OCT INSIGHTS
Revealing Henle's Fiber Layer Using Spectral-domain OCT
CLINICAL APPLICATIONS OF ADVANCED IMAGING TECHNOLOGY
Brandon J. Lujan, MD
Ophthalmologists, and retinal specialists in particular, are unparalleled as adopters of new technology and innovations. Optical coherence tomography in particular appeals to our intuition because of how closely its images resemble the retinal histology inculcated into us from our residency training. However, things are not always as they appear.
Images from OCT are not derived simply from the anatomical structures present but rather are a direct consequence of the optical properties of the tissues being imaged. Perhaps nowhere else in the retina is this fact more striking than in the appearance of the obliquely oriented axons of the photoreceptors, which — along with their supporting Müller cells — make up Henle's fiber layer (HFL).
Joe Carroll, Robert Knighton, Austin Roorda and I describe this phenomenon in our paper, “Revealing Henle's Fiber Layer Using Spectral Domain Optical Coherence Tomography” in Investigative Ophthalmology & Visual Science (IOVS), published ahead of print on November 11, 2010. This paper is the first to demonstrate that, by purposefully entering the pupil through an eccentric position, HFL can be visualized reproducibly and distinctly. Building on a paper we presented at the 2010 ARVO conference, this publication demonstrates the variable OCT reflectivity of HFL across a variety of pupil entry positions (Figure 1) and provides an optical model for this phenomenon.
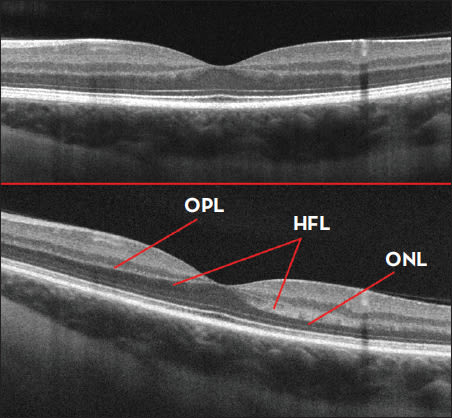
Figure 1. Horizontal SDOCT B-scan of a normal eye acquired under standard imaging conditions (top image). SDOCT of the same eye acquired through a horizontally eccentric pupil location (bottom image). Note the increased contrast of layers between the outer nuclear layer (ONL), Henle's fiber layer (HFL) and the outer plexiform layer (OPL).
HOW IT WORKS
The key to the optical changes in HFL reflectivity is found in the oblique orientation of these fibers in the healthy retina. In normal retinal development, HFL assumes this orientation as centrally migrating photoreceptors elongate their axons to maintain synapses with peripherally migrating cells of the inner retina during foveal development. These photoreceptor axons are filled with microtubules, whose optical properties have been studied extensively in the context of the retinal nerve fiber layer. These fibers have the property of reflecting light along a tight cone that is coaxial to the fiber itself and the shape of which is governed by the angle of incident light.
For our purposes, the reflected signal “seen” by SDOCT from HFL will increase as the beam is oriented more perpendicularly and similarly will actually become less intense than usual as the beam angle decreases. This phenomenon provides the optical contrast that distinguishes HFL from its surrounding layers.
One obvious difference in images derived from light entering (and returning) from an eccentrically located OCT beam position is the tilt of the B-scan. Clearly, the actual retinal anatomy is unchanged; however, there is an increased optical path length of light returning from the far side of the scanning beam compared to the path length of light returning from the near side. Consequently, the resulting image is rendered as a “tilted” B-scan. This tilt phenomenon is well known to OCT operators, so photographers will typically move the beam to a pupil entry position where the B-scan appears “flat” (ie, vertically symmetric) before acquiring a scan.
Under standard imaging conditions, the amount of light that is reflected back from HFL happens to be comparable to the outer nuclear layer, and consequently there is usually no significant contrast between the edges of the photoreceptor nuclei and the HFL axons. However, by orienting the beam angle more normally to the axons, there is an increased reflectivity because of very sensitive directional reflectance exhibited by the cylindrical axons of the photoreceptors.
REDEFINING HFL
Because of the inability to routinely visualize the interface between the photoreceptor nuclei and their axons, HFL has typically been considered to be part of the outer nuclear layer on SDOCT. Histologically, however, HFL has historically been regarded as part of the outer plexiform layer. Now that a means exists to generate optical contrast to HFL in vivo, it would increase precision and minimize confusion to regard HFL as a distinct retinal layer on SDOCT.
Indeed, the synapses present in the outer plexiform layer remain hyperreflective and can be easily distinguished from the hyporeflective HFL, as this part of the retina is relatively impervious to changes in angle of incidence (Figure 1). Furthermore, the thickness of HFL in vivo actually varies considerably by retinal eccentricity and between individuals, thus making historical generalizations about the contribution of the HFL imprecise. Now that a means exists to identify HFL, normative measurements of this distinct SDOCT layer can be accurately performed.
Clinical trials may derive a benefit from the ability to distinguish HFL, albeit indirectly. Indeed, the ability to recognize this layer would allow a more accurate measurement of the true thickness of outer nuclear layer, which contains the photoreceptor nuclei. Classical retinal degeneration research using animal models has primarily focused on the photoreceptor nuclei thickness as an endpoint. By optically differentiating the photoreceptor axons from their nuclei, this becomes possible in vivo. Measurement of the true outer nuclear layer thickness could therefore be used in clinical studies, as well as to understand progression of retinal disease.
In addition to visualization of HFL in normal eyes, the IOVS paper also provides examples of HFL reflectivity changes secondary to retinal pathology that cause outer retinal deformation, such as central serous retinopathy and drusen associated with dry age-related macular degeneration. Even under standard acquisition conditions, any upward deforming pathology will alter the orientation of light reflecting from HFL relative to the beam entry position (Figure 2).
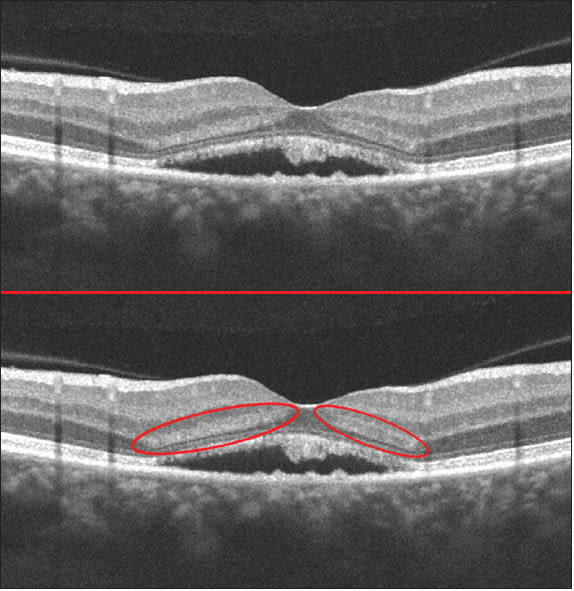
Figure 2. Vertical SDOCT B-scan of an eye with subretinal fluid acquired under standard imaging conditions (top). Note the presence of HFL hyper-reflectivity induced by the fluid such that the normally oblique HFL fibers are more perpendicular to the OCT entrance beam (bottom).
Furthermore, our paper contended that alterations of HFL reflectivity in cases of pathology were optical in nature and not likely a component of the pathological process by prospectively altering the beam entry position in a small sample of patients. Clearly, additional examples of these optical changes will be revealing.
Importantly, the fundamental optical principles that allow for imaging of Henle's fiber layer are independent of which OCT system is employed. In the IOVS paper, we used two different SDOCT systems and subsequently have noted the effect in several other SDOCT instruments as well. Indeed, this effect is even visible using time-domain OCT, though much less obvious. Similarly, frame averaging is not a requirement to generate these images, though, by minimizing speckle noise, the presence of alterations in HFL reflectivity are much more pronounced, and its use certainly facilitated the discovery of this method of HFL visualization.
Like all things in science and medicine, we see what we look for. Once aware of the appearance of Henle's fiber layer in normal patients imaged with spectral-domain OCT through an eccentric pupil position, the presence of this distinct retinal layer will become an obvious pattern detectable in several clinical and experimental situations. RP
Brandon J. Lujan, MD, performs retinal imaging research in the Roorda lab at the University of California at Berkeley, and is in practice with West Coast Retina in San Francisco. The images in this article were captured using the Cirrus HD-OCT from Carl Zeiss Meditec. Dr. Lujan receives research support from CZM and has previously received lecture honoraria from the company. He can be reached at blujan@berkeley.edu for correspondence or to request reprints of the original IOVS paper. |