Current-mediated Ocular Drug Delivery
Iontophoresis and electroporation as drug-delivery systems.
Francine Behar-Cohen, MD, PhD
Ocular drug delivery remains a major challenge in the development of treatments for diseases affecting the posterior segment of the eye. Among currentassisted drug delivery systems, iontophoresis and electroporation (also called electrotransfer when used for nucleic acid delivery) have been widely developed to deliver drugs or nucleic acids into the eye.
Both iontophoresis and electroporation use the application of an external electric field to biological systems, but they act upon separate and distinct biophysical scales and employ separate and distinct mechanisms to achieve different therapeutic goals.
Electroporation uses short electrical pulses (τ ≈ 100 µsec to 20 msec) that lead to transmembrane voltages of the fluid lipid bilayer of individual or groups of cells, reaching values high enough (Vm ≈ 0.7 to 1.0 V) to create new, transient, hydrophilic membrane pores.
Molecules that would otherwise not be permeable enough to pass through unperturbed cell membranes thus become exchangeable between the inside and the outside of the cell. This is achieved by the application of short (~ milliseconds) electrical pulses at field strengths both considerably higher (~ 100 V and currents of several amps) and of shorter pulse lengths than used in iontophoresis.1
Iontophoresis, in contrast, employs low voltages (< 10 V), and low currents (~ few mA) over much longer periods (minutes to tens of minutes or more) to provide a sustained and regulated driving force. Enhanced iontophoretic permeability is based on the classical laws of electrochemical diffusion, repulsion, and migration of charged and polar species, albeit taking place in the complex, multilayered, and often noncellular matrices of the dermis or in tissues such as those characteristically found in ocular structures.
Francine Behar-Cohen, MD, PhD, is professor in ophtalmology at the University Paris Descartes and a vitreoretinal surgeon at Assistance Publique Hôtel-Dieu Hospital in Paris. She reports moderate financial interest in the products mentioned in this article. Dr. Bar-Cohen can be reached via e-mail at francine.behar@gmail.com. |
Iontophoretic drug delivery has been widely studied for transdermal administration, but extrapolation of these findings to ocular tissues remains uncertain. Iontophoresis is thought to enhance drug delivery by three permeation mechanisms: (1) the ion-electric field interaction provides a force that drives ions through tissue; (2) the flow of electric current increases tissue permeability; and (3) electroosmosis produces bulk motion of the solvent, inducing a “solvent stream.”2
Optimal candidate drugs are low-molecular-weight, positively charged molecules. Although iontophoretic delivery could reasonably be expected to be inversely dependent upon the molecular weight of the permeating molecule or species, it has been shown that delivery of neutral molecules and even high-molecular-weight molecules, such as proteins, can also be transported across the skin by the means of iontophoresis. Not only the molecular size of target drugs but also their molecular shape can influence iontophoretic transport.
Both techniques result in transitory effects with total restoration of the cell and the tissue structure and properties, which make them safe techniques when used under appropriate conditions. Furthermore, electroporation and iontophoresis are not mutually exclusive therapeutically, and several recent examples have suggested their combination may serve to alleviate drawbacks in the other technique.3,4
In practice, electroporation is particularly adapted to enhance the intracellular penetration of plasmid DNA, allowing for nonviral gene delivery, while iontophoresis is more suitable for enhancing the intratissue penetration of drugs into the ocular tissues.
TRANS-SCLERAL IONTOPHORESIS: THE PAST AND THE PRESENT
Since the earliest description of zinc salt transcorneal iontophoresis by Wirtz in 1908, later described by Duke-Elder in 1962, and despite a century of publication in the ophthalmic literature, this technique has only recently been developed for clinical applications.
Indeed, early studies, using suboptimal electrode geometries and excessive current densities, reported corneal scarring and tissue damage5 that raised obvious safety concerns on the clinical use of iontophoresis. Many of the designed electrode arrangements for the earliest studies in this area were tubular, with reduced area of contact with the sclera over the pars plana, leading to a very high current density.
Small burns over areas where the current was applied were, not unsurprisingly, commonly described. Under these conditions, high drug concentrations in the vitreous were observed. However, the mechanism of penetration could be attributed, at least in part, to facilitated diffusion of the drug through ruptured tissue barriers. Very few of these studies reported on the complete pharmacokinetics of the target drugs after iontophoresis in the complete range of ocular tissues, which could have contributed to increased understanding of this method of administration.
In the early 1990s, Jean-Marie Parel, at the Bascom Palmer Eye Institute, and I, at l'Hôtel-Dieu in Paris, began to work on novel iontophoresis probes that had larger surfaces of application and that were applied on an area that was thought at that time to have lower resistance: the pars plicata.
We thought that drugs might penetrate through the sclera and follow anteroposterior and anterior migration routes and reach the ocular tissues without inducing high vitreous levels (Figure 1). The probe was 14 mm in inner diameter and 17 mm in outer diameter and covered the whole circumference around the cornea (Figure 2).
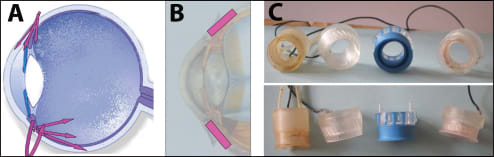
Figure 1. Design of trans-scleral iontophoresis probes. A. Theoretical routes of drug penetration into the eye using annular trans-scleral probes. B. Site of annular probe application. C. Front and lateral views of different early probes with either gel containing the drug or infusion of the drug into the reservoir (made in the JM Parel Laboratory at the Bascom Palmer Eye Institute).
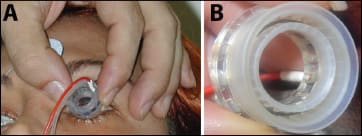
Figure 2. Patient treatment using disposable probe. A. Iontophoresis probe on a patient's eye with return electrode on the forehead. B. Higher magnification of the probe showing the metal electrode at the bottom of the reservoir.
This trans-scleral iontophoretic system has been extensively tested in animal models and has also been used in patients, showing that high levels of drugs (corticosteroids, antibiotics, antimitotics) were achieved in the retina and choroid without such high levels in the vitreous, the drug being driven from the outside toward the ocular tissues.6-10
These studies showed that current densities greater than 50 mA/cm2 thermally affected tissues, especially the conjunctival epithelium, where burns occurred at 100 to 140 mA/cm2. The placement of the electrode appeared crucial, and the design of our annular probe was optimal for guaranteeing the stability and the adequate placement of the reservoir.
We found that sampling only ocular media (aqueous humor and vitreous) did not reflect the efficacy of the delivery system when using trans-scleral approaches. Indeed, high levels of drugs were achieved in the choroid and retina but not in the vitreous using low voltages. In our hands, transscleral iontophoresis was not efficient for enhancing sufficiently the penetration of high-molecular-weight molecules to the posterior segment of the eye, even if penetration could be achieved in the anterior part of the retina.
In 2004, we published some of our results from a large clinical study evaluating the tolerance and efficacy of iontophoresis of methylprednisolone sodium succinate in patients with severe intraocular inflammation. Between April 1999 and October 2001, 93 patients were included in a study designed to evaluate the tolerance of trans-scleral iontophoresis.
Patients with severe intraocular inflammation requiring systemic corticosteroids were included in the study and received, instead of systemic therapy, trans-scleral iontophoresis of sodium succinate methylprednisolone 62.5 mg/mL. The intensity of the current was 1.7±0.18 mA for three minutes, and the patients received one to five treatments, with a mean number of 2.7 ±0.9.
The treatment was well tolerated, with 86% of the patients experiencing no or slight pain during the procedure. Figure 3 (A, B) shows an example of a patient with postoperative inflammation before and after one methylprednisolone iontophoretic treatment. Seventeen other patients were treated for acute graft rejection with iontophoresis of methylprenisolone in place of systemic pulse therapy. We showed that this local treatment allowed for the reversal of rejection, with an efficacy comparable to the known effects of pulse therapy in this indication10 (Figure 3 C, D).
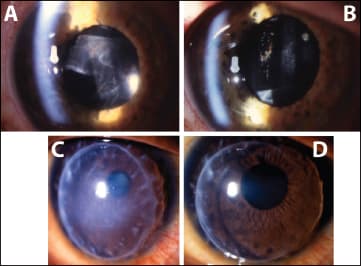
Figure 3. Examples of patients before and after treatment with iontophoresis of methylprednisolone sodium succinate (MPSS). Patients with acute anterior-segment inflammation before treatment (A) and 24 hours after a single iontophoresis of MPSS (62.5 mg/mL, 2.5 mA/cm2, 2 min) (B). Patient with acute corneal graft rejection before treatment (C) and 24 hours after three applications every day of iontophoresis of MPSS (62.5 mg/ml, 2.5 mA/cm2, 2 min) (D).
Already at day 10 and after three iontophoresis procedures, 88% demonstrated a complete reversal of the rejection processes. In two eyes, only partial and temporary improvement was observed. The mean BCVA of all 17 patients at the last follow-up visit was 0.37 ±0.2 compared to 0.06 ±0.05 before the initiation of the iontophoresis treatment. The mean follow-up time was 13.7 months, with a range of 5-29 months for the 17 patients. No significant side effects associated with the iontophoresis treatment were observed.
This technology was further developed by Optis France and is now under clinical development by Eyegate Pharma. Recently, Eyegate realized a prospective, phase 1/2 multicenter, double-masked, parallel-group, randomized clinical trial to evaluate the efficacy of the trans-scleral administration of dexamethasone in noninfectious uveitis. The results showed that two-thirds of the patients reached an anterior-chamber cell score of zero within 28 days, after receiving only one iontophoresis treatment and that the treatment was well tolerated.11
Another technology has been developed by Iomed to perform trans-scleral iontophoresis. The system is different as the semiannular reservoir is place in the cul de sac and covered by the eyelid.
MRI, which was applied to study how the drug penetrated the eye after trans-scleral iontophoresis using the model permeants manganese ion (Mn(2+)) and manganese ethylenediaminetetra-acetic acid complex (MnEDTA(2-)), showed that both anodal and cathodal iontophoresis provided significant enhancement in ocular delivery, compared to passive transport in the in vitro and in vivo experiments.
Trans-scleral iontophoretic delivery was related to the position and duration of the iontophoresis application in vivo. Permeants were observed to be delivered primarily into the anterior segment of the eye when the pars plana was the application site.
Extending the duration of iontophoresis at this site allowed the permeants to be delivered into the vitreous more deeply and to a greater extent than when the application site was at the back of the eye near the fornix, showing that electrode placement was an important factor in transscleral iontophoresis, and the ciliary body (pars plana) was determined to be the pathway of least resistance for iontophoretic transport.12
A NEW LOOK AT OCULAR ELECTROTRANSFER
As to the concept of therapeutic proteins for the treatment of chorioretinal diseases being approved, a primary challenge is to find novel drug delivery systems or gene therapy methods to achieve nontoxic, long-term, and efficient delivery of minimal efficient proteins concentration in situ.13
Many nonviral gene transfer methods have been developed and adapted for ocular gene therapy.14 Electrotransfer (ET) has been widely used in many cells and tissues and was shown to be among the more efficient methods both in preclinical15 and clinical studies.16
In the field of ocular gene therapy, previous reports have shown that after subretinal administration of plasmids, electrotransfer allowed for the efficient transfection of newborn murine RPE cells17 and delayed retinal degeneration in animal models.
A novel electroporation technique for specific transfection of the ocular ciliary muscle has recently been developed in our laboratory, aimed at using the ciliary muscle as a factory for high and long-lasting intraocular expression and the secretion of therapeutic proteins.18
In the eye, the ciliary smooth muscle is located at the crossroads between the anterior and posterior segments and is easily accessible (Figure 4). We have developed specific devices to allow the injection of plasmid DNA encoding for specific secreted proteins into the muscle, together with the application of a localized electric field using electrodes (Figure 5).
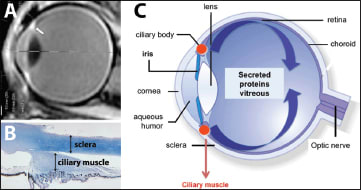
Figure 4. Ciliary muscle elctrotransfer. A. MRI imaging showing the location of the ciliary muscle in an adult eye. B. Histological structure of the muscle from an adult human eye. C. Theoretical diffusion of protein produced by the ciliary muscle after plasmid transfection in the muscle.
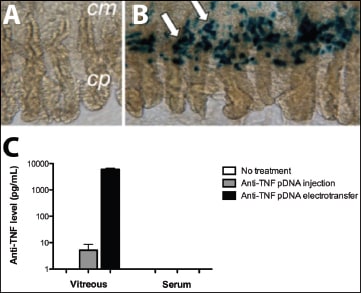
Figure 5. Effect of rat ciliary muscle transfection. Macroscopic examination of ciliary body eight days after naked plasmid transfection (A) and after transfection of a lac-Z (beta galactosidase activity is reflected by blue color, white arrows; cp: ciliary process; cm: ciliary muscle) (B). Levels of anti-TNF measured in the rat vitreous and in the serum three months after a simple injection of anti-TNF-plasmid DNA with or without electrotransfer (C).
Ciliary muscle electrotransfer offers many advantages:
• The transfected cells are non-neuronal cells and are easily reached without a true transparietal injection.
• The ciliary muscle is able to secrete any therapeutic protein in a sustained manner for at least nine months, although the secretion will be transitory.
• Levels of produced proteins are in the range of 10 ng/mL to 1µg/mL, which reaches the therapeutic level of most used compounds.19
• Intraocular levels can be modulated and increased by increasing the plasmid dose and/or the surface of transfected muscle for adaptation to the clinical response.20
• The procedure is quick (a few seconds) and does not require any surgical procedure.
The ciliary muscle electrotransfer method is being further developed by Eyevensys SAS for future clinical evaluation.
CONCLUSIONS
Current-mediated ocular drug delivery offers a wide range of treatment options, adaptable to the biological and physical-chemical characteristics of the therapeutic agent, as well as the treatment regimen and tissue or cell targets. The development of optimized devices and optimal electrical conditions will ensure both efficacy and safety. RP
REFERENCES
1. Weaver JC. Electroporation of cells. IEEE Trans Plasma Sci. 2000;28:24-33.
2. Pikal MJ. The role of electroosmotic flow in transdermal iontophoresis. Adv Drug Deliv Rev. 1992;9:201-237.
3. Badkar AV, Betageri GV, Hofmann GA, Banga AK. Enhancement of transdermal iontophoretic delivery of a liposomal formulation of colchicine by electroporation. Drug Deliv. 1999;6:111-115.
4. Bommannan DB, Tamada J, Leung L, Potts RO. Effect of electroporation on transdermal inotophoretic delivery of luteinizing hormone releasing hormone (LHRH) in vitro. Pharm Res. 1994;11:1809-1814.
5. Hughes L, Maurice D. A fresh look at iontophoresis. Arch Ophthalmol. 1984; 102:1825-1828.
6. Behar-Cohen F, Parel JM, Pouliquen Y, et al. Iontophoresis of dexamethasone in the treatment of endotoxin-induced-uveitis in rats. Exp Eye Res. 1997; 65: 533-545.
7. Behar-Cohen FF, Gautier S, El Aouni A, et al. Methylprednisolone concentrations in the vitreous and the serum after pulse therapy. Retina. 2001;21:48-53.
8. Behar-Cohen FF, El Aouni A, Gautier S, et al. Transscleral Coulomb-controlled iontophoresis of methylprednisolone into the rabbit eye: influence of duration of treatment, current intensity and drug concentration on ocular tissue and fluid levels. Exp Eye Res. 2002;74:51-59.
9. Behar-Cohen FF, Savoldelli M, Parel JM, et al. Reduction of corneal edema in endotoxin-induced uveitis after application of L-NAME as nitric oxide inhibitor in rats by iontophoresis. Invest Ophthalmol Vis Sci. 1998;39:897-904.
10. Halhal M, Renard G, Courtois Y, BenEzra D, Behar-Cohen F. Iontophoresis: from the lab to the bed side. Exp Eye Res. 2004;78:751-757.
11. Cohen AE, Assang C, Patane MA, From S, Korenfeld M; Avion Study Investigators. Evaluation of dexamethasone phosphate delivered by ocular iontophoresis for treating noninfectious anterior uveitis. Ophthalmology. 2012; 119:66-73.
12. Molokhia SA, Jeong EK, Higuchi WI, Li SK. Examination of penetration routes and distribution of ionic permeants during and after transscleral iontophoresis with magnetic resonance imaging. Int J Pharm. 2007;20;335:46-53.
13. El Sanharawi M, Kowalczuk L, Touchard E, Omri S, de Kozak Y, Behar-Cohen F. Protein delivery for retinal diseases: from basic considerations to clinical applications. Prog Retin Eye Res. 2010;29:443-465.
14. Bloquel C, Bourges JL, Touchard E, Berdugo M, BenEzra D, Behar-Cohen F. Non-viral ocular gene therapy: potential ocular therapeutic avenues. Adv Drug Deliv Rev. 2006;15;58:1224-1242.
15. Mir LM, Moller PH, André F, Gehl J. Electric pulse-mediated gene delivery to various animal tissues. Adv Genet. 2005;54:83-114.
16. Mir LM. Application of electroporation gene therapy: past, current, and future. Methods Mol Biol. 2008;423:3-17.
17. Matsuda T, Cepko CL. Controlled expression of transgenes introduced by in vivo electroporation. Proc Natl Acad Sci U S A. 2007;104:1027-1032.
18. Touchard E, Bloquel C, Bigey P, et al. Effects of ciliary muscle plasmid electrotransfer of TNF-alpha soluble receptor variants in experimental uveitis. Gene Ther. 2009;16:862-867.
19. Touchard E, Heiduschka P, Berdugo M, et al. Non-viral gene therapy for GDNF production in RCS rat: the crucial role of the plasmid dose. Gene Ther. 2011 Oct 13. doi: 10.1038/gt.2011.154. [Epub ahead of print]
20. Touchard E, Kowalczuk L, Bloquel C, Naud MC, Bigey P, Behar-Cohen F. The ciliary smooth muscle electrotransfer: basic principles and potential for sustained intraocular production of therapeutic proteins. J Gene Med. 2010; 12:904-919.