PEER REVIEWED
Considering the Illumination Choices in Vitreoretinal Surgery
Continual improvements allow for better, safer outcomes.
Hirokazu Sakaguchi, MD • Yusuke Oshima, MD
Since Robert Machemer, MD, first pioneered closed eye vitrectomy,1 numerous advances in the technologies and techniques have been made for the establishment and refinement of this surgical modality, including enormous advances in minimizing the size of surgical equipment for carrying out sutureless vitrectomy.
As a result, transconjunctival microincision vitrectomy (MIVS) using small-gauge (23- or 25-gauge) instrumentation has emerged as a standard surgical style for modern pars plana vitrectomy.2,3 The advantages of small-gauge vitrectomy are now well recognized, including less traumatic intraocular access and faster patient recovery. With the introduction of this modern surgical style, there have been several innovations in surgical technologies.
Among these innovations is the development of powerful light sources and endoilluminating systems. When looking at the past history of illumination for vitrectomy, an external slit illuminator outside the eye was first used in the early 1970s.1 The current style of endoillumination, using an optic fiber inserted into the vitreous cavity, was first introduced in 1976 by Gholam A. Peyman, MD, for 20-gauge three-port vitrectomy.4 After Peyman, different types of light probes offering wide-angle endoillumination were made available for use with wide-angle fundus viewing systems.5,6 These light probes are currently the standard tool for endoillumination during pars plana vitrectomy.
Hirokazu Sakaguchi, MD, is assistant professor of ophthalmology at the Osaka University Graduate School of Medicine in Suita, Japan. Yusuke Oshima, MD, is associate professor of ophthalmology at the same institution. Dr. Sakaguchi reports no financial interest in any products mentioned in this article. Dr. Oshima is an international board member of Alcon Laboratories and a consultant to Topcon Medical Laser Systems and Synergetics. He has received lecture fees and travel support from Alcon Laboratories, Carl Zeiss Meditec, DORC International, Synergetics, Santen Japan and Senju Japan, when he spoke at sponsored seminars, but he received no proprietary interests or royalties from any companies in relation to any products mentioned in this article. Dr. Oshima can be reached at yusukeoshima@gmail.com. |
In the 20-g era, a halogen or metal halide light bulb was used as the illuminating light source. However, when using a small-gauge optic fiber with a conventional halogen light source, only 50% or less of the brightness found with the 20-g system was obtained. Therefore, recent advancements in illumination systems, including both the light source and endoilluminating style, were important factors in pushing the widespread use of MIVS.
This review is based on recent papers that were obtained from a Medline database search, an industry literature review, personal communications and personal experience. The aim of this article is to provide the reader with a comprehensive review of the latest advances in illumination for modern vitreous surgery.
NOVEL LIGHT SOURCES FOR SMALL-GAUGE VITRECTOMY
To compensate for the insufficient brightness of illumination through small-gauge light probes with conventional halogen light bulbs, improved light sources, i.e., xenon and mercury vapor light bulbs, were introduced.
Xenon Light Illumination
The increased lumens of xenon light sources are substantial, even with a 25-g optic fibers or smaller, and the intraocular illumination is equal to or brighter than the illumination achieved with 20-g probes and conventional halogen or metal halide light bulbs. There are several standalone xenon light illuminators that are commercially available, such as Photon (Synergetics), Accurus High Brightness Illuminator (AHBI, Alcon Laboratories), and Bright Star (DORC). In addition, xenon light bulbs are the standard illuminating systems in the next-generation vitrectomy machines, such as the Constellation Vision System (Alcon Laboratories) and the Stellaris PC (Bausch + Lomb), indicating that small-gauge optic fibers with powerful light sources will be the standard for pars plana vitrectomy for the foreseeable future.
There has been concern raised over possible retinal phototoxicity with xenon light sources because the spectral distribution of xenon light involves the theoretical phototoxic hazard area in aphakic human eyes (Figure 1). Therefore, current commercially available xenon illuminators feature lower wavelength filters (at least <420 nm), cutting off ultraviolet and blue light to reduce phototoxicity with higher hazard efficiency.
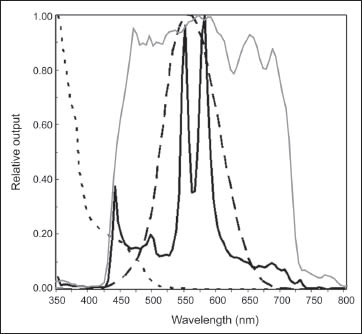
Figure 1. Spectral output curves of a xenon light (gray solid curve) and a mercury vapor light (black solid curve), normal photopic spectral sensitivity curve (dashed curve), and a spectral curve of phototoxic hazard in aphakic eyes (dotted line). Most of the currently available xenon illuminators feature the standard lower wavelength filters (at least <420 nm), cutting off ultraviolet and blue light. The relative output of the mercury vapor lamp is filtered by an integrated 435-nm filter, resulting in an output curve with two peaks at 550 nm and 580 nm, mostly confined within the photopic spectral sensitivity of humans.
In addition, some illuminators have incorporated stronger filters or filter options to increase safety. For example, the Synergetics Photon has an adapter with a 485-nm filter. According to a study by David R. Chow, MD,7 this filter may improve the safety calculation of retina threshold time by 26 times, because the safety of a light source is a function of its output in the range of the aphakic hazard curve.
The DORC Bright Star (DORC) is capable of providing different fundus viewing environments (Figure 2) by changing filters (~420 nm, ~435 nm, ~475 nm and ~515 nm) to produce different lighting within the eye. When using the 515-nm filter, the safety is increased by almost 30 times, compared with the standard 420-nm filter. The Stellaris PC also features a xenon light source with three types of built-in filters (green-tin, yellow-tin and amber filters) increasing the aphakic hazard curve safety as compared with the normal filter.
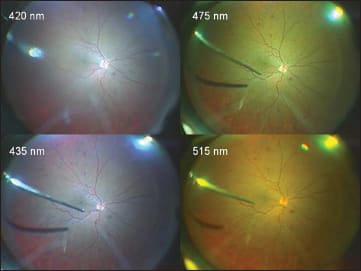
Figure 2. Different colors of fundus viewing environments by changing the lower wavelength filters with a xenon light source (DORC Bright Star). Using a higher wavelength cut filter may allow surgeons to perform surgery for much longer times safely in challenging cases. Instead, the illuminating light hue will shift to a more yellowish color due to the filter.
Table 1 demonstrates the theoretical hazard time (retinal threshold time) depending on the filter choice. A different filter can increase the working time available with the light source, allowing surgeons to perform surgery for longer times in challenging cases, even though the illuminating light hue will be yellowish due to the filter (Figure 3).
Table 1. Filters and Threshold Times | |||
---|---|---|---|
Unit/filter (nm) | ISO value(10-lm output) | Threshold time (10-lm output) | 100% output (lm) |
BrightStar 420 | 0.7 | 11 m 54 s | 45 |
BrightStar 435 | 0.55 | 15 m 09 s | 45 |
BrightStar 475 | 0.16 | 52 m 04 s | 44.6 |
BrightStar 515 | 0.017 | 8h 10 m 12 s | 37.8 |
Measurements done according to ISO15751
Used optic fiber: straight end 23-g (D06)
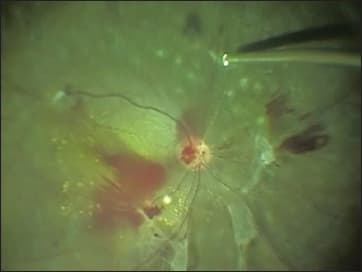
Figure 3. Green-yellowish endoillumination generated by a mercury vapor light source.
Mercury Vapor Light Illumination
To obtain a more powerful illumination source with minimal phototoxicity, a mercury vapor illuminator (Photon II; Synergetics) has been developed.8 This instrument features a dual-output pathway from one mercury vapor bulb, with spherical reflectors adapted to generate homogenized illumination and sharpen the focus spot of the illumination. The luminous efficacy of the mercury vapor illuminator reaches 402 lumens/watt of optical power, which is brighter than any commercially available xenon light illuminators (range, 277-355 lm/W). The actual output level of the mercury vapor illuminator can be enhanced to 56 lm through a 25-g optic fiber, which is approximately twice as bright as that of the xenon light source (photon) that puts out 29 lm through the same optic fiber.
This illumination system incorporates a 435-nm cutoff filter to reduce exposure to ultraviolet and blue light. After passing through the cutoff filter, the output of the mercury vapor illuminator has only two spectral output peaks at 550 nm and 580 nm, and the entire spectral output curve is mostly confined within the range of the photopic spectral system (Figure 1). The hazard efficacy, which represents the magnitude of theoretical phototoxicity, is 2,200 lm/hazard W in the mercury vapor illuminator, which is much higher than the hazard efficacy measured in a xenon or halogen light source (range, 1,150-1,900 lm/hazard W).
The chromaticity of commercially available illuminators is demonstrated in Figure 4. Of these illuminators, because they only have two spectral output peaks (550 nm and 580 nm), illumination generated by a mercury vapor bulb is green-yellow (Figure 5), which produces a less dazzling reflection from the fundus and thus may be comfortable to the surgeon’s eyes. Because yellow and blue are complementary colors, green-yellowish illumination can provide clear contrast during peeling of the ILM stained with brilliant blue G dye. This may be an additional advantage of using a mercury vapor light source (Figure 5). Given these advantages, the Stellaris PC features a mercury vapor bulb, as well as a xenon bulb, as the standard light source for endoillumination.
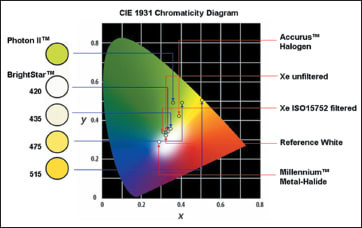
Figure 4. A comparison of the chromaticity of currently available illuminators.
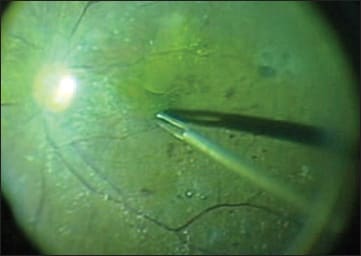
Figure 5. The green-yellowish illumination generated by the mercury vapor light source provides a clear contrast between the ILM-peeled and -unpeeled retina after staining the ILM with brilliant blue G because of the complementary color pairing of yellow and blue.
Overall, novel illuminating systems, using both xenon and mercury vapor bulbs, have improved efficiency and safety compared to conventional illuminating systems. Because of their powerful illumination capabilities, high levels of illumination can be achieved through small-gauge optic fibers.
Although these new illuminating systems have integrated filter systems to improve safety by cutting off high levels of ultraviolet and blue light, surgeons should bear in mind that no commercially available light sources, including xenon and mercury vapor lights, are safe for extremely long periods of exposure with respect to photochemical retinal damage.9
WIDE-ANGLE ENDOILLUMINATION
On the heels of widespread adoption of wide-angle viewing systems and new light sources during vitrectomy, several new light probes have been developed to achieve wide-angle endoillumination and to minimize wide-angle illumination–induced glare.10 Most of the recently developed light probes offer a more than 100º field of view, compared with conventional “focal” light pipes with illumination fields ranging from 50º to 80º. Many of these wide-angle light probes feature beveled sheaths on the tip to prevent glare, thus providing diffuse and uniform endoillumination even while performing a fluid-air exchange.
THE RATIONALE FOR USING CHANDELIER ENDOILLUMINATION
When considering retinal phototoxicity, the working distance for light irradiation is very important; thus, holding the light probe as far away from the retina as possible increases safety. Based on this theory, chandelier endoillumination would be the safest way to avoid retinal phototoxicity when using the same powerful light source. In fact, increasing the working distance of the light probes from 4 mm to 8 mm from the retina can theoretically increase the retinal threshold time more than threefold. If a chandelier endoilluminator is used, the retinal threshold time can be extended for an extremely long time compared to the other types of light probes, as shown in Table 2.
Table 2. Distance and Threshold Times | ||
---|---|---|
Assessed at Max Production Output | Working Distance 4 mm | Working Distance 8 mm |
Alcon Accurus Halogen 20g light pipe High 3 | 13 m 02 s | 43 m 32 s |
B&L Millennium Metal Halide 20g light pipe on Max | 6 m 44 s | 22 m 28 s |
DORC Hexon Metal Halide 20g light pipe on Max | 7 m 13 s | 27 m 01 s |
Synergetics Xenon 20g light pipe Max | 9 m 14 s | 30 m 50 s |
Synergetics Xenon 25g Awh Chandelier on Max | 4 h 17 m 39 s |
In addition to the safety advantage, chandelier endoilluminators can free up a surgeon’s hand from holding a light probe, thus allowing true bimanual manipulation during surgery. In cases with retinal detachment, for example, surgeons can perform scleral indentation by themselves to achieve more controlled and smooth peripheral vitreous base shaving, rather than them being done by an assistant. For membrane dissections in challenging cases, such as diabetic tractional retinal detachment or proliferative vitreoretinopathy, the freed hand is helpful for holding a forceps to grasp the membranes for separation from the retina or dissection using scissors or a cutter with the other hand (Figure 6).
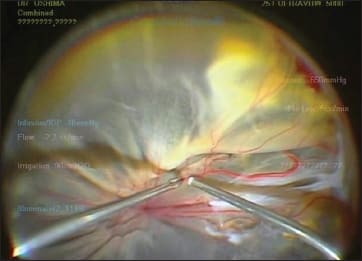
Figure 6. Chandelier endoillumination can free up surgeons’ hands for bimanual manipulation in complex cases because of its self-retaining nature and wide-angle illumination.
Chandelier endoillumination itself is not a new concept. Many surgeons have used the Torpedo Mini Lights chandelier endoilluminator with a single optic fiber or multiple optic fibers.11 However, because of insufficient brightness, either with a conventional halogen or metal halide bulb, this chandelier fiber failed to catch on. In contrast, thanks to the recently developed light sources, the optic fibers for the new chandelier endoilluminators have been developed in a variety of designs and gauges (Figure 7).
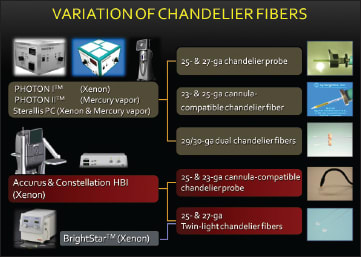
Figure 7. Variations of the currently available chandelier illumination fibers and their combinations with the new light sources and the new generation vitrectomy machines.
Currently, the gauge of choice ranges from 25-g to 27-g for single-fiber endoillumination and 27-g to 29-g for dual-fiber systems.8,12-15 All fibers are designed to be self-retaining after transconjunctival insertion, with or without the use of a trocar-cannula system.
Single-fiber chandelier endoillumination is very simple to use (Figure 8). To obtain sufficient brightness, some surgeons prefer to choose larger-gauge single fibers, i.e., a 25-g fiber rather than 27-g fiber or smaller. However, unlike the oblique incision technique required to insert the cannulas for vitrectomy, a vertical insertion for the 25-g optic fiber directly through the sclera is required to aim the light appropriately at the posterior pole. This vertical incision may cause leakage of the wound after removal of the fiber at the end of surgery, so surgeons need to check carefully after chandelier removal. Repositioning of the fiber is often necessary to complete 360º peripheral manipulation, such as performing peripheral vitreous shaving in retinal detachment cases.
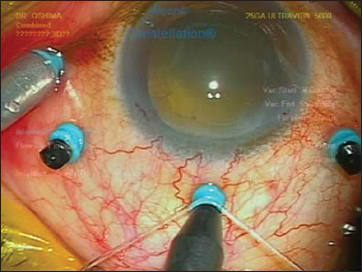
Figure 8. A 25-gauge cannula-guided single-fiber chandelier probe. It is simple to set and easy to self-retain transconjunctivally.
In addition, endoillumination from a single fiber makes it difficult to cover the whole eye homogenously. Therefore, a new 25-g single-fiber chandelier, featuring a trocar-cannula for easy repositioning of the fiber during surgery and a self-sealing wound, has been produced. There are some potential disadvantages of single-fiber chandeliers, including shadowing of instruments. If inserting the chandelier inferiorly, the illumination will come from the inferior side; thus, the annoying shadow would be located from the posterior pole to the superior retina, a desired working zone for intraocular manipulations. Therefore, inserting the fiber around the 12 o’clock position and making the shadow appear interiorly would avoid having the shadow interfere with the working zone (Figure 9).
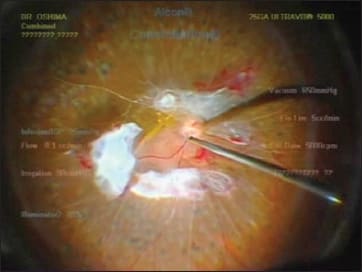
Figure 9. Setting the chandelier fiber around 12 o’clock (as shown in Figure 8) is helpful in making the shadow go down to the inferior area, which can help in avoiding the instrument shadow coming into working zone.
Chandelier endoillumination with two optic fibers, first designed by Eckardt as the Twin-light chandelier, is useful for obtaining homogenous and more widespread illumination, thus eliminating the need to reposition the fiber and minimizing the shadow seen with single-fiber chandelier endoillumination because of the illumination coming from two different directions.12,14
When using two-fiber chandelier systems, we no longer need to use larger-gauge fibers because sufficient brightness can be obtained from the total lumens produced by each fiber. Using smaller fibers is advantageous to produce easily self-sealing wounds despite the extra wounds needed for dual-fibers systems. Currently, the gauge of fibers has been minimized up to 30-gauge (0.3 mm), with the maximum output of 15 lm in each fiber with a mercury vapor bulb (Figure 10).15 These 30-g dual fibers are commercially available with 29-g cannulas to allow transconjunctival placement with easy fiber positioning and to prevent inadvertent removal of the fiber during surgery.
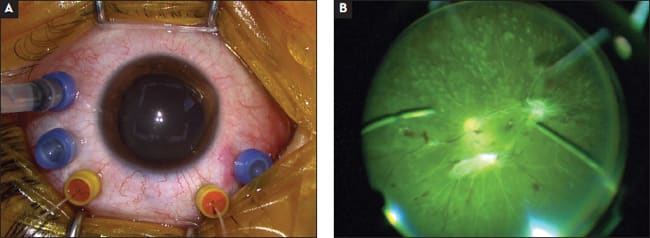
Figure 10. A 29/30-gauge dual-chandelier fiber system (A). The cannulas are helpful for fiber positioning and for avoiding missing the small wounds in cases of inadvertent removal of the fiber during surgery. Chandelier endoillumination with two separated ultra–small-gauge fibers provides homogenous and more widespread illumination (B).
Using either the 27-g Twin-light chandelier (DORC) or the 29/30-g dual chandelier (Synergetics), adjusting the dual tips is possible for optimizing the illuminating angle inside the eye and minimizing any glare from the tips. The direction of illumination can change from the posterior pole to the periphery by changing the curvature of the chandelier fiber outside the eyeball (Figure 11).
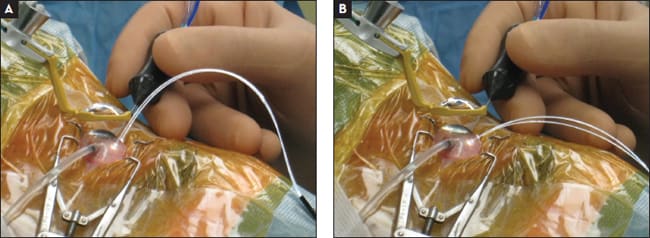
Figure 11. The direction of the illumination focusing on the posterior pole (A) or periphery (B) can be optimized easily by changing the curvature of the chandelier fiber outside the eyeball with this flexible type of chandelier fiber.
The most frequent concern with chandelier endoillumination is the glare to the surgeon, regardless of the type of chandelier fiber used. This annoying situation is often seen in eyes after fluid-air exchange and is further complicated by condensation at the posterior surface of intraocular lenses in pseudophakic eyes with posterior capsulotomy.
Reducing the brightness of the illumination, changing the illuminating angle of the fiber tip, using the amber filter to minimize reflection, instilling a viscoelastic substance on the lens surface or going back to using conventional light probes are all options to resolve this challenging situation. Removing any vitreous hemorrhage or vitreous debris adherent to the optic fiber tip immediately after starting vitrectomy is also an important procedure to prevent the melting of the chandelier optic fiber tip and to maintain bright and broad endoillumination in cases with dense vitreous hemorrhage.16
LOOKING TOWARD THE FUTURE
Excellent wide-angle visualization of the fundus can be achieved with the recently developed powerful light sources, endoillumination optic fiber systems, and improved wide-angle viewing systems, which further encourage surgeons to perform less invasive vitrectomies with smaller gauge instruments. Surgeons can currently choose the illumination source, illumination profiles with filters, endoprobes, and endoillumination styles according to the severity or simplicity of the individual case.
However, surgeons must still bear in mind that the question remains: What is the optimal setting and brightness level of illumination for desirable visualization during vitrectomy? The final goal of illumination is to enhance the efficiency of the surgery while maintaining safety. Similar to the introduction of mercury vapor bulbs in our field, the new LED light sources have unique potential and have recently been tested in prototype machines. The evolution of the next-generation illumination systems continues and looks very promising for the future. RP
REFERENCES
1. Machemer R, Buettner H, Norton EW, Parel JM. Vitrectomy: a pars plana approach. Trans Am Acad Ophthalmol Otolaryngol. 1971;75:813-820.
2. Fujii GY, De Juan E, and Humayun MS, et al. A new 25-gauge instrument system for transconjunctival sutureless vitrectomy surgery. Ophthalmology2002;109:1807-1812.
3. Eckardt C. Transconjunctival sutureless 23-gauge vitrectomy. Retina. 2005;25:208-211.
4. Peyman GA. Improved vitrectomy illumination system. Am J Ophthalmol. 1976; 81:99-100.
5. Ryan EH Jr. Two shield bullet probes for panoramic endoillumination. Arch Ophthalmol. 1997;115:125-126.
6. Peyman GA, Canakis C, Livir-Rallatos C, Easley J. A newwide angle endoillumination probe for use during vitrectomy. Retina. 2002;22:242.
7. Chow DR. Tips on improving your use of endoillumination. Retin Physician. 2011:8(4):43-46.
8. Oshima Y, Chow DR, Awh CC, Sakaguchi H, Tano Y. Novel mercury vapor illuminator combined with a 27/29-gauge chandelier light fiber for vitreous surgery. Retina. 2008;28:171-173.
9. van den Biesen PR, Berenschot T, Verdaasdonk RM, et al. Endoillumination during vitrectomy and phototoxicity thresholds. Br J Ophthalmol. 2000;84:1372-1375.
10. Rossi T, Boccassini B, Iossa M, Rossi A, Mutolo A, Tamburrelli C. Wedge-shaped light pipe aperture for pars plana vitrectomy. Retina. 2009:29;1201-1206.
11. Tornambe PE. Vitrectomy surgery illumination using #26 Torpedo Mini Lights. Video presented at: The Fourth Annual Vitreous Society Film Festival; San Francisco, CA: April 2-4, 2002.
12. Eckardt C. Twin lights: a new chandelier illumination for bimanual surgery. Retina. 2003;23:893- 894.
13. Oshima Y, Awh CC, Tano Y. Self-retaining 27-gauge transconjunctival chandelier endoillumination for panoramic viewing during vitreous surgery. Am J Ophthalmol. 2007;143:166-167.
14. Eckardt C, Eckert T, Eckardt U. 27-gauge Twinlight chandelier illumination system for bimanual transconjunctival vitrectomy. Retina. 2008;28:518-519.
15. Sakaguchi H, Oshima Y, Nishida K, Awh CC. A 29/30-gauge dual-chandelier illumination system for panoramic viewing during microincision vitrectomy surgery. Retina. 2011;31:231-1233.
16. Shimada H, Nakashizuka H, Hattori T, et al. Thermal injury caused by chandelier fiber probe. Am J Ophthalmol. 2007;143:167-169