Where Do PCV and RAP Fit in the Spectrum of AMD CNV Subtypes?
Determining lesion morphology provides for better diagnosis and treatment.
LARRY KOREEN, MD, PhD, MPH • MATTHEW W. HOLLAR, BS • SCOTT W. COUSINS, MD
Although neovascularization is present in a minority of cases of age-related macular degeneration, it is the cause of the majority of severe vision loss in AMD.1 Over the past decade, the spectrum of neovascular AMD (NVAMD) has been expanded beyond classic and occult choroidal neovascularization. Identification of morphologic CNV subtypes (predominantly capillary, branching arteriolar, or mixed patterns) and CNV variants (polypoidal choroidal vasculopathy [PCV] and retinal angiomatous proliferation [RAP]), suggest that the pathobiology and treatment of NVAMD is more complex than previously thought. Understanding these NVAMD phenotypes can help with informing patients of natural disease courses and with creating better-tailored disease management.
SUBTYPES: BEYOND CLASSIC AND OCCULT
Choroidal neovascularization is the growth of pathologic new blood vessels originating from the choriocapillaris invading into the subretinal pigment epithelium and/or the subretinal space. CNV formation initially involves capillary- like vessels that lack the structural integrity of more mature vessels and leak plasma or blood. Incipient capillaries can mature into more organized vessels with features of arteries and veins. CNV can ultimately evolve into fibrovascular scar tissue.
In “classic” CNV, fluorescein angiography reveals choroidal neovascularization with well-defined margins and homogeneous leakage of dye within the entire lesion. Most cases of CNV are “occult,” demonstrating irregular margins with heterogeneous leakage of fluorescein within the lesion. Originally, the differences in leakage pattern were assumed to be explained by the anatomic location of the CNV. In classic CNV, vessels were presumed to be located subretinally, having breached the RPE. Occult CNV was presumed to be located external to or below the RPE so that detection of fluorescein leakage would be dictated by the extent of the loss of integrity of overlying RPE. However, data from the Submacular Surgery Trials Research Group indicated a lack of strong correlation between fluorescein angiography pattern and anatomic localization of the CNV.2 Subsequent attempts to analyze two-dimensional reconstructions of the CNV and compare them with fluorescein angiography were somewhat limited by an inability to characterize the histological growth patterns of many of the CNV specimens.3
In the past decade, newer imaging technologies have revealed that the morphologic spectrum of pathologic new vessels in NVAMD (and presumably other diseases with CNV) is much more diverse than traditionally described using fluorescein angiography. Angiographic imaging technology employing indocyanine green (ICG) dye allows visualization of the morphology and the flow of the choroidal vasculature. Dynamic (or video) ICG angiography can image various components of pathologic vessels in NVAMD, including an intrachoroidal “feeder arteriole,” a subretinal or sub-RPE capillary network, and an intrachoroidal “draining venule.”
By ICG angiography, classic choroidal neovascularizations manifest a morphologic spectrum ranging from “capillary pattern” to “branching arteriolar pattern.” Capillary-pattern CNVs are mainly composed of capillaries and minimal short, small-caliber feeder arterioles (Figure 1). “Pure” branching arteriolar-pattern CNVs have minimal capillary morphology and instead are composed of long, large-caliber feeder arterioles with many branching arterioles. “Mixed-pattern” CNVs demonstrat features of both capillary and branching arteriolar types (Figure 2).
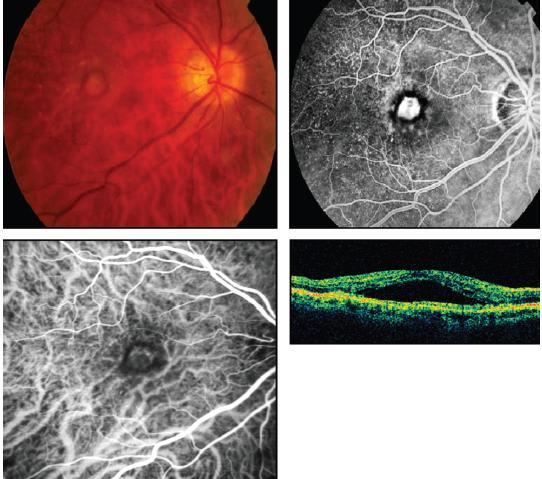
Figure 1. Fluorescein angiogram (upper right) and ICG angiogram (lower left) demonstrate a capillary pattern subtype of classic choroidal neovascularization. OCT shows subretinal fluid.
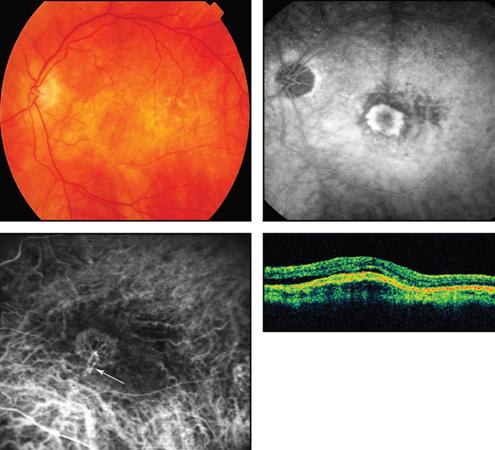
Figure 2. Fluorescein angiogram (upper right) and ICG angiogram (lower left) demonstrate a mixed pattern subtype of classic CNV, characterized by capillaries and an arteriolized feeder vessel and draining venule (white arrow).
Most CNVs likely begin with a capillary structure and then progress, at variable rates, through a maturation process in which small capillaries obtain a sheath of pericytes that stabilizes the incipient new vessels. In some cases, the new vessels become arteriolized by being surrounded with vascular smooth muscle and collagen.
The morphologic variability of CNV may be due to differences in the extent of this maturation. Furthermore, there is the possibility that, in certain cases, the final lesion type may result not from a stepwise progression across different vascular stages but rather from a predetermined vascular fate that from the outset is achieved via some potential express pathway.
Regardless, one emerging hypothesis is that capillary-dominated CNVs are exquisitely responsive to antivascular endothelial growth factor treatment, whereas branching arteriolar CNVs with large feeder vessels are less responsive, or even resistant, to anti-VEGF therapy.
Occult CNVs also demonstrate significant morphologic variation by dynamic ICG. As with classic CNV, about 40% of occult CNVs appear to demonstrate arteriolized vessels (Figure 3). Capillary-only subtypes of occult CNV are not very common. However, by dynamic ICG angiography, many cases of occult lesions manifest as unusual intrachoroidal vascular abnormalities (Figure 4), PCV or RAP. Thus, these types of occult lesions do not appear to be authentic choroidal neovascularization.
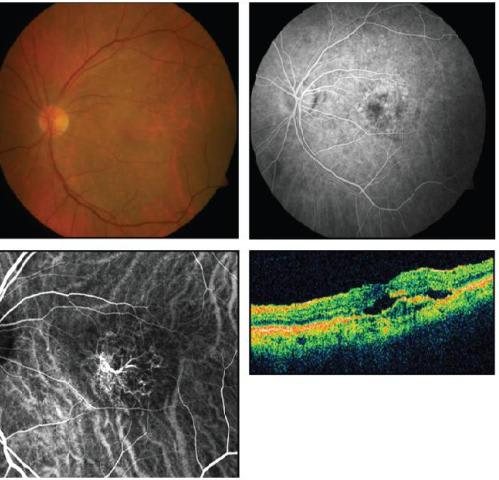
Figure 3. Fluorescein angiogram (upper right) shows a few areas of ill-defined leakage in this arteriolized pattern subtype of occult CNV. ICG angiography (lower left) demonstrates an arteriolized vascular complex. OCT shows intraretinal and subretinal fluid, retinal thickening, and RPE disruption.
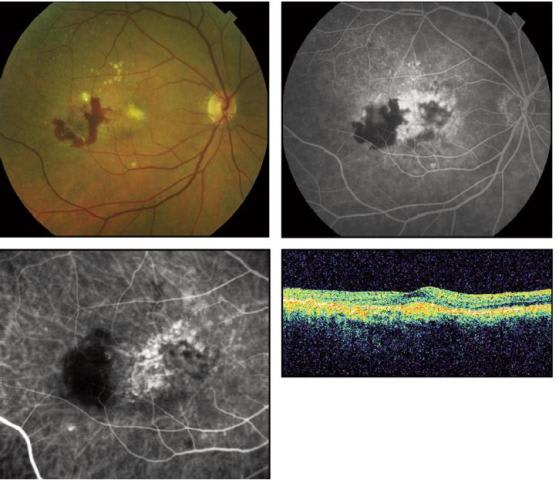
Figure 4. Fluorescein angiogram (upper right) demonstrates leakage from an intrachoroidal vascular abnormality, which is better visualized by ICG angiography (lower left).
VARIANTS OF PATHOLOGIC NEOVASCULARIZATION IN AMD
• Polypoidal Choroidal Vasculopathy. PCV is a form of intrachoroidal neovascularization that accounts for up to 50% of NVAMD in Asians4 and may be underdiagnosed inCaucasians. PCV is characterized by large-caliber feeder vessels perfusing intrachoroidal branching arteriolar networks that terminate in one or more intrachoroidal polypoidal structures, rather than typical subretinal capillaries of CNV (Figure 5). Clinical features include orange-red subretinal nodules corresponding to dilated polyp-like lesions and a frequent association with hemorrhagic or serous pigment epithelial detachments, retinal edema, or subretinal hemorrhage. PCV most commonly occurs in the peripapillary and macular regions. ICG angiography is required for diagnosis and visualization of PCV.
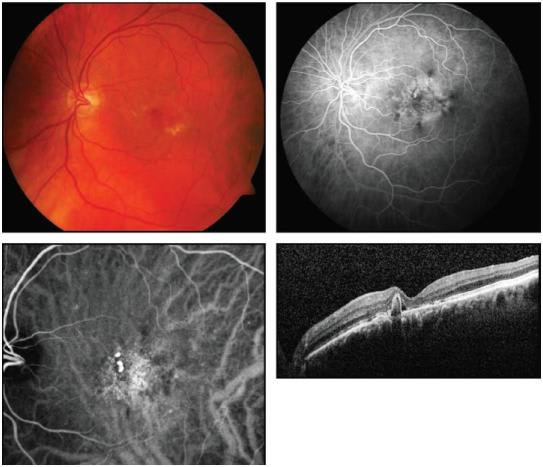
Figure 5. Early-phase fluorescein angiogram (upper right) shows areas of mottled hyperfluorescence. An ICG angiogram (lower left) better demonstrates polypoidal choroidal vasculopathy lesions.
The pathobiology of PCV is debated, especially whether it is a distinct disorder from CNV or a disease of similar biology but with different vascular morphology. Recent data suggest that PCV and AMD may be associated at the genotypic level.5 PCV histopathology demonstrates thin-walled, saccular polypoidal vessels that were initially regarded as being of venular origin. However, some excised specimens demonstrate the presence of both large choroidal arterioles and venules, disruption of inner elastic layer and arteriosclerotic changes of the blood vessels, and, in certain cases, an association between pericyte-like cells and branching arterioles.
The natural history and treatment of PCV is not well established. Anecdotal reports suggest that about half of cases demonstrate deterioration of vision in spite of treatment and that anti-VEGF therapy is less effective than for traditional CNV. Photodynamic therapy to the vascular components identified by ICG angiography has been shown to be effective.6 However, the long-term efficacy of PDT for PCV is not clear.7 Resistant or recurrent macular edema can occur after PDT, which may be a consequence of associated branching vascular networks and may be amenable to additional anti-VEGF therapy.7 The EVEREST study is randomized controlled trial for PCV comparing PDT with or without intravitreal ranibizumab (Lucentis, Genentech) vs intravitreal ranibizumab alone, with the primary endpoint being regression of the polypoidal lesion. Initial presented data have suggested PDT efficacy; a full report has not yet been published.
• Retinal Angiomatous Proliferation. RAP is another recently recognized and common variant of NVAMD that is characterized by the formation of pathologic new vessels within the retina that can secondarily invade into the subretinal space or into the choroid.8 It has been proposed that RAP lesions, also known as type 3 neovascularization, can involve vasculogenesis that initiates from the retina, from the choroid, or simultaneously from both.9,10 RAP requires the use of ICG angiography for definitive diagnosis. Frequency of the RAP variant among NVAMD cases appears to be approximately 15% to 20%;11,12 this variant may be less common in Asians. As with PCV, the pathobiology of RAP is unknown but there are genotyping data supporting an association among RAP, PCV and AMD.13
High-speed ICG angiography offers the best visualization of RAP lesions, particularly for localizing the retinal feeding arterioles and draining venules (Figure 6). Clinically, stage 1 RAP lesions present with intraretinal neovascularization with telangiectatic retinal capillaries and small angiomatous structures perfused by the retinal circulation. Stage 2 RAP lesions extend into the subretinal space to form subretinal neovascularization that often is associated with a serous PED. Most stage 2 RAP lesions are perfused by retinal arterioles and drained by retinal venules. In these cases, focal closure of the retinal feeding arteriole by thermal laser leads to loss of flow into the RAP lesion and disappearance of hemorrhage, retinal edema, and PED. In stage 3 RAP, it is presumed that a retinal-choroidal anastomosis (RCA) is formed. Untreated stage 3 RAP lesions can evolve into large fibrotic scars. The transition from purely retinal perfusion (stage 1-2 RAP) to choroidal perfusion (early stage 3 RAP) can be difficult to determine clinically.
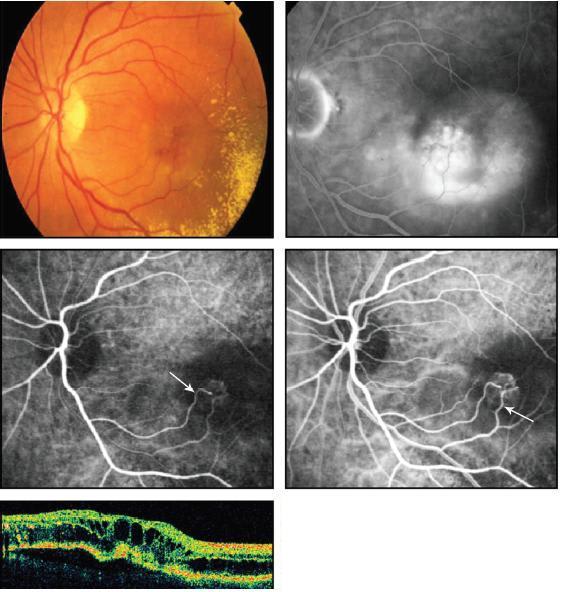
Figure 6. Late arteriovenous-phase fluorescein angiogram (upper right) shows substantial leakage. Early-phase ICG angiogram (middle left) of this RAP lesion demonstrates a capillary complex with an arteriolar feeder vessel ema nating from the retinal arterial circulation (white arrow). Arteriovenous-phase ICG angiogram (middle right) demonstrates a corresponding draining venule (white arrow) connecting to the retinal venous circulation. OCT reveals extensive intraretinal cystic edema and pigment epithelial detachment.
Prior to the advent of anti-VEGF therapy, many treatments for RAP were tried with variable results. Fortunately, anti-VEGF agents effectively control leakage,14 but rapid recurrences necessitate frequent injections. Focal thermal laser treatment of the afferent arteriole and extrafoveal angioma has been demonstrated to be as effective as salvage therapy for recurrences. In our case series of 16 patients with stage 1 (intraretinal lesion) and stage 2 (subretinal invasion) RAP, focal thermal laser therapy to the afferent arteriole demonstrated short-term resolution of retinal edema, with more durable effect associated with prior PDT/intravitreal triamcinolone acetonide.15
Various recent reports on treating RAP lesions with anti-VEGF therapy have shown positive results.16-18 Since RAP lesions respond well to anti-VEGF therapy, this suggests that the underlying pathobiology is strongly VEGF-mediated. Identification of the cellular source of VEGF in RAP (ie, retinal, RPE, or choroidal) to explain the origination of new vessel growth in the retina as differentiated from the choroidal origin in typical CNV remains speculative. Interestingly, it has been observed that unilateral RAP development essentially always predisposes to development of RAP lesions in the fellow eye.19 Advanced forms of the disease involving a vascularized RPE detachment and RCA are unlikely to respond well to any form of current treatment.
CONCLUDING REMARKS
The textbook paradigm that CNV is capillary neovascularization from the choroid has evolved with the advent of better imaging. It is now understood that there is a spectrum of NVAMD lesions (Figure 7), including the five primary types discussed here: predominantly capillary, branching arteriolar, or mixed pattern CNV subtypes; and the PCV and RAP variants of CNV. Of note, a recent study found that, based on different CNV characteristics, the majority of NVAMD patients would not have been con sidered eligible for some of the important anti-VEGF clinical trials. Therefore, results from these trials may not predict well the results from treating all NVAMD patients.12
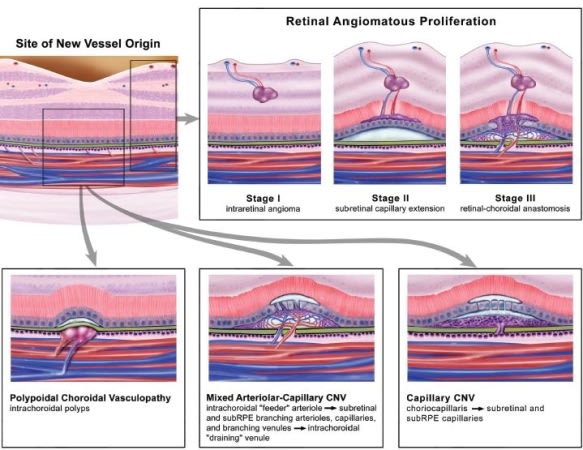
Figure 7. Hypothesized pathobiology of different forms of neovascular AMD.
This concept may help explain the varying treatment successes seen among patients with different lesion types on anti-VEGF or other therapies. Customized treatment plans based on lesion morphology will likely be most effective. As an example, we have found that the presence of an arteriolized CNV often precludes success with anti-VEGF therapy, but ICG-directed PDT targeted at the feeder arteriole can be effective, as it can be with PCV lesions. This observation is in contrast to RAP lesions, which appear exquisitely sensitive to anti-VEGF therapy. Ultimately, efforts at understanding and distinguishing NVAMD lesion types will allow for targeted research toward each entity and better patient care. RP
REFERENCES
1. Clemons TE, Milton RC, Klein R, et al 3rd. Risk factors for the incidence of Advanced Age-Related Macular Degeneration in the Age-Related Eye Disease Study (AREDS) AREDS report no. 19. Ophthalmology. 2005;112:533-539.
2. Grossniklaus HE, Miskala PH, Green WR, et al. Histopathologic and ultrastructural features of surgically excised subfoveal choroidal neovascular lesions: submacular surgery trials report no. 7. Arch Ophthalmol. 2005;123:914-921.
3. Group SSTR. Comparison of 2D reconstructions of surgically excised subfoveal choroidal neovascularization with fluorescein angiographic features: SST report No. 15. Ophthalmology. 2006;113:279 e271-279 e275.
4. Lim TH, Laude A, Tan CS. Polypoidal choroidal vasculopathy: an angiographic discussion. Eye (Lond). 2010;24:483-490.
5. Lima LH, Schubert C, Ferrara DC, et al. Three major loci involved in agerelated macular degeneration are also associated with polypoidal choroidal vasculopathy. Ophthalmology. 2010;117:1567-1570.
6. Eandi CM, Ober MD, Freund KB, Slakter JS, Yannuzzi LA. Selective photodynamic therapy for neovascular age-related macular degeneration with polypoidal choroidal neovascularization. Retina. 2007;27:825-831.
7. Kurashige Y, Otani A, Sasahara M, et al. Two-year results of photodynamic therapy for polypoidal choroidal vasculopathy. Am J Ophthalmol. 2008;146:513-519.
8. Yannuzzi LA, Negrao S, Iida T, et al. Retinal angiomatous proliferation in age-related macular degeneration. Retina. 2001;21:416-434.
9. Freund KB, Ho IV, Barbazetto IA, et al. Type 3 neovascularization: the expanded spectrum of retinal angiomatous proliferation. Retina. 2008;28:201-211.
10. Yannuzzi LA, Freund KB, Takahashi BS. Review of retinal angiomatous proliferation or type 3 neovascularization. Retina. 2008;28:375-384.
11. Cohen SY, Creuzot-Garcher C, Darmon J, et al. Types of choroidal neovascularisation in newly diagnosed exudative age-related macular degeneration. Br J Ophthalmol. 2007;91:1173-1176.
12. George S, Cooke C, Chakravarthy U. Exudative AMD subtypes and eligibility for treatment with ranibizumab. Eye (Lond). 2010;24:1247-1251.
13. Hayashi H, Yamashiro K, Gotoh N, et al. CFH and ARMS2 Variations in Agerelated Macular Degeneration, Polypoidal Choroidal Vasculopathy, and Retinal Angiomatous Proliferation. Invest Ophthalmol Vis Sci. 2010:Epub ahead of print.
14. Meyerle CB, Freund KB, Iturralde D, et al. Intravitreal bevacizumab (Avastin) for retinal angiomatous proliferation. Retina. 2007;27:451-457.
15. Bearelly S, Espinosa-Heidmann DG, Cousins SW. The role of dynamic indocyanine green angiography in the diagnosis and treatment of retinal angiomatous proliferation. Br J Ophthalmol. 2008;92:191-196.
16. Engelbert M, Zweifel SA, Freund KB. "Treat and extend" dosing of intravitreal antivascular endothelial growth factor therapy for type 3 neovascularization/retinal angiomatous proliferation. Retina. 2009;29:1424-1431.
17. Hemeida TS, Keane PA, Dustin L, Sadda SR, Fawzi AA. Long-term visual and anatomical outcomes following anti-VEGF monotherapy for retinal angiomatous proliferation. Br J Ophthalmol. 2010;94:701-705.
18. Saito M, Shiragami C, Shiraga F, Kano M, Iida T. Comparison of intravitreal triamcinolone acetonide with photodynamic therapy and intravitreal bevacizumab with photodynamic therapy for retinal angiomatous proliferation. Am J Ophthalmol. 2010;149:472-481 e471.
19. Gross NE, Aizman A, Brucker A, Klancnik JM, Jr., Yannuzzi LA. Nature and risk of neovascularization in the fellow eye of patients with unilateral retinal angiomatous proliferation. Retina. 2005;25:713-718.
Larry Koreen, MD, PhD, MPH, is a medical retina fellow at the Duke University Eye Center in Durham, NC. Matthew W. Hollar, BS, is a medical student at Duke. Scott W. Cousins, MD, is Robert Machemer Professor of Ophthalmology and Immunology, Vice Chair for Research and Director of the Center for Macular Diseases at Duke. Dr. Cousins cites minimal financial interest as a consultant for Heidelberg Engineering and Genentech. The other authors report no financial interest. Dr. Cousins can be reached at scott.cousins@duke.edu. |