What OCT Reveals About the Fovea
Advances shed light on the clinical relevance of foveal development and architecture.
Ala Moshiri, MD, PhD
Ala Moshiri, MD, PhD, is on the faculty of the Wilmer Eye Institute of the the Johns Hopkins University Hospital in Baltimore. He reports no financial interest in any product mentioned in this article. Dr. Moshiri can be reached via e-mail at quddus@gmail.com. |
The fovea is a specialized retinal area that supports the highest visual acuity. A normal fovea is distinguished from the rest of the retina by a central area exclusively containing cones, with elongated outer segments underlying a capillary-free zone and surrounded by separation of inner retinal layers.1
The development of OCT has made the study of foveal morphology in humans possible without the acquisition of tissue. This technology allows data-gathering in vivo, which overcomes some of the limitations of histology. Unlike histology, OCT is not limited by the paucity of human samples, and with the more recent advent of scan registration and eye-tracking algorithms, the uncertainty of the location of the fixation point is no longer an issue.
BASIC FOVEAL ANATOMY AND DEVELOPMENT
Studies have demonstrated substantial interindividual variations in foveal shape and thickness in the normal population.2,3 It has been shown that people of African descent have thinner foveae than do Caucasians.4 In addition, women have thinner foveae than men.5 Several conditions feature an absence of the foveal depression in some but not all cases, namely albinism,6 aniridia and retinopathy of prematurity.7
It could be argued that the fovea is the most important square millimeter of tissue in the human body. Its appearance in the history of evolution was one of the most important moments in vertebrate history. Yet little is known about the mechanism of foveal development, because few species used for genetic studies have this specialization of the retina.
What is known is based largely on histological studies in primates and computer modeling.8-10 The results of that body of literature suggest that many factors are at play. Early in foveal development, the foveal avascular zone (FAZ) is formed. Then, inner retinal layers move centrifugally out of the FAZ.
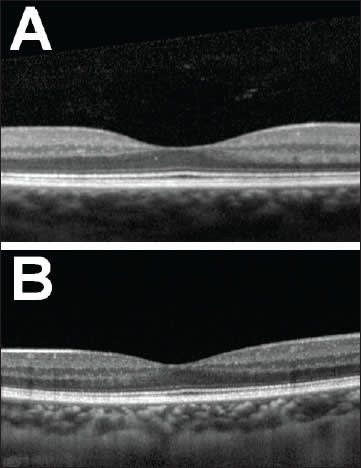
Figure 1. Two normal SD-OCTs through the fovea at the point of fixation are shown from healthy young adults with normal visual acuity. The configuration in (A) demonstrates a more common appearance with a wide and deep foveal pit and with an obvious area of absent inner nuclear layer. Image (B) shows a less common normal variant, with a shallower and narrower pit and less distance between the inner nuclear layer on either side. Much broader variability of normal foveae exists than depicted in this figure. The clinical significance, if any, of the various configurations remains to be elucidated.
At the same time, the outer retina (specifically cone photoreceptors) migrates centripetally inward toward the foveal center, and their outer segments elongate. Finally, as intraocular pressure rises, and the axial length increases with normal eye growth, these mechanical forces may lead the way to foveal pit–deepening within the FAZ.
The relationship between fundus pigmentation and foveal development is an area of active research. People with oculocutaneous albinism lack fundus pigmentation and also exhibit foveal hypoplasia and an absent FAZ, in addition to other ocular abnormalities. There is a correlation between increased fundus pigmentation and less profound foveal hypoplasia in albinos, as well as improved visual functioning. This is the basis of research aimed at increasing fundus pigmentation as a therapy in the clinical setting of albinism.
Similarly, there is a known relationship between increased fundus pigmentation (in people of African descent) and relatively deep foveal pits and correspondingly thin central foveal thickness.4 Taken together, these data suggest that with more fundus pigmentation, there is a progressively more mature foveal structure. Similarly, with less fundus pigmentation, the fovea appears less mature and even hypoplastic on the spectrum of foveal maturation.
The relationship between fundus pigmentation in the retinal pigment epithelium and foveal development remains poorly understood, and future research in this area will clarify both the genetic mechanism and the clinical relevance of the diversity of foveal phenotypes.
OCT STUDIES
Recently, Tick et al. published a study examining foveal shape and structure in a normal population.11 They examined 110 eyes of 57 healthy adults, noting foveal shape and structure by automated segmentation of retinal layers on high-resolution OCT. A subset of eyes underwent simultaneous fluorescein angiography, and in these patients, the size of the FAZ was correlated with foveal shape on OCT. In this normal population, the authors discovered a diverse range of foveal shapes and sizes. They found a structural continuum ranging from a relatively modest and shallow pit, with continuity of the inner nuclear layer over the center of the fovea, to a well-excavated, flat pit with a thinner central outer nuclear layer and complete separation of inner layers overlying it.
In their analyses, central foveal thickness was correlated inversely with the degree of inner layer separation and with the surface of the FAZ. Foveal structure strongly correlates with its neurovascular organization, suggesting a developmental model in which the size of the FAZ determines the extent of centrifugal migration of inner retinal layers and the centripetal packing of cone photoreceptors.
FOVEAL PATHOLOGY
The fovea is the site of pathology in many retinal diseases. Some of the mechanisms of disease can be mechanical. The vitreoretinal interface at the fovea can prove to be abnormal. This relationship can lead to anteroposterior traction, causing blunting of the foveal contour and subsequent visual distortion.
If the vitreoretinal interface causes tangential traction, a full-thickness macular hole may develop, resulting in an absolute visual scotoma in the affected eye. Other macular disease can be a result of edema due to retinal vascular disease. For instance, retinal vein occlusion can lead to incompetent retinal microvasculature and accumulation of fluid in the macula. Similarly, diabetes can result in microvascular abnormalities resulting in macular edema.
Uveitis and postsurgical inflammation can produce a similar picture. Macular telangiectasis is a heterogeneous group of likely congenital and often progressive microvascular abnormalities of FAZ development. AMD, the leading cause of blindness in the Western world, may be a result of oxidative stress on foveal tissues, leading to atrophy and/or neovascular processes that adversely affect vision.
All of these conditions and many others affect the fovea. However, the details of the pathophysiology of disease in each case are at best only moderately understood. Further characterization and categorization of the diverse normal foveal morphologies may clarify which foveae are susceptible to macular edema after cataract surgery, DME, or the development of full-thickness macular holes. Therefore, there may be a predictive role for OCT of the macula in diabetic patients, which may foretell the development of DME.
Similarly, there may be a predictive role not only for the formation of macular holes but also for the potential visual recovery after treatment for macular hole repair. One can imagine that foveal structure may predict responders to anti-VEGF medications and, more importantly, may predict who might be a so-called nonresponder.
THE ROLE OF GENETICS
Genetics controls anatomy, which makes anatomy a strong corollary to nature in the nature vs nurture argument when it comes to the pathophysiology of macular disease. Put an other way, the genetic basis of disease is widely accepted. Perhaps in the context of retinal diseases, the physician might take advantage of extremely precise interrogations of anatomy and morphology, which may represent the genetics of the subject closely enough to predict disease development and progression, especially in pathologies with a largely mechanical component.
Further studies of these phenomena in larger numbers of normal people — across distinctions in race, gender and age — need to be done. Prospective follow-up in large groups will need to be undertaken to analyze the foveal structures prior to the development of pathology, to draw any meaningful conclusions with regard to normal foveal anatomy predisposing certain people to certain foveal pathologies later in life.
High-resolution OCT, adaptive optics, microperimetry and likely other technologies yet to be developed will need to be employed. Outcomes measurements for clinical trials will need to be developed for each of these modalities. Non invasive ultrahigh-resolution evaluation of retinal vasculature, using optical microangiography technology, will likely be employed, in conjunction with the now ubiquitous cross-sectional modalities as part of the same scan.12
One can imagine a day in which a single scan perform ed by a single multifunctional OCT done in a normal patient — which may not only produce a cross-sectional image but also produce en-face imaging of foveal blood flow and vasculature analogous to an angiogram — may combine this information to provide a risk calculation based on empirical data: eg, 1% risk of macular hole, 60% risk of severe AMD, 30% risk of pseudophakic macular edema.
CONCLUSION
Given the rarity of most foveal conditions, tremendous numbers of people would need to be followed prospectively and probably for a very long time, as they transition from no pathology to moderate pathology to severe pathology. Nevertheless, the hypothesis that the diversity of normal foveal developmental subtypes may underlie the diversity of phenotypes seen in various macular diseases is not difficult to believe, although it remains to be proved. RP
REFERENCES
1. Hendrickson AE. Organization of the adult primate fovea. In: Penfold PL, Provis GM, eds. Macular Degeneration. Heidelberg, Germany: Springer Verlag; 2005:1–20.
2. Ishikawa H, Stein DM, Wollstein G, et al. Macular segmentation with optical coherence tomography. Invest Ophthalmol Vis Sci. 2005; 46:2012–2017.
3. Lim MC, Hoh ST, Foster PJ, et al. Use of optical coherence tomography to assess variations in macular retinal thickness in myopia. Invest Ophthalmol Vis Sci. 2005; 6:974–978.
4. Asefzadeh B, Cavallerano AA, Fisch BM. Racial differences in macular thickness in healthy eyes. Optom Vis Sci. 2007; 84:941–945.
5. Kelty PJ, Payne JF, Trivedi RH, et al. Macular thickness assessment in healthy eyes based on ethnicity using Stratus OCT optical coherence tomography. Invest Ophthalmol Vis Sci. 2008; 49:2668–2672.
6. McAllister JT, Dubis AM, Tait DM, et al. Arrested development: high-resolution imaging of foveal morphology in albinism. Vision Res. 2010; 50:810–817.
7. Recchia FM, Recchia CC. Foveal dysplasia evident by optical coherence tomography in patients with a history of retinopathy of prematurity. Retina. 2007; 27:1221–1226.
8. Springer AD, Hendrickson AE. Development of the primate area of high acuity. Use of finite-element analysis models to identify mechanical variables affecting pit formation. Vis Neurosci. 2004; 21:53–62.
9. Springer AD, Hendrickson AE. Development of the primate area of high acuity. 2. Quantitative morphological changes associated with retina and pars plana growth. Vis Neurosci. 2004; 21:775–790
10. Springer AD, Hendrickson AE. Development of the primate area of high acuity, 3: temporal relationships between pit formation, retinal elongation and cone packing. Vis Neurosci. 2005; 2:171–185.
11. Tick S, Rossant F, Ghorbel I, Gaudric A, Sahel JA, Chaumet-Riffaud P, Paques M. Foveal shape and structure in a normal population. Invest Ophthalmol Vis Sci. 2011 Jul 29;52(8):5105-5110.
12. Wang RK, An L. Multifunctional imaging of human retina and choroid with 1050-nm spectral domain optical coherence tomography at 92-kHz line scan rate. J Biomed Opt. 2011 May; 16(5):050503.