Growth Factor Therapy for Retinal Neuroprotection
CNTF's potential role in future retinal therapies.
Reema Syed, MD • Jacque L. Duncan, MD
Age-related macular degeneration is the leading cause of irreversible blindness in North merica, and retinitis pigmentosa (RP) affects an estimated 1.5 million peopleworldwide.1,2 Both AMD and RP are consideredretinal degenerative diseases, a heterogeneous group of disorders that are characterized by gradual destruction of the rod and cone photoreceptors with progressive, irreversible vision loss.2 Currently, there are no effective treatments to slow or prevent vision loss for either dry AMD orRP. However, research has demonstrated that neurotrophic factors may hold promise to treat these devastating diseases.
Pharmacological treatment of retinal degenerations using neurotrophic factors was first reported using basic fibroblast growth factor to delay photoreceptor degeneration in Royal College of Surgeons rats with inherited retinal dystrophy.3 Since then, a number of survival factors have been shown to rescue photoreceptors in animal models of inherited retinal degenerations.4
One of the most widely studied neurotrophic factors, ciliary neurotrophic factor (CNTF), has been shown to be effective in slowing photoreceptor loss in 12 animal modelsof inherited retinal degeneration.5 CNTF was identified as a survival factor in studies involving ciliary ganglion neurons in chick eyes.6 Human CNTF was first cloned in the early 1990s,7-9 and it consists of 200 amino acids that are structured into four alpha helices.10
CNTF exerts its activity via binding to form a complex with the CNTF receptor, which consists of a CNTFbinding protein (CNTFR alpha) and two proteins that are important for signal transduction: glycoprotein 130 and leukemia inhibitory factor receptor beta.11 CNTFR alpha has been localized to rods, cones, retinal pigment epithelial ells, inner nuclear cells, and retinal ganglion cells.12 Binding of CNTF to its receptor triggers multiple intracellular signaling events, including, but not limited to, the extracellular signal-regulated kinases (ERKs),13 the activation of which has been implicated in neuroprotection after insults, such as light-induced injury.14
CNTF IN ANIMAL MODELS OF DEGENERATION
As noted, CNTF is effective at slowing retinal degeneration in at least 12 animal models of RP in four different species, including mice with mutations in the PDE6b,15,16 rds-peripherin,15,17-19 rhodopsin,20 and nr genes15; transgenic rats expressing the P23H or S334ter mutations in rhodopsin19; Rdy cats21; and rcd1-PDE6b dogs.22 CNTF also prolongs photoreceptor survival in models of retinal degeneration caused by light-induced injury.15
The treatment effect of CNTF has been demonstrated by the increased numbers of photoreceptor nuclei in the outer nuclear layer of the retina,15,22 increased outer nuclear layer thickness,4,16,17 increased photoreceptor density,18 and improved integrity and organization of the inner and outersegments of photoreceptors4 in CNTF-treated eyes, compared to control eyes.
In addition to slowing the rate of photoreceptor cell loss, CNTF may be associated with regeneration of cone outer segments in rat eyes treated before significant photoreceptor degeneration has occurred.23 CNTF also alters gene expression in photoreceptors, as it causes uncoiling of heterochromatin and increased nuclear size in mouse rods.18,24
EFFECT OF CNTF ON RETINAL FUNCTION
High-dose CNTF has been associated with altered gene expression. Greater nuclear size and uncoiling of nuclear DNA in mouse photoreceptors have been reported by several groups.18,25 CNTF has also caused decreased retinal function, as measured by electroretinography (ERG) in a number of reports: after eight to nine months of treatment with CNTF, P23H rhodopsin and S334ter rhodopsin transgenic rats had significantly smaller dark-adapted and light-adapted ERG responses in the presence of histological rescue of photoreceptors.19 This adverse effect on retinalfunction was shown to be dose-dependent and, paradoxically, was inversely related to the number of rescued photoreceptors.18
Other measures of visual function, including luminance thresholds at the superior colliculus, visual acuity and behavioral tracking responses were also negatively affected in a dose-dependent manner in normal rat retinas.26 It was proposed that these effects could be due to CNTF-mediated downregulation of rhodopsin27,28 and the phototransduction machinery of rods,29 or perhaps due to an altered photoreceptor ene expression profile.19,24
However, a number of reports have also demonstrated that therapeutic doses of CNTF do not attenuate ERG esponses. Doses of CNTF that produced photoreceptor rescue in a rcd1 canine model of retinal degeneration22 were not leterious to ERG responses in normal rabbit eyes, and low-dose CNTF released at a rate of 5 ng/day increased rod esponses at very low intensity stimuli near threshold.30
A similar therapeutic effect of low-dose CNTF in the absence of ERG suppression was also shown in albino rats ith retinal degeneration after exposure to constant light.26 Thus, CNTF affects photoreceptor survival and retinal function in a dose-dependent manner, and there appear to be optimum doses of CNTF at which photoreceptor rescue is not associated with reduced retinal function.
MODE OF INTRAOCULAR DELIVERY
The slowly progressive nature of retinal degenerations creates a major treatment challenge to delivering neurotrophic ctors such as CNTF to the retina on a long-term basis. NTF is a protein that does not cross the blood-retinal barrier and has a short half-life in vivo.31 Systemic administration is associated with numerous side effects.32
In early animal studies, a single intravitreal injection of a modified recombinant CNTF protein was used to deliver CNTF,3,4,15 which produced short-term effects and inconsistent results across species.15 Later, more controlled and sustained delivery of CNTF was achieved by gene expression, with the use of a recombinant adeno-associated virusvector (rAAV).16-20 Photoreceptor survival was observed as long as eight and nine months after treatment in rat and use models of retinal degeneration.19
However, patients with retinal degenerations require treatment over a lifetime, and despite the promising survival effects of CNTF on photoreceptors, it was not evaluated for se in human eyes until a safer and more reliable mode ofdelivery than frequent intravitreal injections was designed.
Neurotech USA developed encapsulated cell technology (ECT), a strategy to allow sustained, targeted delivery of CNTF into the vitreous, with direct access to the retina on a long-term basis. The NT-501 en capsulated cell implant ntains immortalized RPE cells that have been engineered to release CNTF at a continuous rate for periods up to 18 months (Figure 1a).22 The RPE cells are contained within a semipermeable membrane, with 15-nm pores that provide access to oxygen and nutrients such as glucose but that protect the RPE cells from interaction with the host's immunesystem (Figure 1b).28
The NT-501 implant provides an especially attractive drug delivery system because it bypasses the need for systemic administration or repeated intraocular injections, and it provides long-term drug delivery. Pharmacokinetic data rom preclinical studies showed continued CNTF productionbeyond one year.33
The implant is surgically inserted through the pars lana into the vitreous cavity, and a titanium loop is sutured to the sclera.5 It does not obstruct the visual axis but canbe seen at the slit-lamp with the pupil dilated. Fur ther, the implant can be re trieved from the eye at any time, providing an added level of safety (Figure 1c).
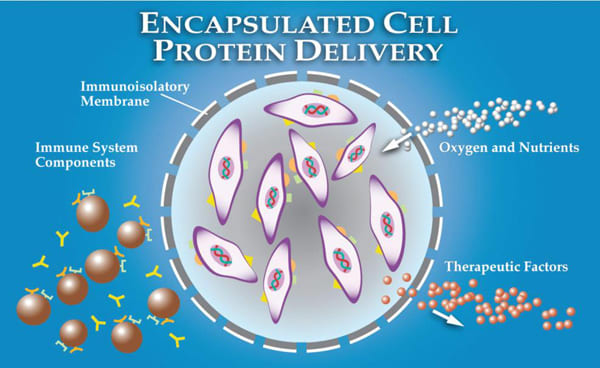
Figure 1a. NT-501 implant consists of genetically engineered cells encapsulated in a semipermeable membrane that produces CNTF. The implant has a suture loop at one end to anchor it to the sclera in the vitreoretinal body.
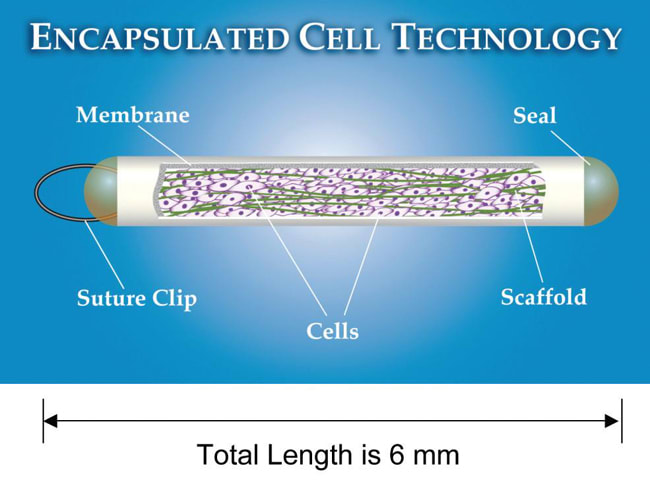
Figure 1b. The diffusive characteristics of the ECT membrane allow long-term cell survival by allowing influx of oxygen and nutrients, while simultaneously preventing direct contact of the encapsulated cells with the cellular and molecular elements of the immune system.
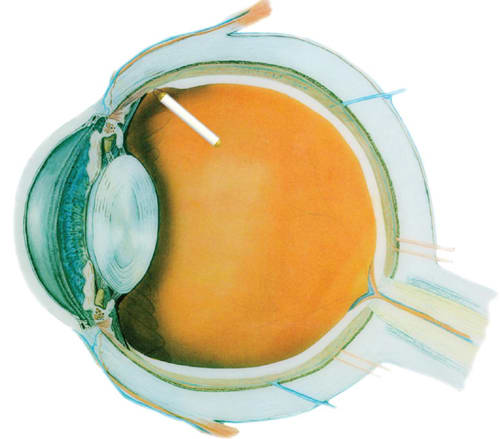
Figure 1c. Intraocular location of the surgically placed implant.
CLINICAL TRIALS
A phase 1 clinical trial34 was successfully ompleted in 2005, in whichNT-501 implants, containing human PE cells that were genetically engineered o secrete CNTF, were surgically laced for six months into the vitreous of 10 participants with advanced vision loss due to retinal degeneration. The implants secreted CNTF at 0.28 ±0.07 ng/day (low dose) and 1.53 ±0.54 ng/day (high dose).
Both doses were well tolerated without any systemic or ocular complications. Three of the seven eyes for which visualacuity could be measured by standard methods gained and maintained improved acuities of 10-15 letters, equivalent to two to three lines of improvement on Snellen acuitycharts. Only one participant had retinal function that was easurable with ERG, and in this patient, ERG responseswere reduced during the implant period, similar to results observed in animal studies. How ever, visual acuity improved while the NT-501 device was in the vitreous, and the reduced ERG responses recovered after the implant was removed.34
A 12-month, multicenter, randomized, controlled, phase 2 clinical trial was conducted on 51 participants with AMD with geographic atrophy.36 Although no statistically significant improvement in visual acuity was observed across the high-dose, low-dose and sham treatment groups, this trial showed a dose-dependent increase in outer retinal thickness and better stabilization of visual acuity in the high-dose group, compared with the low-dose and sham groups, in whom visual acuity declined over the 12-month study period.
Subgroup analysis showed that 100% of patients with ision better than or equal to 20/63 at baseline in the highdose CNTF group lost less than 15 letters of vision, compared with 55.6% of patients in the low-dose or sham groups (P=.033), suggesting that CNTF may slow vision loss due to atrophic AMD in patients with vision of 20/63 or better.35
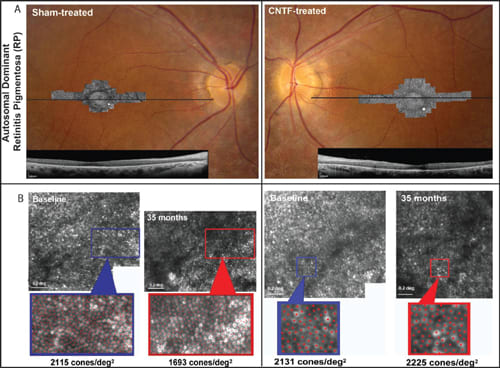
Figure 2a. Fundus photographs superimposed with adaptive optics SLO images and foveal horizontal SD-OCT scans in CNTF-treated and sham-treated eyes of a patient with retinitis pigmentosa. Horizontal lines: OCT scan location; white square on AOSLO images: retinal locations of density measures as shown in Figure 2b.
Figure 2b. Cone photoreceptor density measured using AOSLO images at baseline and post-treatment in sham and CNTF-treated eyes of the RP pa tient in Figure 2A (yellow squares in A). Red dots: cones identified for density analysis. White scale bars indicate 0.2º.
CNTF was also studied in two multicenter, randomized, controlled, phase 2 clinical trials of patients with nherited retinal degenerations. In these studies, the patients had symmetric retinal degeneration in each eye; one eye was randomly assigned to receive CNTF delivered by the NT-501 implant, while the other eye was assigned toreceive sham surgery with no CNTF. Patients were also randomly assigned to receive an implant that secreted either a high (20 ng/day) or low (5 ng/day) dose of CNTF. Patients were masked to which eye received the CNTF, and patients and doctors were masked to the dose of CNTF delivered.
One study (CNTF4; ClinicalTrials.gov number NCT00447980) evaluated the effect of CNTF in patients ith earlier stages of retinal degeneration, using visual field ensitivity changes over 12 months as the primary outcome measure. The other study (CNTF3; ClinicalTrials.gov mber NCT00447993) measured change in best-corrected visual acuity over 12 months as the primary outcome, in patients with later stages of retinal degeneration. In each of these studies, there were no serious adverse events, and the treatment was well tolerated, but no significant change was ob served in visual field sensitivity or visualacuity over the study period for either the CNTF-treated or sham-treated eyes.
Three patients from the CNTF4 trial were evaluated using adaptive optics scanning laser ophthalmoscopy (AOSLO), which allowed direct visualization of cones noninvasively (Figure 2).36 All three patients showed no significant differences in visual acuity, visual field sensitivity, or ERG amplitudes, between the sham and CNTF-treated eyes, over 30 months. However, cone spacing increased by 2.9%, and cone density decreased by 9% to 24% more in ham-treated eyes compared with CNTF-treated eyes.
Further, in one retinal region of one CNTF-treated eye, individual cones were tracked longitudinally using AOSLO nd showed no significant changes over 32 months. This novel method of evaluating outcomes showed less cone loss n CNTF-treated eyes, compared to contralateral shamtreated eyes, while conventional methods of measuring visual function did not show any significant differences. Because the natural history of retinal degenerations is suchthat it may take several years and significant photoreceptor loss before conventional methods can reliably measure a significant decline in visual function,37-39 AOSLO may provide a sensitive method of measuring disease progression and response to treatment before severe loss of photoreceptors has occurred.
CONCLUSION
In less than two decades, ciliary neurotrophic factor has emerged as a potent growth factor with potential applications for photoreceptor rescue, and there is substantialevidence that it reduces photoreceptor loss in several animal models of retinal degeneration. Encapsulated cell technology has provided an efficient, sustained method to deliver CNTF at a consistent dose on a long-term basis.
Although clinical trials to date have been limited in size and duration, results suggest CNTF may provide a safe and ffective treatment for retinal degenerations such as AMD with geographic atrophy as well as retinitis pigmentosa. Additional, larger studies, using high-resolution measures of retinal structure and function, are urgently needed to aluate CNTF further for the treatment of humans with etinal degenerations. RP
REFERENCES
1. Friedman DS, O'Colmain BJ, Muñoz B, Tomany SC, McCarty C, de Jong PT, Nemesure B, Mitchell P, Kempen J; Eye Diseases Prevalence Research Group. Prevalence of age-related macular degeneration in the United States. Arch Ophthalmol. 2004;122:564-572.
2. Hartong DT, Berson EL, Dryja TP. Retinitis pigmentosa. Lancet. 2006; 368:1795-1809.
3. Faktorovich EG, Steinberg RH, Yasumura D, Matthes MT, LaVail MM. Photoreceptor degeneration in inherited retinal dystrophy delayed by basic fibroblast growth factor. Nature. 1990;347:83-86.
4. LaVail MM, Unoki K, Yasumura D, Matthes MT, Yancopoulos GD, Steinberg RH. Multiple growth factors, cytokines, and neurotrophins rescue photoreceptors from the damaging effects of constant light. Proc Natl Acad Sci U S A. 1992;89:11249-11253.
5. MacDonald IM, Sauvé Y, Sieving PA. Preventing blindness in retinal disease: ciliary neurotrophic factor intraocular implants. Can J Ophthalmol. 2007;42:399-402.
6. Adler R, Landa KB, Manthorpe M, Varon S. Cholinergic neuronotrophic factors: intraocular distribution of trophic activity for ciliary neurons. Science. 1979;204:1434-1436.
7. Masiakowski P, Liu HX, Radziejewski C, Lottspeich F, Oberthuer W, Wong V, Lindsay RM, Furth ME, Panayotatos N. Recombinant human and rat ciliary eurotrophic factors. J. Neurochem. 1991;57:1003-1012.
8. Lam A, Fuller F, Miller J, Kloss J, Manthorpe M, Varon S, Cordell B. Sequence and structural organization of the human gene encoding ciliary neurotrophic factor. Gene. 1991;102:271-276.
9. Negro A, Corona G, Bigon E, Martini I, Grandi C, Skaper SD, Callegaro L. Synthesis, purification, and characterization of human ciliary neuronotrophic factor from E. coli. J Neurosci Res. 1991;29:251-260.
10. He C, Chen J, Ao S, Lu C. Preparation and a structure-function analysis of human ciliary neurotrophic factor. Neurosci Res. 1995;23:327-333.
11. Bazan JF. Neuropoietic cytokines in the hematopoietic fold. Neuron. 1991;7:197-208.
12. Ip NY, McClain J, Barrezueta NX, Aldrich TH, Pan L, Li Y, Wiegand SJ, Friedman B, Davis S, Yancopoulos GD The alpha component of the CNTF receptor is equired for signaling and defines potential CNTF targets in the adult and during development. Neuron. 1993;10:89-102.
13. Beltran WA, Zhang Q, Kijas JW, Gu D, Rohrer H, Jordan JA, Aguirre GD. Cloning, mapping, and retinal expression of the canine ciliary neurotrophic factor receptor alpha (CNTFRalpha). Invest Ophthalmol Vis Sci. 2003;44:3642-3649.
14. Boulton TG, Stahl N, Yancopoulos GD. Ciliary neurotrophic factor/leukemia inhibitory factor/interleukin 6/oncostatin M family of cytokines induces tyrosine phosphorylation of a common set of proteins overlapping those induced by other cytokines and growth factors. J Biol Chem. 1994;269:11648-11655.
15. Valter K, Bisti S, Gargini C, Di Loreto S, Maccarone R, Cervetto L, Stone J. Time course of neurotrophic factor upregulation and retinal protection against ight-induced damage after optic nerve section. Invest Ophthalmol Vis Sci. 2005;46:1748-1754.
16. LaVail MM, Yasumura D, Matthes MT, et al. Protection of mouse photoreceptors by survival factors in retinal degenerations. Invest Ophthalmol Vis Sci. 1998;39:592-602.
17. Cayouette M, Gravel C. Adenovirus-mediated gene transfer of ciliary neurotrophic factor can prevent photoreceptor degeneration in the retinal degeneration (rd) mouse. Human Gene Ther. 1997;8:423-430.
18. Cayouette M, Behn D, Sendtner M, Lachapelle P, Gravel C. Intraocular gene transfer of ciliary neurotrophic factor prevents death and increases responsiveness of rod photoreceptors in the retinal degeneration slow mouse. J Neurosci. 1998;18:9282-9293.
19. Bok D, Yasumura D, Matthes MT, Ruiz A, Duncan JL, Chappelow AV, Zolutukhin S, Hauswirth W, LaVail MM. Effects of adeno-associated virus-vectored ciliary eurotrophic factor on retinal structure and function in mice with a P216Lrds/peripherin mutation. Exp Eye Res. 2002;74:719-35.
20. Liang FQ, Aleman TS, Dejneka NS, Dudus L, Fisher KJ, Maguire AM, Jacobson SG, Bennett J. Long-term protection of retinal structure but not function using RAAV.CNTF in animal models of retinitis pigmentosa. Mol Ther. 2001;4:461-472.
21. Liang FQ, Dejneka NS, Cohen DR, Krasnoperova NV, Lem J, Maguire AM, Dudus L, Fisher KJ, Bennett J. AAV-mediated delivery of ciliary neurotrophic factor prolongs photoreceptor survival in the rhodopsin knockout mouse. Mol Ther. 2001;3:241-248.
22. Chong NH, Alexander RA, Waters L, Barnett KC, Bird AC, Luthert PJ. Repeated injections of a ciliary neurotrophic factor analogue leading to long-term photoreceptor survival in hereditary retinal degeneration. Invest Ophthalmol Vis Sci. 1999;40:1298-1305.
23. Tao W, Wen R, Goddard MB, Sherman SD, O'Rourke PJ, Stabila PF, Bell WJ, Dean BJ, Kauper KA, Budz VA, Tsiaras WG, Acland GM, Pearce-Kelling S, Laties AM, Aguirre GD. Encapsulated cell-based delivery of CNTF reduces photoreceptor degeneration in animal models of retinitis pigmentosa. Invest Ophthalmol Vis Sci. 2002;43:3292-3298.
24. Li Y, Tao W, Luo L, Huang D, Kauper K, Stabila P, Lavail MM, Laties AM, Wen R. CNTF induces regeneration of cone outer segments in a rat model of retinal degeneration. PLoS One. 2010;5:e9495.
25. Rhee KD, Ruiz A, Duncan JL, Hauswirth WW, Lavail MM, Bok D, Yang XJ. Molecular and cellular alterations induced by sustained expression of ciliary neurotrophic factor in a mouse model of retinitis pigmentosa. Invest Ophthalmol Vis Sci. 2007;48:1389-1400.
26. Bush RA, Lei B, Tao W, Raz D, Chan CC, Cox TA, Santos-Muffley M, Sieving PA. Encapsulated cell-based intraocular delivery of ciliary neurotrophic factor in normal rabbit: dose-dependent effects on ERG and retinal histology. Invest Ophthalmol Vis Sci. 2004;45:2420-2430.
27. McGill TJ, Prusky GT, Douglas RM, Yasumura D, Matthes MT, Nune G, Donohue- Rolfe K, Yang H, Niculescu D, Hauswirth WW, Girman SV, Lund RD, Duncan JL, LaVail MM. Intraocular CNTF reduces vision in normal rats in a dosedependent manner. Invest Ophthalmol Vis Sci. 2007;48:5756-5766.
28. Caffé AR, Söderpalm AK, Holmqvist I, van Veen T. A combination of CNTF and BDNF rescues rd photoreceptors but changes rod differentiation in the presence of RPE in retinal explants. Invest Ophthalmol Vis Sci. 2001;42:275-282.
29. Zeiss CJ, Allore HG, Towle V, Tao W. CNTF induces dose-dependent alterations in retinal morphology in normal and rcd-1 canine retina. Exp Eye Res. 2006; 82:395-404.
30. Wen R, Song Y, Kjellstrom S, Tanikawa A, Liu Y, Li Y, Zhao L, Bush RA, Laties AM, Sieving PA. Regulation of rod phototransduction machinery by ciliary neurotrophic factor. J Neurosci. 2006;26:13523-13530.
31. Bush RA, Lei B, Tao W, Raz D, Chan CC, Cox TA, Santos-Muffley M, Sieving PA. Encapsulated cell-based intraocular delivery of ciliary neurotrophic factor in normal rabbit: dose-dependent effects on ERG and retinal histology. Invest Ophthalmol Vis Sci. 2004;45:2420-2430.
32. Dittrich F, Thoenen H, Sendtner M. Ciliary neurotrophic factor: Phar maco kinetics and the acute-phase response in the rat. Ann Neurol. 1994;35:151-163.
33. ALS CNTF Treatment Study Group. A double blind placebo controlled clinical trial of subcutaneous recombinant human ciliary neurotrophic factor (rhcntf) in amyotrophic lateral sclerosis. ALS CNTF treatment study group. Neurology 1996;46:1244-1249
34. Thanos CG, Bell WJ, O'Rourke P, Kauper K, Sherman S, Stabila P, Tao W. Sustained secretion of ciliary neurotrophic factor to the vitreous, using the encapsulated cell therapy-based NT-501 intraocular device. Tissue Eng. 2004;10:1617-1622.
35. Sieving PA, Caruso RC, Tao W, Coleman HR, Thompson DJ, Fullmer KR, Bush RA. Ciliary neurotrophic factor (CNTF) for human retinal degeneration: phase I trial of CNTF delivered by encapsulated cell intraocular implants. Proc Natl cad Sci U S A. 2006;103:3896-3901. Epub 2006 Feb 27.
36. Zhang K, Hopkins JJ, Heier JS, Birch DG, Halperin LS, Albini TA, Brown DM, Jaffe GJ, Tao W, Williams GA. Ciliary neurotrophic factor delivered by encapsulated cell intraocular implants for treatment of geographic atrophy in age-related macular degeneration. Proc Natl Acad Sci U S A. 2011;108:6241-6245. Epub 2011 Mar 28.
37. Talcott KE, Ratnam K, Sundquist SM, Lucero AS, Lujan BJ, Tao W, Porco TC, Roorda A, Duncan JL. Longitudinal study of cone photoreceptors during retinal degeneration and in response to ciliary neurotrophic factor treatment. Invest Ophthalmol Vis Sci. 2011 Apr 6;52:2219-2226.
38. Fishman GA, Bozbeyoglu S, Massof RW, Kimberling W. Natural course of visual field loss in patients with type 2 Usher syndrome. Retina. 2007;27:601-608.
39. Grover S, Fishman GA, Anderson RJ, Alexander KR, Derlacki DJ. Rate of visual field loss in retinitis pigmentosa. Ophthalmology. 1997;104:460-465.
40. Iannaccone A, Kritchevsky SB, Ciccarelli ML, et al. Kinetics of visual field loss in Usher syndrome type II. Invest Ophthalmol Vis Sci. 2004;45:784-792.
Reema Syed, MD, is a postdoctoral scholar in the Department of Ophthalmology at the University of California, San Francisco. Jacque L. Duncan, MD, is a professor in the Department of Ophthalmology at the University of California, San Francisco. Neither author reports any financial interest in any of the products mentioned in this article. Dr. Duncan can be reached via e-mail at DuncanJ@vision.ucsf.edu. |