The State of Gene Therapy With Viral Vectors for AMD
A look at how new modalities might transform our approach to drug delivery.
HANI SALEHI-HAD, MD · JOAN W. MILLER, MD
Age-related macular degeneration (AMD) is the leading cause of vision loss in people in the developed world over the age of 50.1,2 The neovascular or "wet" form of the disease, characterized by the development of choroidal neovascular membranes (CNV), is the main cause of visual impairment in macular degeneration.3,4 As such, the treatment of ocular neovascularization has been under intense investigation during the past 2 decades. As the treatment paradigm has shifted from laser photocoagulation to targeted antineovascular pharmaceutical agents, the route of delivery of these agents has become increasingly important. Gene therapy is an attractive option as a delivery vehicle. This article will focus on the different gene therapeutic approaches under development for AMD.
ADVANTAGE OF GENE THERAPY OVER CURRENT THERAPIES IN CLINICAL PRACTICE
With the advent of antineovascular pharmacotherapy, the visual prognosis of patients with wet AMD has improved significantly.5-7 However, the current standard-of-care therapies require monthly intravitreal injections by a retina specialist due to their short half-life in the vitreous.8 Besides the logistic difficulties and the patient's discomfort, it also puts the patient at risk for cataract formation, endophthalmitis, vitreous hemorrhage, and retinal detachment. Thus, there is a great need for alternative means of delivering antineovascular therapy to the retina. Gene therapy provides a means for local sustained delivery of therapeutic agents following a single intraocular injection.
Hani Salehi-Had, MD, is senior resident in the Department of Ophthalmology at Harvard Medical School's Massachusetts Eye and Ear Infirmary (MEEI) in Boston. Joan W. Miller, MD, is Henry Willard Williams Professor of Ophthalmology Chief and Chair of the Department of Ophthalmology at Harvard/MEEI. Dr. Miller has performed ad hoc consulting for Genzyme and has minimal financial interest. Dr. Salehi-Had has no financial interests to disclose. Dr. Salehi-Had can be reached via e-mail at Hani_Salehi-Had@meei.harvard.edu. |
DELIVERY VECTORS
The eye is an ideal organ for gene therapy utilizing viral vectors.9 The relative immune privilege of the eye reduces the chances of reactive inflammation;10,11 the small volume of the eye allows for local delivery of a high concentration of viral vectors with minimal systemic uptake; and the phagocytic nature of the retinal pigment epithelium (RPE) cells results in active uptake of viral particles after subretinal injection.9
Adeno-associated virus (AAV) or lentivirus vectors have been used extensively for intraocular gene therapy in rodents and nonhuman primates, and they have the advantage of being nonpathogenic, having low toxicity, showing long-term persistence, and engendering minimal immunogenicity.12-16 Intravitreal injection of AAV vector mainly results in the transduction of the ganglion cells,14,16 whereas subretinal administration results in the transduction of the RPE and the photoreceptors.12,15,17 Bovine immunodeficiency lentivirus vector similarly has been used for subretinal gene delivery in mice.18
Recombinant adenovirus vectors that are incapable of replicating have been used in intraocular gene therapy and have shown long-term persistent gene expression of up to 6 months in the RPE after subretinal delivery.19,20 The only 2 published ocular gene therapy phase 1 human trials have also utilized this vector and have demonstrated no significant adverse events or dose-limiting toxicity at doses of up to 1011 viral particles.21,22
Equine infectious anemia virus (EIAV) is a nonprimate lentivirus that is nonpathogenic in humans.23 It has been shown to efficiently transduce RPE cells with reporter gene expression of up to 16 months after single subretinal delivery in mice.17,24
It should be noted that there is a theoretical concern regarding intravitreal injection of viral vectors that would express anti–vascular endothelial growth factor (VEGF) proteins. These agents could have access to the brain as anterograde transport from the retinal ganglion cells has been shown.25 In addition to the unknown effect of these viral vectors on the brain, if anti-VEGF proteins are transported into the central nervous system, they may cause long-term deleterious effects on learning and memory since VEGF may play a role in neurogenesis.26
VEGF BINDING MOLECULES
Vascular endothelial growth factor is among the main factors involved in pathologic neovascularization and is a major simulator of CNV formation in AMD, both in humans and animal models.27-29 The Food and Drug Administration–approved antineovascular treatments in clinical practice today are based on binding and inhibiting VEGF by a VEGF-neutralizing oligoclonal aptamer (pegaptanib sodium; Macugen, OSI/Pfizer)6 or a humanized monoclonal antibody fragment (ranibizumab; Lucentis, Genentech).7 The gene therapeutic approaches being developed have focused on transducing the retina or RPE cells to express a VEGF binder. AAV and adenovirus vectors encoding a soluble VEGF receptor, sFlt-1, have been demonstrated to inhibit the formation of new vessels in different models of neovascularization in rodents and nonhuman primates after subretinal, intravitreal, or periocular gene delivery.15,30-32 Pechan and colleagues constructed a vector encoding a high affinity soluble chimeric VEGF-binder delivered by AAV and demonstrated its efficacy in reduction of neovascularization in the oxygen induced retinopathy of prematurity mouse model.16 They also demonstrated stable protein expression with no histological evidence of toxicity for more than 1 year.
APPROACHES UTILIZING SMALL INTERFERING RNA (SIRNA)
Ribonucleic acid interference (RNAi) is a gene-silencing mechanism utilized by cells to downregulate the expression of genes corresponding to a double-stranded RNA or siRNA that has been introduced into the cell in vivo.33,34 This cellular machinery can be employed to silence inappropriate overexpression of disease-causing genes (Figure 1).34,35 In the case of neovascular AMD, investigators have focused on downregulating VEGF and its receptor.19,36,37 Adenovirus vector has been used as the delivery vehicle for the siRNA targeting VEGF. This model overcomes the main hindrance to the therapeutic potential of siRNA, its limited stability, and the need to specifically deliver it to the pathologic cells. Cashman and colleagues showed VEGF messenger RNA (mRNA) silencing of >90% in a human RPE cell line and over 80% reduction in the size of CNV in a murine model of neovascular AMD by adenovirus-mediated subretinal delivery of siRNA targeting VEGF.19 Interestingly, Kleinman and colleagues have recently shown that siRNAs 21-nucleotide or longer, as a class, suppress CNV in mice regardless of the sequence they are targeting via their interaction with a cell surface receptor called toll-like receptor 3.38
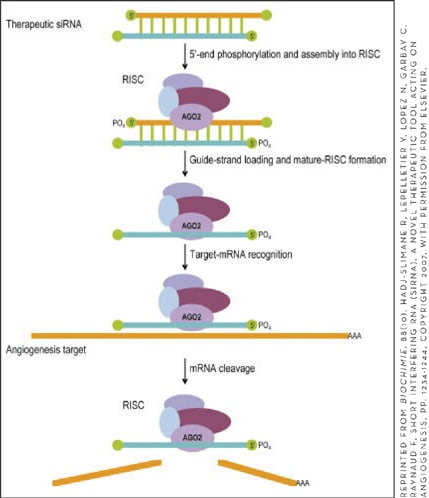
Figure 1. Schematic representation of the mechanism of action of siRNA. Delivery of siRNA in the transfected cells results in the assembly of a protein-RNA complex called the RNA-inducing silencing complex (RISC). After the unwinding of the siRNA in the complex, it binds to the complementary proangiogenic mRNA, causing its destruction.
There are 2 clinical trials being conducted for the treatment of wet AMD with siRNA. A phase 2 clinical trial is currently active for Sirna-027 (Allergan, Irvine, CA), which targets a VEGF receptor, VEGFR-1, and a phase 2 clinical trials for bevasiranib (OPKO Health, Miami) targeting the VEGF mRNA has been completed.
GENE THERAPY WITH ANGIOSTATIN AND ENDOSTATIN
Endostatin and angiostatin are endogenous antiangiogenic proteins that were first studied in tumor angiogenesis.39,40 Endostatin is a cleavage product of collagen 18 and angiostatin is an internal fragment of plasminogen. Subretinal injection of AAV vector expressing angiostatin has been shown to decrease the size of laser-induced CNV in rats 28% to 39% after 14 and 150 days, respectively.41 Endostatin-mediated reduction of CNV size was first demonstrated after intravenous administration of adenoviral vector, transducing liver cells.42 The authors demonstrated an inverse correlation between endostatin serum levels and CNV size. Subsequently, it has been shown that endogenous endostatin and endostatin gene expression after subretinal delivery of adenovirus, EIAV, AAV, or BIV vectors reduce VEGF-induced retinal vascular permeability, retinal neovascularization, and CNV lesion size in mice.18,24,43,44 Delivery of EIAV-angiostatin vector in the subretinal space has also been shown to decrease the vascular permeability and the size of laser-induced CNV in mice — however, to a lesser extent than EIAV-endostatin (40% vs 31%, respectively, reduction in CNV size).24
PIGMENT EPITHELIAL DERIVED FACTOR
Pigment epithelial derived factor (PEDF) is a neurotrophic protein secreted by human fetal RPE cells that has been shown to have neuroprotective and antineovascular properties.45-49 Gene therapy with subretinal and intravitreal delivery of adenovirus vector or AAV expressing PEDF has been shown to reduce the formation of CNV in various mice models.50,51 Intravitreous and subretinal injection of adenoviral vector expressing PEDF cause regression of already formed CNV in mice.52 This is the only pharmacologic therapy to date that has shown regression of already formed CNV in the rodent model.
The first published results from a phase 1 clinical trial for gene therapy in AMD utilized an adenoviral vector delivery of PEDF.22 This study showed that the treatment is well tolerated, with no dose-limiting toxicity, and it can stabilize or reduce the CNV lesion size (Figure 2). Twenty-five percent of patients had mild transient inflammation and 21% had transient elevation of intraocular pressure of up to 40-50 mm Hg, which was easily managed by topical medications.
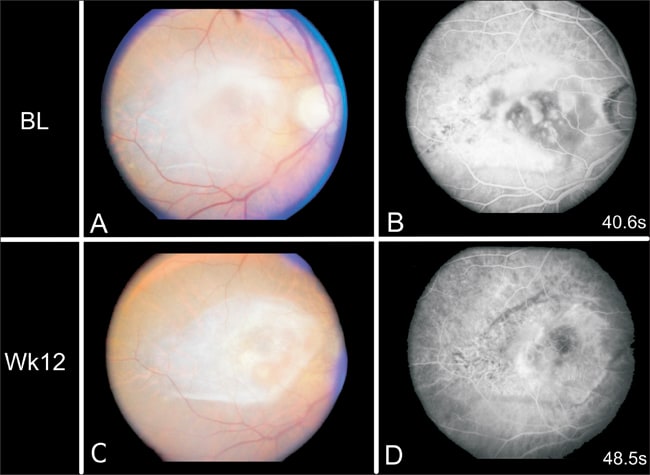
REPRINTED FROM HUMAN GENE THERAPY, 17 (2), CAMPOCHIARO PA, NGUYEN QD, SHAH SM, ET AL., ADENOVIRAL VECTOR DELIVERED PIGMENT EPITHELIUM-DERIVED FACTOR FOR NEOVASCULAR AGE-RELATED MACULAR DEGENERATION: RESULTS OF A PHASE I CLINICAL TRIAL, PP. 167-176, COPY RIGHT 2006, WITH PERMISSION FROM MARY ANN LIBERT, INC., PUBLISHERS.
Figure 2. Fluorescein angiogram (FA) of a patient at baseline (BL) and 3 months after an intravitreous injection of 107 adenovirus vector expressing PEDF. The appearance of the retina (A and C) shows no apparent toxicity and less subretinal fluid after treatment. The FA indicates less leakage after treatment.
CONCLUSION
The eye is an ideal organ for gene therapy using viral vectors as it allows for local delivery of a high number of viral particles with little systemic uptake. Pharmacological approaches to the treatment of neovascular AMD have significantly improved the visual prognosis; however, the delivery of these agents has proved to be a challenge. Gene therapy holds great potential for delivery of antineovascular pharmacotherapy. Various viral vectors have been used for the successful treatment of different rodent models of ocular neovascularization and have shown long-term expression of the intended pharmacological agent. Clinical trials conducted to date have not shown any dose-limiting toxicity.22 Currently there are 3 active clinical trials utilizing gene therapy for neovascular AMD: Two clinical trials are employing siRNA targeting the mRNA for VEGF or its receptor, and the other clinical trial involves the use of adenoviral vectors for the delivery of PEDF. The results to date are encouraging for the future of gene therapy in the treatment of neovascular AMD. As our understanding of the inflammatory components of AMD increases, local and systemic delivery of anti-inflammatory genes may play a role in the treatment of this disease. Apoptosis of the RPE and photoreceptor cells in non-neovascular and neovascular AMD is another prominent feature that could present a target for gene therapy in the future. RP
REFERENCES
- Pascolini D, Mariotti SP, Pokharel GP, et al. 2002 global update of available data on visual impairment: a compilation of population-based prevalence studies. Ophthalmic Epidemiol. 2004;11:67-115.
- Congdon N, O'Colmain B, Klaver CC, et al. Causes and prevalence of visual impairment among adults in the United States. Arch Ophthalmol. 2004;122:477-485.
- Ferris FL 3rd, Fine SL, Hyman L. Age-related macular degeneration and blindness due to neovascular maculopathy. Arch Ophthalmol. 1984;102:1640-1642.
- Seddon JM, Chen CA. The epidemiology of age-related macular degeneration. Int Ophthalmol Clin. 2004;44:17-39.
- D'Amato RJ, Adamis AP. Angiogenesis inhibition in age-related macular degeneration. Ophthalmology. 1995;102:1261-1262.
- Gragoudas ES, Adamis AP, Cunningham ET Jr, Feinsod M, Guyer DR. Pegaptanib for neovascular age-related macular degeneration. N Engl J Med. 2004;351:2805-2816.
- Rosenfeld PJ, Brown DM, Heier JS, et al. Ranibizumab for neovascular age-related macular degeneration. N Engl J Med. 2006;355:1419-1431.
- Gaudreault J, Fei D, Rusit J, Suboc P, Shiu V. Preclinical pharmacokinetics of Ranibizumab (rhuFabV2) after a single intravitreal administration. Invest Ophthalmol Vis Sci. 2005;46:726-733.
- Rex TS. Rescue of sight by gene therapy — closer than it may appear. Ophthalmic Genet. 2007;28:127-133.
- Anand V, Duffy B, Yang Z, Dejneka NS, Maguire AM, Bennett J. A deviant immune response to viral proteins and transgene product is generated on subretinal administration of adenovirus and adeno-associated virus. Mol Ther. 2002;5:125-132.
- Bennett J. Immune response following intraocular delivery of recombinant viral vectors. Gene Ther. 2003;10:977-982.
- Ali RR, Reichel MB, Thrasher AJ, et al. Gene transfer into the mouse retina mediated by an adeno-associated viral vector. Hum Mol Genet. 1996;5:591-594.
- Ali RR, Reichel MB, Hunt DM, Bhattacharya SS. Gene therapy for inherited retinal degeneration. Br J Ophthalmol. 1997;81:795-801.
- Ali RR, Reichel MB, De Alwis M, et al. Adeno-associated virus gene transfer to mouse retina. Hum Gene Ther. 1998;9:81-86.
- Lai CM, Shen WY, Brankov M, et al. Long-term evaluation of AAV-mediated sFlt-1 gene therapy for ocular neovascularization in mice and monkeys. Mol Ther. 2005;12:659-668.
- Pechan P, Rubin H, Lukason M, et al. Novel anti-VEGF chimeric molecules delivered by AAV vectors for inhibition of retinal neovascularization. Gene Ther. 2008 Jul 17. [Epub ahead of print]
- Balaggan KS, Binley K, Esapa M, et al. Stable and efficient intraocular gene transfer using pseudotyped EIAV lentiviral vectors. J Gene Med. 2006;:275-285.
- Takahashi K, Saishin Y, Silva RL, et al. Intraocular expression of endostatin reduces VEGF-induced retinal vascular permeability, neovascularization, and retinal detachment. FASEB J. 2003;17:896-898.
- Cashman SM, Bowman L, Christofferson J, Kumar-Singh R. Inhibition of choroidal neovascularization by adenovirus-mediated delivery of short hairpin RNAs targeting VEGF as a potential therapy for AMD. Invest Ophthalmol Vis Sci. 2006;47:3496-3504.
- Kreppel F, Luther TT, Semkova I, Schraermeyer U, Kochanek S. Long-term transgene expression in the RPE after gene transfer with a high-capacity adenoviral vector. Invest Ophthalmol Vis Sci. 2002;43:1965-1970.
- Chevez-Barrios P, Chintagumpala M, Mieler W, et al. Response of retinoblastoma with vitreous tumor seeding to adenovirus-mediated delivery of thymidine kinase followed by ganciclovir. J Clin Oncol. Nov 1 2005;23(31):7927-7935.
- Campochiaro PA, Nguyen QD, Shah SM, et al. Adenoviral vector-delivered pigment epithelium-derived factor for neovascular age-related macular degeneration: results of a phase I clinical trial. Hum Gene Ther. 2006;17:167-176.
- Sellon DC, Fuller FJ, McGuire TC. The immunopathogenesis of equine infectious anemia virus. Virus Res. 1994;32:111-138.
- Balaggan KS, Binley K, Esapa M, et al. EIAV vector-mediated delivery of endostatin or angiostatin inhibits angiogenesis and vascular hyperpermeability in experimental CNV. Gene Ther. 2006;13:1153-1165.
- Margolis TP, Marchand CM, Kistler HB Jr, LaVail LH. Uptake and anterograde axonal transport of wheat germ agglutinin from retina to optic tectum in the chick. J Cell Biol. 1981;89:152-156.
- Cao L, Jiao X, Zuzga DS, et al. VEGF links hippocampal activity with neurogenesis, learning and memory. Nat Genet. 2004;36:827-835.
- Kliffen M, Sharma HS, Mooy CM, Kerkvliet S, de Jong PT. Increased expression of angiogenic growth factors in age-related maculopathy. Br J Ophthalmol. 1997;81:154-162.
- Ryan SJ. Subretinal neovascularization. Natural history of an experimental model. Arch Ophthalmol. 1982;100:1804-1809.
- Yi X, Ogata N, Komada M, et al. Vascular endothelial growth factor expression in choroidal neovascularization in rats. Graefes Arch Clin Exp Ophthalmol. 1997;235:313-319.
- Bainbridge JW, Mistry A, De Alwis M, et al. Inhibition of retinal neovascularisation by gene transfer of soluble VEGF receptor sFlt-1. Gene Ther. 2002;9:320-326.
- Lai YK, Shen WY, Brankov M, Lai CM, Constable IJ, Rakoczy PE. Potential long-term inhibition of ocular neovascularisation by recombinant adeno-associated virus-mediated secretion gene therapy. Gene Ther. 2002;9:804-813.
- Gehlbach P, Demetriades AM, Yamamoto S, et al. Periocular gene transfer of sFlt-1 suppresses ocular neovascularization and vascular endothelial growth factor-induced breakdown of the blood-retinal barrier. Hum Gene Ther. 2003; 14:129-141.
- Rana TM. Illuminating the silence: understanding the structure and function of small RNAs. Nat Rev Mol Cell Biol. 2007;8:23-36.
- Hadj-Slimane R, Lepelletier Y, Lopez N, Garbay C, Raynaud F. Short interfering RNA (siRNA), a novel therapeutic tool acting on angiogenesis. Biochimie. 2007; 89:1234-1244.
- Cejka D, Losert D, Wacheck V. Short interfering RNA (siRNA): tool or therapeutic? Clin Sci (Lond). 2006;110:47-58.
- Reich SJ, Fosnot J, Kuroki A, et al. Small interfering RNA (siRNA) targeting VEGF effectively inhibits ocular neovascularization in a mouse model. Mol Vis. 2003; 9:210-216.
- Shen J, Samul R, Silva RL, et al. Suppression of ocular neovascularization with siRNA targeting VEGF receptor 1. Gene Ther. 2006;13:225-234.
- Kleinman ME, Yamada K, Takeda A, et al. Sequence- and target-independent angiogenesis suppression by siRNA via TLR3. Nature. 2008;452:591-597.
- O'Reilly MS, Holmgren L, Shing Y, et al. Angiostatin: a novel angiogenesis inhibitor that mediates the suppression of metastases by a Lewis lung carcinoma. Cell. 1994;79:315-328.
- O'Reilly MS, Boehm T, Shing Y, et al. Endostatin: an endogenous inhibitor of angiogenesis and tumor growth. Cell. 1997;88:277-285.
- Lai CC, Wu WC, Chen SL, et al. Suppression of choroidal neovascularization by adeno-associated virus vector expressing angiostatin. Invest Ophthalmol Vis Sci. 2001;42:2401-2407.
- Mori K, Ando A, Gehlbach P, et al. Inhibition of choroidal neovascularization by intravenous injection of adenoviral vectors expressing secretable endostatin. Am J Pathol. 2001;159:313-320.
- Auricchio A, Behling KC, Maguire AM, et al. Inhibition of retinal neovascularization by intraocular viral-mediated delivery of anti-angiogenic agents. Mol Ther. 2002;6:490-494.
- Marneros AG, She H, Zambarakji H, et al. Endogenous endostatin inhibits choroidal neovascularization. FASEB J. 2007;21:3809-3818.
- Tombran-Tink J, Johnson LV. Neuronal differentiation of retinoblastoma cells induced by medium conditioned by human RPE cells. Invest Ophthalmol Vis Sci. 1989;30:1700-1707.
- Ogata N, Wang L, Jo N, et al. Pigment epithelium derived factor as a neuroprotective agent against ischemic retinal injury. Curr Eye Res. 2001;22:245-252.
- Steele FR, Chader GJ, Johnson LV, Tombran-Tink J. Pigment epithelium-derived factor: neurotrophic activity and identification as a member of the serine protease inhibitor gene family. Proc Natl Acad Sci U S A. 1993;90:1526-1530.
- Cao W, Tombran-Tink J, Chen W, Mrazek D, Elias R, McGinnis JF. Pigment epithelium-derived factor protects cultured retinal neurons against hydrogen peroxide-induced cell death. J Neurosci Res. 1999;57:789-800.
- Dawson DW, Volpert OV, Gillis P, et al. Pigment epithelium-derived factor: a potent inhibitor of angiogenesis. Science. 1999;285:245-248.
- Mori K, Duh E, Gehlbach P, et al. Pigment epithelium-derived factor inhibits retinal and choroidal neovascularization. J Cell Physiol. 2001;188:253-263.
- Mori K, Gehlbach P, Yamamoto S, et al. AAV-mediated gene transfer of pigment epithelium-derived factor inhibits choroidal neovascularization. Invest Ophthalmol Vis Sci. 2002;43:1994-2000.
- Mori K, Gehlbach P, Ando A, McVey D, Wei L, Campochiaro PA. Regression of ocular neovascularization in response to increased expression of pigment epithelium-derived factor. Invest Ophthalmol Vis Sci. 2002;43:2428-2434.