Is There a Future for siRNA in AMD?
Despite recent setbacks, research bodes well for this innovative approach.
SUSAN S. M. CHANG MD, BS, MPH · R. C. ANDREW SYMONS, MD, BS, PhD
Small interfering RNA (siRNA) molecules fascinate clinicians and scientists as a means of turning off disease-causing genes. They offer a targeted approach to physiological manipulation without the difficulty of developing, synthesizing, and purifying a monoclonal antibody or a small molecule. siRNAs have sequence-specific effects on messenger RNA (mRNA), which lead to inhibition of gene expression. (Messenger RNA is copied from a cell's DNA sequence and is used by cells as the code for protein synthesis.) siRNA technology has already become a standard laboratory tool for investigating gene function, and clinical trials are under way in fields as diverse as oncology, infectious disease, and neurology. There has been great interest in the potential of siRNA to treat ocular disease, especially given the ability to deliver siRNA directly to the site of interest.
Two major trials of siRNAs for age-related macular degeneration (AMD) have recently been closed down. This raises the question: Does siRNA have a future in the treatment of AMD?
Susan S. M. Chang, MD, BS, MPH, will begin a pediatrics residency at the Kansas University Medical Center (KUMC) in July. R. C. Andrew Symons, MD, BS, PhD, is assistant professor of ophthalmology at KUMC. Dr. Chang has no financial disclosures to make. Dr. Symons was involved in the phase 1 trial of siRNA-027. Dr. Symons may be reached via e-mail at asymons@kumc.edu. |
WHAT IS SIRNA?
Small interfering RNAs are short, double-stranded RNA molecules that occur in yeast, animals, and plants. RNA interference (RNAi) is the sequence-specific inhibition of gene expression by endogenous or exogenous RNAs, approximately 20 to 30 nucleotides in length.1
The work of siRNA is largely performed through the RNA-induced silencing complex (RISC). A siRNA joins with specific protein components to form the RISC, which directly cleaves mRNAs that have perfect sequence complementarity to the siRNA (Figure 1). Translational repression and RNA degradation can also occur when there is more limited complementarity between siRNA and a target mRNA.
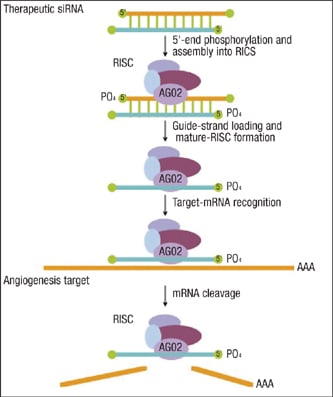
Figure 1. siRNA joins with specific protein components to form RISC, which cleaves mRNAs.
SIRNAS THAT HAVE BEEN TESTED IN AMD
Three siRNA treatments have been or are under clinical investigation in AMD. Figure 2 summarizes the biological pathways that these medications are designed to inhibit.
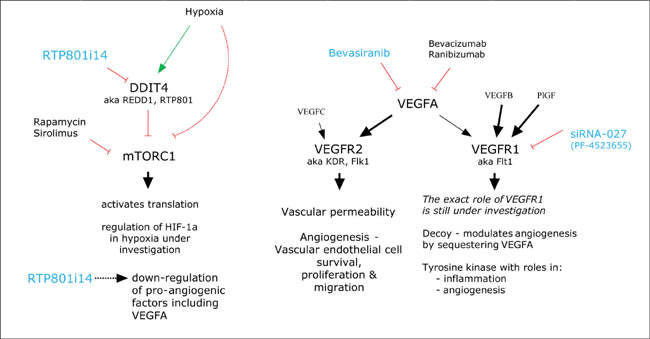
Figure 2. Biological pathways that siRNAs are designed to inhibit.
■ Bevasiranib. Bevasiranib, which was developed by OPKO Health, targets vascular endothelial growth factor (VEGF) A. When delivered by subretinal injection, it has been demonstrated to suppress laser-induced CNV in mice.2 Phase 1 and 2 trials investigating the safety and efficacy of the compound gave satisfactory results and a phase 3 trial, COBALT (Combining Bevasiranib And Lucentis) was started. This last study aimed to enroll 330 participants, to be randomized between 3 different treatment arms. The study was to last 2 years. The comparison arm consisted of ranibizumab injections every 4 weeks, as in the MARINA and ANCHOR trials. The other 2 arms consisted of 3 initial ranibizumab injections every 4 weeks, with bevasiranib injections at weeks 2, 6, and 12. These 2 arms differed after week 12. Thereafter, bevasiranib injections were administered either every 8 weeks or every 12 weeks. (Figure 3) This trial was halted in March 2009 by its data-monitoring committee. Although it appeared that bevasiranib had biological activity when used in conjunction with ranibizumab, it became apparent that the primary endpoint of the trial was unlikely to be met. There were no safety concerns.
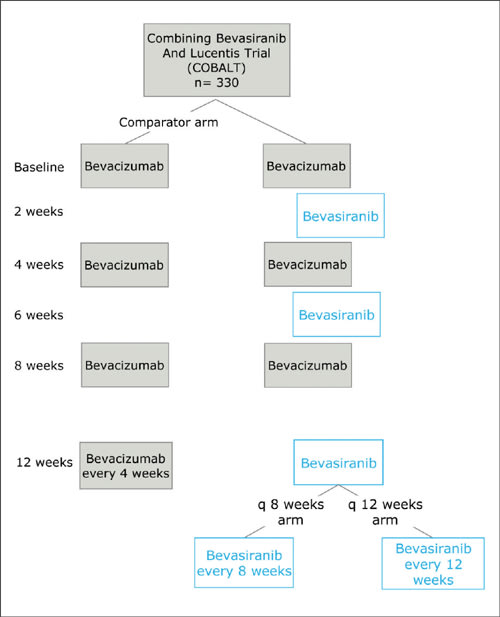
Figure 3. The COBALT study model.
The role of bevasiranib in diabetic macular edema has also been tested in a phase 2 trial where patients were randomized between different doses of the compound. This trial has now been completed, but the results are not yet available.
OPKO is now working on a siRNA that spares the antiangiogeneic3 VEGFA isoform VEGFA165b, and announced on Feb. 10, 2009, that it plans to present data on this agent at the ARVO meeting in May 2009.
■ siRNA-027. Developed by siRNA Therapeutics, which was taken over by Merck in 2007, siRNA-027 has since been renamed AGN211745. In a bold move, siRNA Therapeutics developed siRNA-027 to target VEGF receptor 1 (VEGFR1). VEGF receptor 2 (VEGFR2) is thought to be the main receptor that drives pathological angiogenesis. It has effects on vascular permeability, as well as on endothelial cell survival, proliferation, and migration. The role of the VEGFR1 is not completely understood, although work with genetically modified animals suggests that it transduces signals involved in inflammation and angiogenesis. However, there is also some evidence that VEGFR1 may function as a decoy receptor, meaning that it binds up VEGFA so that it does not have access to VEGFR2.4 If this is true, then silencing expression of VEGFR1 might even be proangiogenic.
Despite these theoretical concerns about targeting VEGFR1, siRNA Therapeutics invested in developing a siRNA against the gene. The company chose its lead compound based on ability to inhibit corneal neovascularization in a model system, providing proof of the drug's antiangiogenic function. Dr. J. Shen at the Wilmer Eye Institute tested siRNA-027 in a mouse model of laser-induced CNV and found that it was effective in reducing the area of choroidal neovascular membranes.5 Interestingly, the inverted control siRNA produced the same effect as the vehicle alone, failing to inhibit the neovascularization. Dr. Jayakrishna Ambati's group has shown that some siRNAs inhibit angiogenesis due to activation of toll-like receptor 3 (TLR3), rather than through specific gene silencing.6 Drs. Ambati and Shen could both be correct. Dr. Shen's control siRNA may have been one which does not stimulate TLR3.
A phase 1 and 2 dose-escalation trial was performed at the Wilmer Eye Institute and Cleveland Clinic. The trial suggested that the agent was efficacious. A further phase 2 trial was commenced in a partnership with Allergan. This trial randomized patients between 4 treatment arms. The active comparator consisted of 3 ranibizumab injections at monthly intervals. The 3 experimental arms consisted of different doses of the study medication to be administered 3 times at monthly intervals. This compound failed to meet an efficacy hurdle and Allergan and Merck are no longer collaborating. Merck may seek other opportunities for use of this compound.
■ RTP801i-14. RTP801i-14, or PF-04523655, is a siRNA developed by Quark and now licensed to Pfizer. It targets the DNA-damage-inducible transcript 4 (DDIT4) gene, also known as REDD1 and RTP-801. RTP-801 was first described by Quark as a result of a program for discovery of genes involved in hypoxia.7 An initial examination of DDIT4's role shows that it is a repressor of the activity of the mammalian target of rapamycin complex 1 (mTORC1). mTORC1 operates in multiple ways to activate protein translation in energy- and oxygen-replete states. Although in general its activity is inhibited in hypoxia, it has gene-specific effects on increasing the expression of HIF-1α and VEGFA. The modulation of these effects by hypoxia is still under investigation. So, does silencing a gene whose product inhibits a positive regulator of HIF-1α function make sense in retinal vascular disease? If that were the entire story, then it would not make sense. However, the regulation of mTOR is very complex, and Quark seems to have data that show multiple ways in which silencing of DDIT4 is beneficial.
Quark's Web site (www.quarkpharma.com/qbi-en/products/53/) notes that the RTP801 gene has been demonstrated to play a role in choroidal neovascularization in laboratory animal studies. These studies have included genetically modified "knock-out" mice, as well as intravitreal injection of RTP801i-14. The Web site also claims that RTP801i-14 led to "induction of expression of anti-angiogenic and neurotrophic factors." The induction of neurotrophic factors suggests that it is possible that RTP801i-14 may offer some protection against apoptosis. Indeed, Peter Kaiser, MD, showed data at the Retinal Physician Symposium in the Bahamas in March 2009 that the use of RTP801i-14 reduced retinal apoptosis in a mouse model.
Phase 1 studies of RTP801i-14 in neovascular AMD have been completed. There were no safety concerns and, in particular, no evidence of ocular inflammation or retinal toxicity. Phase 2 studies of this compound in the treatment of neovascular AMD and diabetic macular edema have been planned. The diabetic macular edema study has already recruited patients.
The AMD study is known as "Multicenter Study For Age Related Macular Degeneration Comparing An Investigational Drug PF-04523655 Versus Lucentis In The Treatment Of Subjects With Choroidal Neovascularization" or MONET. At the time of writing, www.clinicaltrials.gov listed this as a randomized trial where patients were to be randomized between receiving monthly injections of 1 of 3 different doses of the study drug or of the active comparator compound, ranibizumab. Since the COBALT investigators could not get an effect similar to ranibizumab with a VEGFA silencing siRNA, it will be interesting to see whether a siRNA targeting a gene that is not directly in the VEGF pathway can yield similar results to ranibizumab treatment. Perhaps it would be better for Pfizer and Quark to design a study to determine whether RTP801i-14 in addition to ranibizumab is more efficacious than the use of ranibizumab alone.
The DME study that is already recruiting is known as DEGAS, and it seeks to compare the efficacy of 3 different doses of intravitreal RTP801i-14 with that of focal/grid laser photocoagulation. Efficacy will be measured at 24 and 36 months in terms of visual acuity change and changes in fluorescein angiographic leakage and optical coherence tomography measurements.
SIRNA IN THE LABORATORY
As mentioned earlier, siRNA has become an important research tool. An example of laboratory work using siRNA that may yield a new therapeutic target in managing CNV is from Dr. W. Hauswirth's group in Gainesville, FL. This work investigated whether reactive oxygen species produced by the NADPH oxidase play a role in CNV development. A viral vector expressing a siRNA against NADPH oxidase subunit p22phox was injected under mouse retinas and was subsequently demonstrated to inhibit the development of laser induced CNV.8 Although the first 2 siRNAs in clinical trials in AMD may have finished in failure, there are likely to be more agents on the horizon.
■ Safety of siRNA in AMD. Although all studies have suggested that the intravitreal injection of siRNA compounds is safe, there are important theoretical concerns.
■ Could siRNA Cause Progression of Geographic Atrophy? A retina-specific issue arises from recent genetic studies of geographic atrophy in dry AMD.9 A variant (at the single nucleotide polymorphism rs3775291) in the TLR3 gene has been associated with the presence of geographic atrophy. In addition, cultured human retinal pigment epithelial cells that were homozygous for the 412Leu variant (which is encoded by the C allele at rs3775291) were susceptible to cell death induced by a synthetic long double-stranded RNA (dsRNA) molecule that activates TLR3. It is possible that patients carrying this variant will prove susceptible to progression of geographic atrophy if they are treated with siRNA.
■ Could siRNA Overwhelm Cellular Metabolism? There is no evidence that siRNAs in the doses used in clinical trials have overwhelmed the RNAi machinery of the retina. However, a study of the use of short hairpin RNA as a means of inducing RNAi in the livers of adult mice found cases of morbidity and mortality associated with downregulation of liver microRNAs. This study suggested that it is possible to oversaturate cellular small RNA pathways.10
■ Are siRNAs even specific? siRNAs unfortunately do not act purely against the target they are designed to block. Nor do they act entirely by RNA interference.
Experiments using microarrays to determine mRNA expression on a genome-wide level have demonstrated widespread off-target gene regulation.11 Sequence analysis of the off-target genes regulated most rapidly has shown that they contain either a core of 14 to 15 similar nucleotides in the central region of the siRNA sequence or an area of 9 nucleotides of similarity at the 3’ end of the siRNA. Less rapidly regulated genes share much smaller degrees of sequence similarity with the siRNA. Subsequently, a chemical modification that can reduce off-target effects has been demonstrated.12
As mentioned previously, data from Dr. Ambati's group suggest that the antiangiogenic effect of siRNAs 21 nucleotides or longer is a class effect rather than a sequence-specific effect.6 This effect was TLR3 on the cell surface, resulting in induction of interferon-gamma and interleukin-12. Intracellular toll-like receptors on plasmacytoid dendritic are also able to recognize siRNAs and induce interferon-alpha.13 It is understood that RTP-801i14 is shorter than 21 nucleotides and does not activate TLR3.
EFFICACY
Because of the great promise of siRNAs, a very substantial amount has been learned about how to optimize their function. Principles to aid incorporation of siRNA molecules into the RISC and to direct siRNA molecules toward mRNA sequences that are likely to be susceptible to cleavage have been developed.1 Chemical modifications have been developed that do not interfere with siRNA function but allow for greater nuclease resistance, greater serum stability, increased cellular uptake, reduced passenger strand activity, and decreased activation of toll-like receptors. All of these factors should be expected to increase efficacy.
Unfortunately there is not a great deal of information available concerning activity of siRNAs in the retina and retinal pigment epithelium, especially in humans. There are data suggesting that the agents are efficacious in laboratory rodent models. However, other evidence for predicting efficacy is scant. A study of subretinal injections of a vector carrying enhanced green fluorescent protein (EGFP) in concert with a siRNA against EGFP showed that the siRNA suppressed EGFP at 48 to 60 hours postinjection.2 The same investigators found similar results for suppression of expression of human VEGF in mice. Radio-labeled bevasiranib has been demonstrated to be present in the retina of Dutch-belted rabbits 168 hours after intravitreal injection.14 These studies also demonstrated that intact bevasiranib was present 24 hours after injection. These data from short-term experiments are insufficient to enable a prediction that siRNA technology will ever allow time between intravitreal injections to be drawn out beyond the 4 to 5 weeks commonly seen with monoclonal antibodies. The closure of recent clinical trials make the outlook even more pessimistic.
THE FUTURE OF SIRNAS
At this stage it is too early to predict the role that siRNAs will have in medical practice in the future. Much research has been done into the design, chemical modification, and delivery of siRNAs, and improved control over these factors should lead to better pharmaceutical products. They have become an important research tool, and therefore new compounds with enticing properties will continue to be developed. However, many questions remain concerning the efficacy and duration of effect of these compounds in the eye, and while many of the pertinent data remain unpublished, it is difficult to formulate an opinion about the ultimate fate of this field. RP
REFERENCES
- Castanotto D, Rossi JJ. The promises and pitfalls of RNA-interference-based therapeutics. Nature. 2009;457:426-433.
- Reich SJ, Fosnot J, Kuroki A, Tang W, Yang X, Maguire AM, Bennett J, Tolentino MJ. Small interfering RNA (siRNA) targeting VEGF effectively inhibits ocular neovascularization in a mouse model. Mol Vis. 2003;9:210-216.
- Konopatskaya O, Churchill AJ, Harper SJ, Bates DO, Gardiner TA. VEGF165b, an endogenous C-terminal splice variant of VEGF, inhibits retinal neovascularization in mice. Mol Vis. 2006;12:626-632.
- Fischer C, Mazzone M, Jonckx B, Carmeliet P. FLT1 and its ligands VEGFB and PlGF: drug targets for anti-angiogenic therapy? Nat Rev Cancer. 2008;8:942-956.
- Shen J, Samul R, Silva RL, Akiyama H, Liu H, Saishin Y, Hackett SF, Zinnen S, Kossen K, Fosnaugh K, Vargeese C, Gomez A, Bouhana K, Aitchison R, Pavco P, Campochiaro PA. Suppression of ocular neovascularization with siRNA targeting VEGF receptor 1. Gene Ther. 2006;13:225-234.
- Kleinman ME, Yamada K, Takeda A, Chandrasekaran V, Nozaki M, Baffi JZ, Albuquerque RJ, Yamasaki S, Itaya M, Pan Y, Appukuttan B, Gibbs D, Yang Z, Kariko K, Ambati BK, Wilgus TA, DiPietro LA, Sakurai E, Zhang K, Smith JR, Taylor EW, Ambati J. Sequence- and target-independent angiogenesis suppression by siRNA via TLR3. Nature. 2008;452:591-597.
- Shoshani T, Faerman A, Mett I, Zelin E, Tenne T, Gorodin S, Moshel Y, Elbaz S, Budanov A, Chajut A, Kalinski H, Kamer I, Rozen A, Mor O, Keshet E, Leshkowitz D, Einat P, Skaliter R, Feinstein E. Identification of a novel hypoxiainducible factor 1-responsive gene, RTP801, involved in apoptosis. Mol Cell Biol. 2002;22:2283-2293.
- Li Q, Dinculescu A, Shan Z, Miller R, Pang J, Lewin AS, Raizada MK, Hauswirth WW. Downregulation of p22phox in retinal pigment epithelial cells inhibits choroidal neovascularization in mice. Mol Ther. 2008;16:1688-1694.
- Yang Z, Stratton C, Francis PJ, Kleinman ME, Tan PL, Gibbs D, Tong Z, Chen H, Constantine R, Yang X, Chen Y, Zeng J, Davey L, Ma X, Hau VS, Wang C, Harmon J, Buehler J, Pearson E, Patel S, Kaminoh Y, Watkins S, Luo L, Zabriskie NA, Bernstein PS, Cho W, Schwager A, Hinton DR, Klein ML, Hamon SC, Simmons E, Yu B, Campochiaro B, Sunness JS, Campochiaro P, Jorde L, Parmigiani G, Zack DJ, Katsanis N, Ambati J, Zhang K. Toll-like receptor 3 and geographic atrophy in age-related macular degeneration. N Engl J Med. 2008;359:1456-1463.
- Grimm D, Streetz KL, Jopling CL, Storm TA, Pandey K, Davis CR, Marion P, Salazar F, Kay MA. Fatality in mice due to oversaturation of cellular microRNA/short hairpin RNA pathways. Nature. 2006;441:537-541.
- Jackson AL, Bartz SR, Schelter J, Kobayashi SV, Burchard J, Mao M, Li B, Cavet G, Linsley PS. Expression profiling reveals off-target gene regulation by RNAi. Nat Biotechnol. 2003;21:635-637.
- Jackson AL, Burchard J, Leake D, Reynolds A, Schelter J, Guo J, Johnson JM, Lim L, Karpilow J, Nichols K, Marshall W, Khvorova A, Linsley PS. Position-specific chemical modification of siRNAs reduces "off-target" transcript silencing. RNA. 2006;12:1197-205.
- Hornung V, Guenthner-Biller M, Bourquin C, Ablasser A, Schlee M, Uematsu S, Noronha A, Manoharan M, Akira S, de Fougerolles A, Endres S, Hartmann G. Sequence-specific potent induction of IFN-alpha by short interfering RNA in plasmacytoid dendritic cells through TLR7. Nat Med. 2005;11:263-270.
- Dejneka NS, Wan S, Bond OS, Kornbrust DJ, Reich SJ. Ocular biodistribution of bevasiranib following a single intravitreal injection to rabbit eyes. Mol Vis. 2008;14:997-1005.