSPECTIVES
Although OCT technology has advanced greatly, the depth of the image is still limited, and the observation of the perioptic SAS was limited within the area of the myopic conus. Further modification of OCT technology should be undertaken so that we can observe the ON and surrounding structures of the ON more deeply and more clearly.
Also, how such papillary and peripapillary abnormalities develop and progress and how such abnormalities cause VF defects must be investigated in a long-term study. Also, to include fewer younger myopic children might be necessary to investigate early changes and their progression. Recently, Kim and colleagues34 reviewed the optic disc photographs of 118 eyes of 118 Korean children with incipient myopia and showed that tilted discs, as well as PPAs, which may be an acquired feature in myopic eyes, arose from scleral stretching.
Although many issues remain, the development of powerful tools such as swept-source OCT to visualize deeper ON structures has greatly improved the investigation of papillary and peripapillary structures in high myopia. Further studies are expected to clarify how these develop and progress and finally cause VF defects, which are a major cause of blindness in patients with pathologic myopia. RP
REFERENCES
1. Jonas JB, Gusek GC, Naumann GO. Optic disk morphometry in high myopia. Graefes Arch Clin Exp Ophthalmol. 1988;226:587-590.
2. Jonas JB, Dichtl A. Optic disc morphology in myopic primary open-angle glaucoma. Graefes Arch Clin Exp Ophthalmol. 1997;235:627-633.
3. Dichtl A, Jonas JB, Naumann GO. Histomorphometry of the optic disc in highly myopic eyes with absolute secondary angle closure glaucoma. Br J Ophthalmol. 1998;82:286-289.
4. Hwang YH, Yoo C, Kim YY. Myopic optic disc tilt and the characteristics of peripapillary retinal nerve fiber layer thickness measured by spectral-domain optical coherence tomography. J Glaucoma. 2012;21:394-400.
5. Xu L, Li Y, Wang S, Wang Y, Jonas JB. Characteristics of highly myopic eyes: the Beijing Eye Study. Ophthalmology. 2007;114:121-126.
6. Wang Y, Xu L, Zhang L, Yang H, Ma Y, Jonas JB. Optic disc size in a population based study in northern China: the Beijing Eye Study. Br J Ophthalmol. 2006;90:353-356.
7. Jonas JB. Optic disk size correlated with refractive error. Am J Ophthalmol. 2005;139:346-348.
8. Ohno-Matsui K, Shimada N, Yasuzumi K, et al. Long-term development of significant visual field defects in highly myopic eyes. Am J Ophthalmol. in press.
9. Jonas JB, Berenshtein E, Holbach L. Lamina cribrosa thickness and spatial relationships between intraocular space and cerebrospinal fluid space in highly myopic eyes. Invest Ophthalmol Vis Sci. 2004;45:2660-2665.
10. Ren R, Wang N, Li B, et al. Lamina cribrosa and peripapillary sclera histomorphometry in normal and advanced glaucomatous Chinese eyes with various axial length. Invest Ophthalmol Vis Sci. 2009;50:2175-2184.
11. Drexler W, Fujimoto JG, eds. Optical Coherence Tomography: Technology and Applications. New York, NY: Springer; 2008:359-378.
12. Unterhuber A, Povazay B, Hermann B, Sattmann H, Chavez-Pirson A, Drexler W. In vivo retinal optical coherence tomography at 1040 nm - enhanced penetration into the choroid. Opt Express. 2005;13:3252-3258.
13. Potsaid B, Baumann B, Huang D, et al. Ultrahigh speed 1050nm swept source/Fourier domain OCT retinal and anterior segment imaging at 100,000 to 400,000 axial scans per second. Opt Express. 2010;18:20029-20048.
14. Spaide RF, Akiba M, Ohno-Matsui K. Evaluation of peripapillary intrachoroidal cavitation with swept source and enhanced depth imaging optical coherence tomography. Retina. 2012;32:1037-1044.
15. Freund KB, Ciardella AP, Yannuzzi LA, et al. Peripapillary detachment in pathologic myopia. Arch Ophthalmol. 2003;121:197-204.
16. Shimada N, Ohno-Matsui K, Yoshida T, et al. Characteristics of peripapillary detachment in pathologic myopia. Arch Ophthalmol. 2006;124:46-52.
17. Shimada N, Ohno-Matsui K, Nishimuta A, Tokoro T, Mochizuki M. Peripapillary changes detected by optical coherence tomography in eyes with high myopia. Ophthalmology. 2007;114:2070-2076.
18. Toranzo J, Cohen SY, Erginay A, Gaudric A. Peripapillary intrachoroidal cavitation in myopia. Am J Ophthalmol. 2005;140:731-732.
19. Wei YH, Yang CM, Chen MS, Shih YF, Ho TC. Peripapillary intrachoroidal cavitation in high myopia: reappraisal. Eye (Lond). 2009;23:141-144.
20. Forte R, Pascotto F, Cennamo G, de Crecchio G. Evaluation of peripapillary detachment in pathologic myopia with en face optical coherence tomography. Eye. 2008;22:158-161.
21. Margolis R, Spaide RF. A pilot study of enhanced depth imaging optical coherence tomography of the choroid in normal eyes. Am J Ophthalmol. 2009;147:811-815.
22. Shimada N, Ohno-Matsui K, Iwanaga Y, Tokoro T, Mochizuki M. Macular retinal detachment associated with peripapillary detachment in pathologic myopia. Int Ophthalmol. 2009;29:99-102.
23. Jonas JB, Jonas SB, Jonas RA, Holbach L, Panda-Jonas S. Histology of the parapapillary region in high myopia. Am J Ophthalmol. 2011;152:1021-1029.
24. Park SC, De Moraes CG, Teng CC, Tello C, Liebmann JM, Ritch R. Enhanced depth imaging optical coherence tomography of deep optic nerve complex structures in glaucoma. Ophthalmology. 2011;119:3-9.
25. Ohno-Matsui K, Akiba M, Moriyama M, Ishibashi T, Tokoro T, Spaide RF. Imaging the retrobulbar subarachnoid space around the optic nerve by swept source optical coherence tomography in eyes with pathologic myopia. Invest Ophthalmol Vis Sci. 2011;52:9644-9650.
26. Ohno-Matsui K, Akiba M, Moriyama M, et al. Acquired optic nerve and peripapillary pits in pathologic myopia. Ophthalmology. in press.
27. Healey PR, Mitchell P. The prevalence of optic disc pits and their relationship to glaucoma. J Glaucoma. 2008;17:11-14.
28. Nduaguba C, Ugurlu S, Caprioli J. Acquired pits of the optic nerve in glaucoma: prevalence and associated visual field loss. Acta Ophthalmol Scand. 1998;76:273-277.
29. Radius RL, Maumenee AE, Green WR. Pit-like changes of the optic nerve head in open-angle glaucoma. Br J Ophthalmol. 1978;62:389-393.
30. Ugurlu S, Weitzman M, Nduaguba C, Caprioli J. Acquired pit of the optic nerve: a risk factor for progression of glaucoma. Am J Ophthalmol. 1998;125:457-464.
31. Dandona L, Quigley HA, Brown AE, Enger C. Quantitative regional structure of the normal human lamina cribrosa. A racial comparison. Arch Ophthalmol. 1990;108:393-398.
32. Curtin BJ. The posterior staphyloma of pathologic myopia. Trans Am Ophthalmol Soc. 1977;75:67-86.
33. Hsiang HW, Ohno-Matsui K, Shimada N, et al. Clinical characteristics of posterior staphyloma in eyes with pathologic myopia. Am J Ophthalmol. 2008;146:102-110.
34. Kim TW, Kim M, Weinreb RN, Woo SJ, Park KH, Hwang JM. Optic disc change with incipient myopia of childhood. Ophthalmology. 2012;119:21-26.
Kyoko Ohno-Matsui, MD, PhD, is an associate professor in the Department of Ophthalmology and Visual Science at Tokyo Medical and Dental University and chief of the High Myopia Clinic at Tokyo Medical and Dental University. She reports no financial interest in any products mentioned in this article. She expresses her sincere appreciation to Richard F. Spaide, MD, for his collaboration and suggestions. Dr. Ohno-Matsui can be reached via e-mail at k.ohno.oph@tmd.ac.jp. |