The Ever-Evolving Role of Imaging in DME Management
We have seen continuous improvement as new technologies emerge.
Marcel Menke, MD • Ceklic Lala, MD • Carsten Framme, MD • Sebastian Wolf, MD
Marcel Menke, MD, is on the faculty of the Universitätsklinik für Augenheilkunde of the University of Bern in Switzerland. Ceklic Lala, MD, is on the faculty of the University of Bern and of the Eye Clinic of the Clinical Center of Eastern Sarajevo in Bosnia and Herzegovina. Carsten Framme, MD, is on the faculty of the University of Bern. Sebastian Wolf, MD, is on the faculty of the University of Bern and of the university's Photographic Reading Center. None of the authors reports any financial interests in any products mentioned in this article. Dr. Wolf can be reached via e-mail at Sebastian.Wolf@insel.ch. |
Recently published data indicate that diabetic retinopathy (DR) is the leading cause of new blindness in patients aged 25-74 years old in the United States. The estimated prevalence of DR in the United States is up to 28.5% among patients with diabetes aged 40 years and older.1 Vascular endothelial damage is caused in diabetic macular edema by chronic hyperglycemia, hypoxia and low-grade inflammation.3-8 Considering that DME is a multifactorial disease, modern and standard treatment options include laser treatment, anti-VEGF and corticosteroid therapy.4 Due to the socioeconomic burden of DME, reliable screening methods and early disease detection and treatment became major research focuses, triggering the development of various diagnostic methods and tools.9
THE BEGINNING: FUNDUS PHOTOGRAPHY AND FLUORESCEIN ANGIOGRAPHY
The evolution of imaging for DME began with standard procedures, such as dilated stereoscopic fundus examination and stereoscopic fundus photography. The first photographic fundus images were obtained by the end of the 19th and the beginning of the 20th centuries. In addition, the concept of a fundus camera system dates back to that time.10 These achievements represented the keystones for fundus documentation in DR and DME. Even today, the importance of photographic fundus documentation in diabetes cannot be overemphasized.
Down the timeline, continuous efforts have been made to improve fundus imaging.11 The introduction of digital fundus imaging led to several advantages over analog photography that had an impact on DR screening and DME documentation. Among these advantages were the speed and ease of data access,12 immediate access to the results, and fast and precise duplication, archiving and transmission of data.13-15 With digital photography, it became possible to repeat the imaging procedures immediately in cases of bad image quality.14,15
All these features allowed for time-saving and cost-effective diabetes screening. Furthermore, telemedicine projects in diabetes became possible because of improved digitalization and data transfer protocols.16 Today, the development of wide-angle, nonmydriatic fundus imaging systems allow for easy and fast fundus documentation, without the need of a retina specialist. Images can be reviewed in special reading centers, and results can be transferred back to general physicians to plan further steps, if required (Figure 1).17
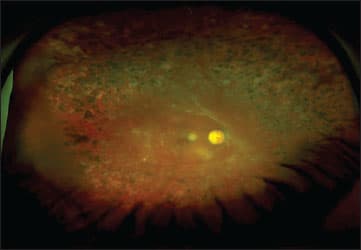
Figure 1. A patient with proliferative diabetic retinopathy and panretinal laser photocoagulation viewed with an ultra-widefield Optomap fundus photo.
The Early Treatment of Diabetic Retinopathy Study provided recommendations for the definition and classification of and treatment approach to DME. Those recommendations are still considered the gold standard in clinical practice worldwide. The ETDRS was based on stereoscopic fundus photography evaluation. Dilated stereoscopic fundus examination by experienced examiners is highly sensitive and specific, but for a more precise assessment of DME, it provides only limited information.4,18 In particular, substructural retinal changes or altered retinal circulation due to DR and DME cannot be observed by stereoscopic fundus biomicroscopy.
Fluorescein angiography is very useful in the management of DME as it provides information on retinal circulation and the blood-retinal barrier. FA combined with high-speed confocal scanning laser ophthalmoscopy brought new insights into the onset and progression of DR.19-25 In FA, a light beam of appropriate wavelength is used to excite sodium fluorescein molecules that emit light at certain wavelengths. The peaks wavelengths of absorption and emission are 490 and 520 nm or 490 and 510 nm,26 respectively. FA is helpful in detecting leakage and ischemia prior to treatment but it is an invasive procedure. The application of fluorescein has a risk of serious side effects that could be even fatal for patients in rare conditions.27-29
THE ERA OF OPTICAL COHERENCE TOMOGRAPHY
In 1991, Huang and coworkers published research on a new imaging technique called time-domain optical coherence tomography.30 The TD-OCT technique is based on the degree of absorption and dispersion of light traversing tissue. The light emitted by a superluminescent diode at a wavelength of approximately 840 nm is split in two arms: detection and reference. Comparing a reflected beam of light to a beam of light from a reference mirror, this technology measures time delay between those two beams.
With this method, it became possible to obtain B-scan cross-sections every 1.6 seconds (400 A-scans per second).31 The first two commercially available TD-OCTs were only available at few institutions. It was not until the commercially available Stratus OCT 3000 (Carl Zeiss Meditec) that OCT imaging became accessible to a large number of retina specialists.
In 1995, Hee et al. published first OCT data on quantitative assessment of macular edema.32 Since then, OCT has become the superior method for early DME detection and for monitoring disease progress and response to treatment. With increased OCT image resolution, enormous progress has been made in the differentiation of retinal layers, measuring retinal thickness, and evaluating substructural changes caused by DME.
Spectral-domain (SD)-OCT, invented in recent years, has superior depth resolution compared to conventional TD-OCT. The current axial image resolution ranges from 3-17 µm, depending on the OCT model.33 This technology uses a spectrometer on the detector arm to measure the differences in wavelength between the light coming from the fixed reference arm and light returning from tissue. The instrument uses spectrometric analyses to compute images according to the light-wavelength record. Thus, avoiding movements of the reference arm (using a spectrometer instead) increases the scanning speed significantly.
The higher speed allows up to 40,000 A-scans per second.34 Retinal scan coverage was increased, and three-dimensional macular imaging became available. In addition, the risk of artifacts due to eye movements and poor fixation stability of low-vision patients was reduced.35
Due to its increased axial resolution and increased retinal scan coverage, SD-OCT revealed DME-associated alterations of the photoreceptors, external limiting membrane, retinal pigment epithelium layer, and Bruch's membrane. In addition, SD-OCT helped to identify associated epiretinal membranes, changes in the vitreoretinal interface and retinal atrophy. Further, SD-OCT helped to distinguish between diffuse, focal and cystoid macular edema (Figure 2).14-17,31-36
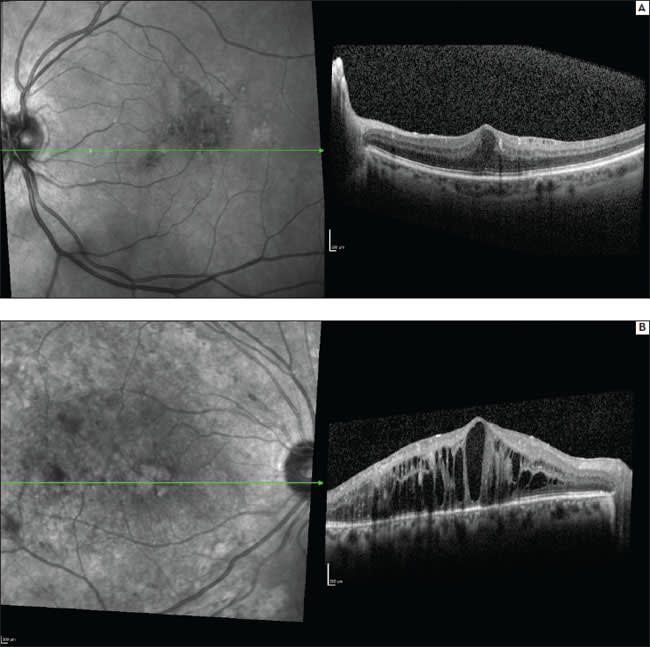
Figure 2. Spectralis OCT+HRA images of focal (a) and cystoid DME (b).
Currently, there are various SD-OCT instruments available: Cirrus HD-OCT (Carl Zeiss Meditec), RTVue-Fourier Domain OCT (Optovue), Copernicus OCT (Reichert/Optopol Technology), Spectral OCT/SLO (Opko/Oti), Spectralis HRA+OCT (Heidelberg Engineering), Topcon 3D OCT-1000 (Topcon) and RS-3000 Retiscan (Nidek). All of these instruments provide high-quality OCT images and three-dimensional macular reconstructions.33 Information obtained from retinal thickness maps and retinal profiles are valuable for exact objective and numerical quantification of improvement or progression of disease during follow-up (Figure 3).
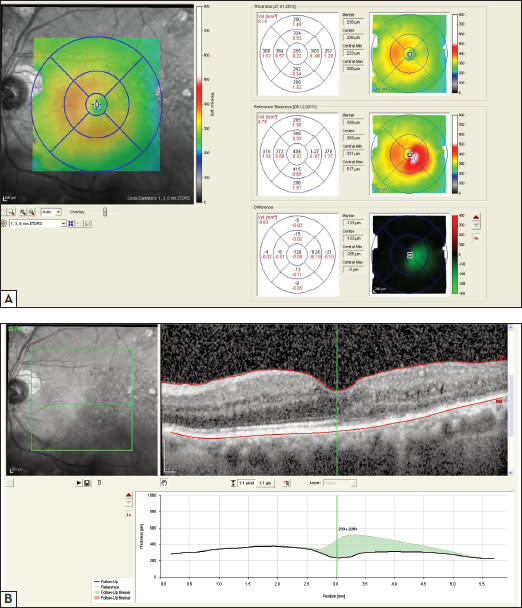
Figure 3. (a) Spectralis OCT retinal thickness map shows improvement of diabetic macular edema after treatment with ranibizumab. (b) In the retinal thickness profile, resolution of diabetic macular edema is marked in green (graph).
With improved OCT image resolution, more and more structural retinal changes have been identified that are associated with DME and that correlate with visual function. SDOCT technology has shown a relationship between cystoid spaces in the outer plexiform layer and photoreceptor damage beneath the cystoid spaces in patients with DME.33-36
Furthermore, recent published data have correlated fluorescein pooling characteristics with OCT characteristics of cystoid spaces and serous retinal detachment in DME. These authors have suggested several mechanisms by which the blood-retinal barrier is disrupted and concomitant edematous changes develop.37
Otani et al. were among the first who observed structural changes in DME by OCT, and they described three types: sponge-like swelling (88%), edema with cystic spaces (47%) and edema with serous retinal detachment (15%).38 Shin et al. found that structural changes in restoration of the photoreceptor inner and outer segment junction (IS/OS) and the ELM might be predictive factors for visual acuity before and after treatment with intravitreal triamcinolone acetonide.39
In addition, the presence of hyper-reflective foci in the outer retina seems to be closely associated with a disrupted ELM and IS/OS line on SD-OCT images and decreased visual acuity in DME.40 Also, OCT imaging seems to have predictive value when considering surgical treatment in DR and DME.
Gentile et al. found that a taut ILM can cause diffuse DME after vitrectomy, and its removal can restore the normal foveal contour and improve visual acuity. The authors hypothesized that tangential tractional forces from contractile cells that propagated across the fovea via the ILM might be the etiology.41,42
Some of the early morphological changes in DME seem to be better appreciated with OCT than FA. Few researchers have correlated intraretinal abnormalities found on OCT with FA changes. In particular, vitreomacular traction and serous macular detachment have evidently been better visualized and detected with OCT compared to FA in multiple studies.43-46
Considering all of the substructural changes revealed by OCT imaging, there might be a need for an update of DME classification.
QUANTIFICATION OF DME USING OCT
The quantitative assessment of DME includes retinal thickness, central macular thickness and macular volume measurements. Retinal thickness is measured as the distance between automatic segmentation of the inner and outer retinal borders. Most instruments identify the vitreoretinal interface as the inner retinal border.
However, the segmentation of the outer border differs between instruments. Based on this fact, discrepancies in macular thickness measurements in healthy eyes between among OCT instruments have been identified.33,47-48 Various studies have compared retinal thickness between the normal and diabetic populations. Those parameters have been useful for the early detection of macular thickening and could be used as early indicators of disease progression in patients with diabetic retinopathy.
Although SD-OCT provides plenty of new insights into substructural retinal changes associated with DR and DME, it does not test retinal function. Additional functional tests, such as microperimetry or multifocal ERG, might help us to understand which structural changes finally lead to visual impairment. Furthermore, standard SD-OCT cannot provide information regarding retinal circulation, which is problematic because DR is mainly a vascular disease. OCT only shows the structural results of circulatory dysfunction but might not provide enough information to understand the underlying mechanisms.
Currently, FA remains the best monitoring tool in ischemic DME, as OCT cannot provide sufficient information regarding this particular condition.33,42 The future will show whether new techniques, such as Doppler blood flow OCT devices, or other diagnostic techniques such as retinal oximetry, will add further to the understanding of DME development.49-51
THE ROLE OF OCT IMAGING IN DME TREATMENT
Laser photocoagulation is still considered the gold standard in treating DME.4 However, because standard photocoagulation leads to thermal retinal damage and permanent loss of photoreceptors at the location of treatment, new methods of laser treatment have been investigated.
Takatsuna et al. reported on the long-term efficacy of suprathreshold micropulse diode laser photocoagulation, which uses laser pulses shorter in duration than thermal relaxation time of a given tissue, thus generating microexplosions and thermomechanical effects with lower-power micropulse, in the treatment of DME, with promising benefits with regard to best-corrected visual acuity and OCT-measured retinal thickness.52
Framme et al. used SD-OCT to demonstrate postoperative RPE proliferation, RPE atrophy and neurosensory retina alteration following laser photocoagulation. Future directions in DME treatment might be focused more on medical therapy or mild laser, such as selective laser therapy using Nd:YLF to prevent structural damage;53 SD-OCT will help to prove the effects of such therapies (Figure 4).
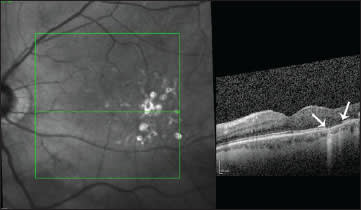
Figure 4. Spectralis OCT HRA picture of a patient with non-proliferative diabetic retinopathy and focal macular edema after laser photocoagulation. Corresponding to the laser scar, damage of the photoreceptor layer can easily be identified by OCT.
In cases of DME not responding to laser treatment, medical treatment is a valid option. The TDMO study reported a reduction in central macular thickness, observed by OCT, and significant improvement in visual acuity.54 Recently published studies show even better efficacy of a sustained-release delivery system of dexamethasone (Ozurdex), compared to triamcinolone, in the treatment of refractory and diffuse DME.55-56
The PACORES group reported that primary intravitreal bevacizumab for diffuse DME seems to provide stability or improvement in BCVA, OCT, and FA after 12 months of treatment.57 Results of the RESOLVE study (Group RS) also showed promising outcomes for the safety and efficacy of ranibizumab treatment in patients with DME at 12 months' duration.33,58
All of these trials used OCT retinal thickness measurements as secondary or even primary outcomes. OCT imaging has become a standard procedure worldwide concerning the indications for anti-VEGF treatment, follow-up, and evaluation of therapy response. In clinical trials, OCT is used for the early detection of pathomorphological changes due to adverse effects of novel treatments and to observe and document treatment success.59-62 Furthermore, OCT might help us to develop new ideas and approaches to novel diagnostic and therapeutic options.
CONCLUSION
Although new therapeutic options such as anti-VEGF, slow-release steroid therapy or selective laser treatment have evolved, DME remains a challenging complication of DR that is potentially sight-threatening. Good quality fundus photography remains an important diagnostic tool to document and follow up DR and DME. Progress has been made in the development of wide-angle, nonmydriatic fundus imaging systems. Digitalization has helped to develop telemedicine projects and has assisted DR reading centers in facilitating screening, even in remote areas without retinal specialists.
The invention of OCT had great impact on the management of DR and DME. Quantification of DME has become more reproducible and precise. In addition, OCT has revealed many substructural retinal changes that are associated with disease progression and correlated with visual function.
Future research should lead to the development of more precise methods for discerning various retinal layers and boundaries. Improved imaging helps us to understand which retinal changes need to be treated and which do not. Observation of the development and progression of various intraretinal changes might lead to new ideas for novel therapeutic approaches and better recognition of those patients who are at high risk for disease progression or who would be good responders to treatment.
There is no doubt that OCT imaging provides tremendous advantages, but we should not forget that this technique has some limitations. OCT is still incapable in evaluating ischemic areas and retinal circulation in the macular region. Impaired retinal circulation and ischemia are among the landmarks of diffuse DME resistant to therapy and are challenging factors in visual prognosis.
Technological improvements will also further increase our understanding of DME by refining our knowledge regarding the pathomechanisms that lead to retinal cell death. In the future, color Doppler OCT might provide retinal blood flow information beyond structural imaging in a noninvasive way. In addition, measurements of retinal oxygenation might yield further information regarding the metabolic changes associated with DME and DME treatment. RP
REFERENCES
1. Zhang X, Saaddine JB, Chou CF, et al. Prevalence of diabetic retinopathy in the United States, 2005-2008. JAMA. 2010; 304:649-656.
2. Klein R, Klein BE, Moss SE, Davis MD, DeMets DL. The Wisconsin epidemiology study od diabetic retinopathy.IV Diabetic macular edema. Ophthalmology. 1984; 91:1464-1474.
3. Anticliff RJ, Marshall J. The pathogenesis of edema in diabetic maculopathy. Semin Ophtalmol. 1999; 14:223-1232.
4. American Academy of Ophthalmology Retina Panel. Preferred Practice Pattern Guidelines. Diabetic Retinopathy. Available at: http://one.aao.org/CE/PracticeGuidelines/PPP.aspx. Accessed on February 2, 2010.
5. Retinopathy and nephropathy in patients with type 1 diabetes four years after a trial of intensive therapy. N Engl J Med. 2000; 342:381-389.
6. Gardner TW, Antonetti DA, Barber AJ, LaNoue KF, Levison SW. Diabetic retinopathy: more than meets the eye. Surv Ophthalmol. 2002; 47(Suppl 2):S253-S262.
7. Ferrara N, Gerber HP, LeCouter J. The biology of VEGF and its receptors. Nat Med. 2003; 9:669-676.
8. Diabetes Control and Complications Trial Research Group. Progression of retinopathy with intensive versus conventional treatment in the Diabetes Control and Complications Trial. Ophthalmology. 1995; 102:647-661.
9. Chen E, Looman M, Laouri M, et al. Burden of illness of diabetic macular edema: literature review. Curr Med Res Opin. 2010; 26:1587-1597.
10. Abràmoff M, Garvin M, Sonka M. Retinal imaging and image analysis. IEEE Rev Biomed Eng. 2010; 3:169-208.
11. Hofer H, Chen L, Yoon G, Singer B, Yamauchi Y, Williams D. Improvement in retinal image quality with dynamic correction of the eye's aberrations. Opt Express. 2001; 8:631-643.
12. Lim J, LaBree L, Nichols T, Cardenas I. A comparison of digital nonmydriatic fundus imaging with standard 35-millimeter slides for diabetic retinopathy. Ophthalmology. 2000; 107:866-870.
13. Mead A, Burnett S, Davey C. Diabetic retinal screening in the UK. J R Soc Med. 2001; 94:127-129.
14. Teng T, Lefley M, Claremont D. Progress towards automated diabetic ocular screening: a review of image analysis and intelligensystems for diabetic retinopathy. Med Biol Eng Comput. 2002; 40:2-13.
15. Yannuzzi L, Ober M, Slakter J, Spaide R, Fisher Y, Flower R, Rosen R. Ophthalmic fundus imaging: today and beyond. Am J Ophthalmol 2004; 137:511-524.
16. Sanchez CR, Silva PS, Cavallerano JD, Aiello LP, Aiello LM. Ocular telemedicine for diabetic retinopathy and the Joslin Vision Network. Semin Ophthalmol. 2010; 25:218-224.
17. Kernt M, Pinter F, Hadi I, et al. [Diabetic retinopathy: comparison of the diagnostic features of ultra-widefield scanning laser ophthalmoscopy Optomap with ETDRS 7-field fundus photography]. Ophthalmologe. Feb; 108:117-123. German.
18. Photocoagulation for diabetic macular edema. Early Treatment Diabetic Retinopathy Study report number 1. Early Treatment Diabetic Retinopathy Study research group. Arch Ophthalmol. 1985; 103:1796-1806.
19. Lobo C, Bernardes R, Santos F, Cunha-Vaz J. Mapping retinal fluorescein leakage with scanning laser fluorometry of the human vitreous. Arch Ophthalmol. 1999; 117:631-637.
20. Lobo C, Bernardes R, Cunha-Vaz J. Alterations of the blood retinal barrier and retinal thickness in preclinical retinopathy in subjects with type 2 diabetes mellitus. Arch Ophthalmol. 2000; 118:1364-1369.
21. Lobo C, Bernardes R, Abreu J, Cunha-Vaz J. One-year follow-up of blood retinal barrier and retinal thickness in patients with type 2 diabetes mellitus and mild non proliferative diabetic retinopathy. Arch Ophthalmol. 2001; 119:1469-1474.
22. Lobo C, Bernardes R, Figueira J, Abreu J, Cunha-Vaz J. Three-year follow-up of blood retinal barrier and retinal thickness in patients with type 2 diabetes mellitus and mild non proliferative diabetic retinopathy. Arch Ophthalmol. 2004; 122:211-217.
23. Bernardes R, Dias J, Cunha-Vaz J. Mapping the human blood-retinal barrier function. IEEE Trans Biomed Eng. 2005; 52:106-116.
24. Cunha-Vaz J. Measurement and mapping of retinal leakage and retinal thickness - surrogate outcomes for the initial stages of diabetic retinopathy. Curr Med Chem. 2002; 2:91-108.
25. Cunha-Vaz J, Bernardes R. Non proliferative retinopathy in diabetes type 2. Initial stages and characterization of phenotypes. Prog Retin Eye Res. 2005; 24:355-377.
26. Docchio F, ed. Introduction to Ocular Fluorometry. EUROEYE; Coimbra, Portugal; 1997.
27. el Harrar N, Idali B, Moutaouakkil S, et al. [Anaphylactic shock caused by application of fluorescein on the ocular conjunctiva]. Presse Med. 1996; 25:1546-1547. French.
28. Fineschi V, Monasterolo G, Rosi R, Turillazzi E. Fatal anaphylactic shock during a fluorescein angiography. Forensic Sci Int. 1999; 100:137-142.
29. Hitosugi M, Omura K, Yokoyama T, et al. An autopsy case of fatal anaphylactic shock following fluorescein angiography: a case report. Med Sci Law. 2004; 44; 264-265.
30. Huang D, Swanson EA, Lin CP, et al. Optical coherence tomography. Science. 1991:22:1178-1181.
31. Lumbroso B, Rippoli M. Guide to Interpreting Spectral Domain Optical Coherence Tomography. James Allyn, Inc.; Dublin, CA; 2010.
32. Hee MR, Puliafito CA, Wong C, et al. Quantitative assessment of macular edema with optical coherence tomography. Arch Ophthalmol. 1995; 113:1019 1029.
33. Salam A, Framme C, Wolf S. How SD-OCT is changing our view of DME. Retin Physician. 2010; 7(8):41-46.
34. Heidelberg Engineering. Spectralis OCT. Available at: http://www.heidelbergengineering.com/germany/produkte/spectralis-oct/. Accesssed April 11, 2012.
35. Murakami T, Nishijima K, Akagi T, et al. Optical coherence tomographic reflectivity of photoreceptors beneath cystoid spaces in diabetic macular edema. Invest Ophthalmol Vis Sci. 2012 Feb 8. [Epub ahead of print]
36. Giammaria D, Ioni A, Bartoli B, Cofini V, Pellegrini G, Giannotti B. Comparison of macular thickness measurements between time-domain and spectral-domain optical coherence tomographies in eyes with and without macular abnormalities. Retina. 2011; 31:707-716.
37. Horii T, Murakami T, Nishijima K, et al. Relationship between fluorescein pooling and optical coherence tomographic reflectivity of cystoid spaces in diabetic macular edema. Ophthalmology. 2012 Feb 11. [Epub ahead of print]
38. Otani T, Kishi S, Maruyama Y. Patterns of diabetic macular edema with optical coherence tomography. Am J Ophthalmol. 1999; 127:688-693.
39. Shin HJ, Lee SH, Chung H, Kim HC. Association between photoreceptor integrity and visual outcome in diabetic macular edema. Graefes Arch Clin Exp Ophthalmol. 2012; 250:61-70.
40. Uji A, Murakami T, Nishijima K, et al. Association between hyperreflective foci in the outer retina, status of photoreceptor layer, and visual acuity in diabetic macular edema. Am J Ophthalmol. 2011 Dec 1. [Epub ahead of print]
41. Panozzo G, Gusson E, Parolini B, Mercanti A. Role of optical coherence tomography in diagnosis and follow up diabetic macular edema. Semin Ophthalmol. 2003; 18:74-81.
42. Gentile RC, Milman T, Eliott D, Romero JM, McCormick SA. Taut internal limiting membrane causing diffuse diabetic macular edema after vitrectomy: clinicopathological correlation. Ophthalmologica. 2011; 226:64-70.
43. Soliman W, Sander B, Hasler PW, Larsen M. Correlation between intraretinal changes in diabetic macular edema seen in fluorescein angiographic and optical coherence tomography. Acta Ophthalmol. 2008; 86:34-39.
44. Kang SW, Park CY, Ham DI. The correlation between fluorescein angiographic and optical coherence tomography feature in clinically significant diabetic macular edema. Am J Ophthalmol. 2004; 137:313-322.
45. Ozdemir H, Karacorlu M, Karacorlu S. Serous macular detachment in diabetic cystoid macular edema. Acta Ophthalmol Scand. 2005; 83:63-66.
46. Koleva-Georgieva D, Sivkova N. Assessment of serous macular detachment in eyes with diabetic macula edema by use of spectral-domain optical coherence tomography. Graefes Arch Clin Exp Ophthalmol. 2009; 247:1461-1469.
47. Wolf-Schnurrbusch U, Ceklic L, Brinkmann CK, et al. Macular thickness measurement in healthy eyes using six different optical coherence tomography instruments. Invest Ophthalmol Vis Sci. 2009; 50:3432-3437.
48. Menke MN, Dabov V, Sturm V. Comparison of three different optical coherence tomography models for total macular thickness measurements in healthy controls. Ophthalmologica. 2009; 223:352-356.
49. Konduru RK, Tan O, Nittala MG, Huang D,Sadda SR. Reproducibility of retinal blood flow measurements derived from semiautomated Doppler OCT analysis. Ophthalmic Surg Lasers Imaging. 2012; 43:25-31.
50. Srinivasan VJ, Radzakrishnam H, Lo EH, et al. OCT methods for capillary velocimetry. Biomed Opt Express. 2012; 3:612-629.
51. Palsson O, Geirsdottir A, Hardarson SH, Olafsdottir OB, Kristjansdottir JV, Stefánsson E. Retinal oximetry images must be standardized. A methodological analysis. Invest Ophthalmol Vis Sci. 2012 Mar 6. [Epub ahead of print]
52. Takatsuna Y, Yamamoto S, Nakamura Y, Tatsumi T, Arai M, Mitamura Y. Longterm therapeutic efficacy of the subthreshold micropulse diode laser photocoagulation for diabetic macular edema. Jpn J Ophthalmol. 2011; 55:365-369.
53. Framme C, Alt C, Theisen-Kunde D, Brinkmann R. Structural changes of the retina after conventional laser photocoagulation and selective retinal treatment (SRT) in spectral-domain optical coherence tomography. Curr Eye Res. 2009; 34:568-579.
54. Gillies MC, Sutter FK, Simpson JM, Larsson J, Ali H, Zhu M. Intravitreal triamcinolone for refractory diabetic macular edema: two-year results of a double-masked, placebo-controlled, randomized clinical trial. Ophthalmology. 2006; 113:1533-1538.
55. Boyer DS, Faber D, Gupta S, et al.; Ozurdex CHAMPLAIN Study Group. Dexamethasone intravitreal implant for treatment of diabetic macular edema in vitrectomized patients. Retina. 2011; 31:915-923.
56. Zucchiatti I, Lattanzio R, Querques G, et al. Intravitreal dexamethasone implant in patients with persistent diabetic macular edema. Ophthalmologica. 2012 Feb 3. [Epub ahead of print]
57. Arevalo JF, Sanchez JG, Fromow-Guerra J, et al.; Pan-American Collaborative Retina Study Group (PACORES). Comparison of two doses of primary intravitreal bevazumab (Avastin) for diffused diabetic macular oedema: result from the Pan American Collaborative Retina Study Group (PACORES) at 12 months follow up. Graefes Arch Clin Exp Ophthalmol. 2009; 247:735-743.
58. Wolf S, Massin P, Bandello F, et al. Safety and efficacy of ranibizumab treatment in patients with diabetic macular edema: 12-months results of the RESOLVE study. Paper presented at: Annual meeting of the Association for Research in Vision and Ophthalmology; Fort Lauderdale, FL; May 6, 2009.
59. Pece A, Isola V, Piermarocchi S, Calori G. Efficacy and safety of anti-vascular endothelial growth factor (VEGF) therapy with intravitreal ranibizumab (Lucentis) for naive retinal vein occlusion: 1-year follow-up. Br J Ophthalmol. 2011; 95:56-68.
60. Lorenzo D, Arias L, Alcubierre R, et al. Intravitreal ranibizumab for choroidal neovascularization secondary to pathological myopia: 12-month follow-up. Ophthalmologica. 2011; 226:103-109.
61. Gharbiya M, Giustolisi R, Allievi F, et al. Choroidal neovascularization in pathologic myopia: intravitreal ranibizumab versus bevacizumab — a randomized controlled trial. Am J Ophthalmol. 2010; 149:458-64.e1.
62. Querques G, Forte R, Berboucha E, et al. Spectral-domain versus time domain optical coherence tomography before and after ranibizumab for age-related macular degeneration. Ophthalmic Res. 2011; 46:152-159.